Objective
Exercise systolic pulmonary arterial pressure (SPAP) predicts the occurrence of symptoms in patients with degenerative mitral regurgitation (MR). However, this measurement is not always available. The purpose of this study was to identify resting echocardiographic predictors of exercise SPAP and whether predicted exercise SPAP may predict symptom-free survival.
Methods
We prospectively enrolled 89 consecutive asymptomatic patients with moderate or greater degenerative MR in whom exercise SPAP was measurable. Left ventricular (LV) systolic and diastolic function were assessed by comprehensive transthoracic echocardiography. Tissue Doppler imaging was used to measure Ea- (early diastolic) and Sa- (systolic) wave velocities and time-to-peak (TP) Sa velocity. Multiple linear regression was built to identify resting predictors of exercise SPAP.
Results
Patients with exercise pulmonary hypertension (PHT) ( n = 45, 51%) were significantly older and more frequently male, and had shorter TP-Sa velocity and higher E/Ea ratio and LV end-diastolic volume than those without exercise PHT. Exercise SPAP was best correlated with resting LV end-diastolic volume ( r = 0.55), TP-Sa ( r = 0.72), and E/Ea ratio ( r = 0.52). Multiple linear regression analysis allowed us to build the following predictive equation with good precision ( r 2 = 0.80):
Predicted Exercise SPAP = 0.13 × Age + 0.05 × LVED Vol + 0.7 × E/Ea ratio − TP . Sa 10 + 51 mm Hg
By using this formula, predicted exercise SPAP was well correlated with observed exercise SPAP ( r = 0.89). Predicted exercise PHT was associated with significant reduced symptom-free survival in both univariate ( P = .04) and multivariate ( P = .045) analyses.
Conclusion
In asymptomatic patients with degenerative MR, exercise PHT can be accurately predicted using resting comprehensive echocardiography.
Chronic degenerative mitral regurgitation (MR) is a frequent and serious valvular heart disease. During a long asymptomatic phase, severe MR may contribute to the elevation of systolic pulmonary arterial pressure (SPAP) and lead to pulmonary hypertension (PHT), even in the absence of apparent left ventricular (LV) dysfunction. Although PHT is relatively infrequent in asymptomatic patients, one third of such patients requiring mitral valve surgery may have an SPAP ≥ 50 mm Hg. The management of patients with asymptomatic severe MR with preserved LV systolic function remains controversial, opposing early elective surgery to “watchful waiting” strategy. Current American College of Cardiology (ACC)/American Heart Association (AHA) guidelines recommend mitral valve repair for patients with either PHT or exercise PHT (SPAP > 60 mm Hg), even in the absence of LV dysfunction or dilatation or symptoms (Class IIa). A recent study reported that exercise SPAP is more accurate than resting SPAP to predict the occurrence of symptoms in patients with asymptomatic chronic degenerative MR. Moreover, exercise PHT is associated with a 3.4-fold increase in risk of developing symptoms at 3 years, whereas resting PHT was not found as an independent predictor of low symptom-free survival. Consequently, the use of exercise stress echocardiography and the measurement of exercise SPAP in asymptomatic patients with chronic MR seem to be useful to unmask patients at high risk to rapidly and more frequently develop symptoms, and thus to identify those in whom early elective surgery could be recommended. Nevertheless, exercise echocardiography is not always available, and exercise SPAP is impossible to obtain in the absence of tricuspid regurgitation and difficult to acquire and to accurately quantify in the presence of poor acoustic window, severe tricuspid regurgitation, or inability to perform exercise.
The objective of this study was to identify potential baseline or resting variables that may predict the occurrence of exercise PHT in asymptomatic patients with degenerative chronic MR. We also aimed to assess whether predicted exercise PHT may be useful to identify patients at high risk to develop symptoms.
Materials and Methods
We prospectively enrolled 110 consecutive asymptomatic patients with moderate or greater degenerative MR (effective regurgitant orifice [ERO] >20 mm 2 or regurgitant volume [RV] >30 mL) referred for exercise echocardiography. Patients with LV systolic dysfunction, according to current European Society of Cardiology guidelines (LV ejection fraction <60% or LV end-systolic diameter >45 mm), or atrial fibrillation were excluded. As required by the purpose and the design of the study, patients without measurable resting or exercise SPAP were also excluded from the final analysis ( n = 21, 19%). Demographic and clinical data were collected at the time of the echocardiographic examination.
Exercise Echocardiography
After a comprehensive resting Doppler echocardiogram, a symptom-limited graded bicycle exercise test was performed in the semi-supine position on a dedicated tilting exercise table, as previously described. Briefly, patients started with an initial workload of 25 W maintained for 2 minutes; the workload was increased every 2 minutes by 25 W. Blood pressure and a 12-lead electrocardiogram were recorded every 2 minutes. Two-dimensional and Doppler echocardiographic imaging were available throughout the test. Doppler hemodynamic measurements were obtained at peak exercise (i.e., within the minute before and the minute after the end of exercise).
Echocardiographic Study
Transthoracic echocardiographic examinations were performed with a Vivid 7 or 9 imaging device (GE Healthcare, Little Chalfont, UK). All echocardiographic and Doppler data were obtained at rest and at peak exercise in digital format and then stored on a workstation for offline analysis (EchoPAC, GE Vingmed Ultrasound AS, Horten, Norway).
LV dimensions were measured from parasternal long-axis views by two-dimensional guided M-mode using the leading edge methodology at end-diastole and end-systole. The biapical Simpson disk method was used to obtain LV end-diastolic and end-systolic volumes and left atrial maximal volume. The LV stroke volume was calculated by multiplying the LV outflow tract area by the LV outflow tract velocity–time integral measured by pulsed-wave Doppler. Mitral E- and A-wave velocities were measured with pulsed-wave Doppler. To estimate the LV filling pressure, pulsed-wave tissue Doppler imaging was used to measure early diastolic (Ea) mitral annulus velocities, and the E/Ea ratio was calculated. The systolic (Sa) and time-to-peak (TP) Sa and Ea velocities were quantified using color tissue Doppler imaging. Both Ea and Sa velocities were obtained from septal and lateral sites and then averaged ( Figure 1 ). Sa and TP-Sa are markers of LV longitudinal myocardial function.

MR severity was quantified using both the Doppler volumetric method and proximal isovelocity surface area (PISA) method. The results of the two methods were averaged to obtain the ERO area and the RV. Continuous-wave Doppler was used to measure the maximal velocity and the time-velocity integral of the MR jet. The Doppler volumetric method allows the calculation of ERO by dividing the RV, which is obtained by the subtraction of LV outflow tract stroke volume from mitral antegrade stroke volume, by the time-velocity integral of the MR. As recommended, the midsystolic PISA radius was measured from at least three frames with optimal flow convergence. The most appropriate negative aliasing velocity to obtain hemispheric PISA was selected offline on the workstation.
SPAP was obtained from the regurgitant jet of tricuspid regurgitation using systolic transtricuspid pressure gradient calculated by the modified Bernoulli equation (∆P = 4v 2 , where v is maximal tricuspid regurgitant jet velocity in m/s) and the addition of 10 mm Hg for right atrial pressure as previously described and validated. According to ACC/AHA guidelines and to our recent studies, resting PHT and exercise PHT were defined as SPAP >50 and >60 mm Hg, respectively. Right atrial pressure was assumed to be constant from rest to exercise.
Symptom-Free Survival
Patient follow-up was performed according to current guidelines and as previously described. Briefly, patients were classified as symptomatic when shortness of breath, angina, dizziness, or syncope with exertion was identified during follow-up. Patients were reevaluated every 12 months, including physical examination and echocardiography. Intervals of evaluations were shortened to 6 or 3 months in patients with changes compared with previous measurements or if echocardiographic measurements were close to guideline cutoff values used for surgical indication.
Statistical Analysis
Patients were divided into two groups according to the presence or absence of exercise PHT. The results are expressed as mean ± standard deviation or percentages unless otherwise specified. Statistical differences between groups were assessed using Student t test, chi-square test, or Fisher exact test, as appropriate.
Correlations between echocardiographic data were evaluated using linear regression. To identify the independent predictors of exercise SPAP and build a predictive equation, we used multiple linear regression. Variables univariately associated with exercise SPAP were incorporated into the model. To avoid colinearity, which could interfere with the multivariate model, special care was taken in the choice of variables included. The resulting equation was used to create the “predicted exercise SPAP.” Receiver operative characteristic analyses were performed to compare the accuracy of resting variables to predict the occurrence of exercise PHT. The symptom-free survival was assessed using Kaplan-Meier curves, and statistical differences between patients with or without exercise PHT were obtained using a log-rank test. The effect of PHT on symptom-free survival was assessed with Cox proportional-hazards models in univariate and multivariable analyses. All statistical analyses were performed with STATISTICA version 6 (StatSoft Inc., Tulsa, OK).
Results
Patient Characteristics
Among the 110 consecutive patients examined, 89 (61 ± 14 years, 55% were male) had measurable exercise SPAP (81%). The SPAP significantly increased during exercise from 38.4 ± 4 to 62.5 ± 17 mm Hg ( P < .0001, Figure 2 ). Whereas 11 patients (12%) had resting PHT, 45 (51%) developed PHT during exercise ( Table 1 ). Patients with exercise PHT were significantly older ( P = .012) and more frequently male ( P = .043). No other demographic or clinical data were useful to identify patients developing exercise PHT. Mitral valve prolapse more frequently involved both leaflets in patients without exercise PHT ( P = .04). There was a trend for higher prevalence of severe MR in the group of patients with exercise PHT ( P = .09).

Variables | All patients ( n = 89) | No Ex-PHT ( n = 44, 49%) | Ex-PHT ( n = 45, 51%) | P value |
---|---|---|---|---|
Age, y | 61 ± 14 | 58 ± 14 | 65 ± 12 | .012 |
Male, n (%) | 52 (55) | 20 (45) | 31 (69) | .043 |
Heart rate, bpm | 73 ± 12 | 73 ± 2 | 73 ± 2 | .88 |
Systolic BP, mm Hg | 137 ± 18 | 131 ± 16 | 143 ± 19 | .012 |
Diastolic BP, mmHG | 76 ± 11 | 74 ± 9 | 78 ± 12 | .16 |
Maximal load, W | 106 ± 31 | 106 ± 32 | 106 ± 31 | .95 |
Hypertension, n (%) | 40 (45) | 18 (41) | 22 (49) | .59 |
Overweight, n (%) | 11 (12) | 6 (14) | 5 (11) | .97 |
Hypercholesterolemia, n (%) | 21 (24) | 10 (22) | 11 (24) | .95 |
Diabetes, n (%) | 6 (7) | 2 (4) | 4 (9) | .68 |
Smoker, n (%) | 26 (29) | 13 (30) | 13 (29) | .87 |
Mitral prolapse localization | ||||
Anterior, n (%) | 9 (10) | 2 (5) | 7 (15) | .16 |
Posterior, n (%) | 42 (47) | 18 (41) | 24 (53) | .34 |
Both, n (%) | 38 (43) | 24 (54) | 14 (31) | .04 |
Flail leaflet, n (%) | 11 (12) | 3 (7) | 8 (18) | .21 |
Echocardiographic Data
Patients with exercise PHT ( Table 2 ) had shorter TP-Sa ( P < . 0001) and higher Ea wave ( P = .01), E/Ea ratio ( P = .0009), LV end-diastolic volume ( P = .014), and SPAP ( P < . 0001). There was no other significant difference between the two groups with regard to resting LV end-systolic volume, LV systolic and diastolic function, and MR severity as assessed with ERO and RV. During exercise, patients with exercise PHT had significant higher E-wave velocity, E/Ea ratio, and MR severity ( Table 2 ).
Variables | All patients ( n = 89) | No Ex-PHT ( n = 44, 49%) | Ex-PHT ( n = 45, 51%) | P value |
---|---|---|---|---|
Resting LV volumes | ||||
LV end-systolic volume, mL | 35 ± 12 | 34 ± 12 | 36 ± 12 | .44 |
LV end-diastolic volume, mL | 116 ± 36 | 107 ± 34 | 126 ± 37 | .014 |
LV stroke volume, mL | 81 ± 23 | 83 ± 24 | 77 ± 25 | .40 |
Resting LV systolic function | ||||
Cardiac output, L/min | 5.8 ± 1.9 | 6.0 ± 2.0 | 6.6 ± 1.9 | .53 |
LV ejection fraction, % | 69 ± 6 | 69 ± 6 | 69 ± 6 | .79 |
Mean Sa velocity, cm/s | 6.4 ± 1.5 | 6.7 ± 1.3 | 6.0 ± 1.7 | .12 |
Mean T-P Sa, ms | 143 ± 44 | 171 ± 32 | 115 ± 39 | <.0001 |
Resting LV diastolic function | ||||
Mean Ea, cm/s | 7.3 ± 1.9 | 7.9 ± 1.8 | 6.6 ± 1.8 | .01 |
Mean T-P Ea, ms | 468 ± 46 | 479 ± 43 | 461 ± 48 | .15 |
Peak E velocity, cm/s | 99 ± 33 | 94 ± 25 | 109 ± 38 | .10 |
E/A ratio | 1.5 ± 0.6 | 1.4 ± 0.6 | 1.5 ± 0.7 | .54 |
E/Ea ratio | 14.2 ± 5.6 | 12.1 ± 3.5 | 17.1 ± 6.3 | .0009 |
Resting MR | ||||
RV, mL | 71 ± 29 | 71 ± 34 | 71 ± 20 | .95 |
ERO area, mm 2 | 44 ± 20 | 43 ± 24 | 44 ± 16 | .88 |
Severe MR, n (%) | 57 (64) | 28 (63) | 29 (64) | .89 |
Resting left atrial volume, mL | 70 ± 24 | 69 ± 27 | 72 ± 21 | .41 |
Resting systolic PAP, mm Hg | 38.4 ± 10 | 33.5 ± 7 | 43.9 ± 11 | <.0001 |
Exercise LV volumes | ||||
LV end-systolic volume, mL | 30 ± 14 | 30 ± 16 | 30 ± 13 | .98 |
LV end-diastolic volume, mL | 105 ± 39 | 103 ± 40 | 109 ± 38 | .57 |
LV stroke volume, mL | 86 ± 25 | 89 ± 25 | 83 ± 25 | .38 |
Exercise LV systolic function | ||||
Cardiac output, L/min | 10.8 ± 3.1 | 11 ± 2.9 | 10.4 ± 3.2 | .43 |
LV ejection fraction, % | 72 ± 9 | 71 ± 9.7 | 72 ± 9.4 | .55 |
Mean Sa velocity, cm/s | 9 ± 2.5 | 9.4 ± 2.4 | 8.3 ± 2.4 | .10 |
Mean T-P Sa, ms | 101 ± 33 | 105 ± 37 | 99 ± 29 | .49 |
Exercise LV diastolic function | ||||
Mean Ea, cm/s | 9.6 ± 2.4 | 9.8 ± 5.6 | 8.9 ± 2.1 | .18 |
Mean T-P Ea, ms | 361 ± 60 | 369 ± 52 | 360 ± 65 | .58 |
Peak E velocity, cm/s | 140 ± 42 | 130 ± 41 | 150 ± 41 | .01 |
E/A ratio | 1.5 ± 0.4 | 1.4 ± 0.4 | 1.5 ± 0.3 | .42 |
E/Ea ratio | 15.2 ± 5.2 | 13.4 ± 4.9 | 17 ± 4.7 | .0023 |
Exercise MR | ||||
RV, mL | 71 ± 31 | 63 ± 32 | 81 ± 29 | .007 |
ERO area, mm 2 | 47 ± 24 | 40 ± 22 | 54 ± 24 | .005 |
Severe MR, n (%) | 58 (65) | 20 (45) | 38 (84) | <.0001 |
Exercise left atrial volume, mL | 78 ± 29 | 83 ± 36 | 86 ± 23 | .55 |
Exercise systolic PAP, mm Hg | 62.5 ± 17 | 48 ± 6 | 76 ± 12 | <.0001 |
Prediction of Exercise MR Severity
Exercise ERO was significantly correlated with resting ERO ( r = 0.80, P < . 0001), Sa wave velocity ( r = 0.49, P = .0001), TP-Sa ( r = −0.51, P = .0001), E/Ea ratio ( r = 0.42, P = .001), and LV end-diastolic volume ( r = 0.53, P < . 0001). Of note, there was no significant correlation between age and exercise ERO. On multiple linear regression, only resting ERO (β = 0.87 ± 0.13, P < . 0001) and Sa wave velocity (β = 3.1 ± 1.2, P = .018) were identified as independent predictors of exercise ERO. In the same model, when resting ERO was excluded, resting Sa velocity (β = 6.9 ± 1.5, P < . 0001), TP-Sa (β = −0.12 ± 0.05, P = .026), and LV end-diastolic volume (β = 0.12 ± 0.05, P = .025) were independent predictors of exercise ERO. We found similar result with exercise RV.
Relationship Between Resting Data and Exercise SPAP
There was a good correlation between exercise SPAP and age ( r = 0.29, P = .005). Correlations between resting echocardiographic data and exercise SPAP are reported in Table 3 . In the whole cohort, the best correlations were found with LV end-diastolic volume ( r = 0.55, P < . 0001), E/Ea ratio ( r = 0.35, P = .008), TP-Sa ( r = −0.72, P < . 0001), and, as expected, resting SPAP ( r = 0.58, P < . 001). Similar results were found in both groups of patients with or without exercise PHT ( Table 3 ).
Variables | Whole cohort | No Ex-PHT ( n = 44, 49%) | Ex-PHT ( n = 45, 51%) | |||
---|---|---|---|---|---|---|
r | P value | r | P value | r | P value | |
LV volumes | ||||||
LV end-systolic volume | 0.19 | .14 | 0.19 | .29 | 0.17 | .36 |
LV end-diastolic volume | 0.55 | <.0001 | 0.42 | .003 | 0.37 | .04 |
LV stroke volume | 0.04 | .79 | −0.03 | .86 | −0.08 | .67 |
LV systolic function | ||||||
Cardiac output | 0.04 | .79 | 0.09 | .62 | 0.07 | .96 |
LV ejection fraction | 0.04 | .74 | 0.08 | .65 | 0.25 | .17 |
Mean Sa velocity | 0.03 | .85 | 0.17 | .35 | 0.28 | .13 |
Mean T-P Sa | −0.72 | <.0001 | −0.43 | .002 | −0.54 | <.0001 |
LV diastolic function | ||||||
Mean Ea | 0.05 | .71 | 0.02 | .89 | 0.08 | .67 |
Mean T-P Ea | −0.19 | .14 | 0.02 | .93 | −0.03 | .86 |
Peak E velocity | 0.30 | .02 | 0.06 | .74 | 0.08 | .65 |
E/A ratio | 0.29 | .03 | 0.18 | .36 | 0.31 | .12 |
E/Ea ratio | 0.52 | .008 | 0.28 | .05 | 0.30 | .04 |
MR | ||||||
RV | 0.08 | .48 | 0.29 | .052 | 0.19 | .23 |
ERO area | 0.16 | .14 | 0.14 | .62 | 0.36 | .02 |
Left atrial volume | 0.08 | .48 | 0.07 | .66 | 0.19 | .23 |
Systolic PAP | 0.58 | <.0001 | 0.33 | .023 | 0.38 | .008 |
Patients with resting PHT had significant higher exercise SPAP compared with those without resting PHT (81.4 ± 14.7 mm Hg vs. 59.8 ± 15.9 mm Hg, P < . 0001).
Prediction of Exercise PHT
In multivariate analysis, age, E/Ea, TP-Sa, LV end-diastolic volume, and resting SPAP were independent predictors ( r 2 = 0.83) of exercise SPAP ( Table 4 ). Except age (area under the curve [AUC] = 0.63), these parameters were associated with good accuracy ( Figure 1 ) for predicting the occurrence of exercise PHT (E/Ea: AUC = 0.90; TP-Sa: AUC = 0.82; LV end-diastolic volume: AUC = 0.83; resting SPAP: AUC = 0.80).
Variables | β | SE | P value |
---|---|---|---|
Age | 0.1 | 0.06 | .05 |
E/Ea ratio | 0.6 | 0.1 | .0006 |
TP–Sa | −0.1 | 0.02 | .0007 |
LV end-diastolic volume | 0.07 | 0.002 | .0013 |
Resting systolic PAP | 0.3 | 0.05 | .0012 |
The following formula (1) was built using the multivariable results to predict exercise SPAP:
Predicted Exercise SPAP = 0.3 × Resting SPAP + Age 10 + 0.07 × LVED Vol + 0.6 × E/Ea ratio − TP . Sa 10 + 36 mm Hg ,
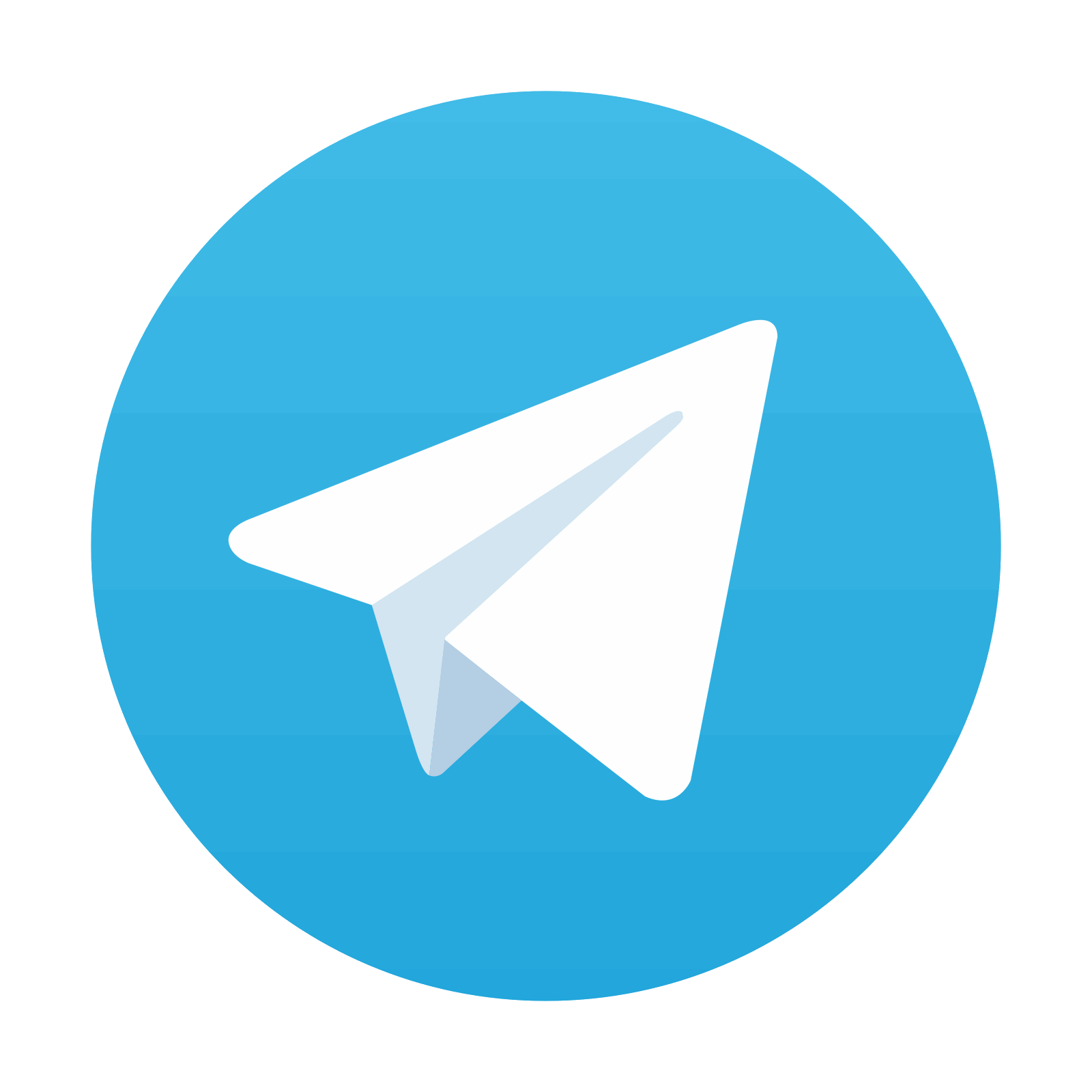
Stay updated, free articles. Join our Telegram channel

Full access? Get Clinical Tree
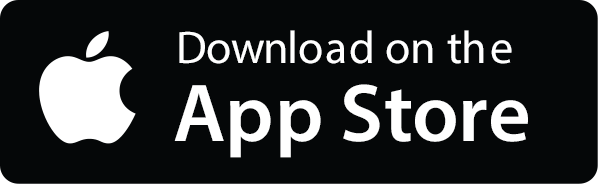
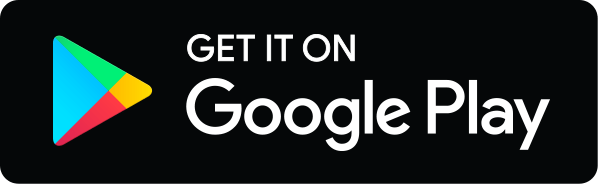