Score
Clinical term
+4
Combative
+3
Very agitated
+2
Agitated
+1
Restless
0
Alert and calm
−1
Drowsy
−2
Light sedation
−3
Moderate sedation
−4
Deep sedation
−5
Unarousable
(Sessler et al. 2001, 2002, 2008, 2013; Ely et al. 2003; Turkmen et al. 2006; Khan et al. 2012; Patel and Kress 2012; Barr et al. 2013; Benitez-Rosario et al. 2013)
5.2.2 Sedation-Agitation Scale (SAS)
SAS is much easier than RASS; in other words, it is a 7-step scale without negative scores. The “calm and cooperative patient” in SAS gets a score of 4. However, SAS is graded as in Table 5.2. SAS is demonstrated as a valid and reliable scale for assessment of sedation status in postoperative period of adult patients in intensive care unit.
Table 5.2
A summary of the Sedation-Agitation Scale (SAS)
Score | Clinical term |
---|---|
7 | Dangerous agitation |
6 | Very agitated |
5 | Agitated |
4 | Calm and cooperative |
3 | Sedated |
2 | Very sedated |
1 | Unarousable |
5.3 Electroencephalography (EEG) Including Multichannel or Uni-channel EEG
Electroencephalography (EEG) was first introduced by German psychiatrist Hans Berger in 1926 while he presented a number of very exact recordings of the brain electrical activities which are still valid.
Speaking physiologically, the oxygen supply to the CNS is divided as two main categories; the majority (i.e., about 60 %) is provided for specialized neuronal activity including axonal and synaptic transmission; this section is suppressed after administration of anesthetic. Another 40 % of the energy supply is assigned to maintenance of cellular integrity; usually, anesthetics do not affect the latter while hypothermia could depress it. Usually CNS neurons adjust themselves in the ischemic state to be able to continue their basal function, i.e., the portion of their activity related to the 40 % energy requirements for basal homeostasis.
When monitoring the CNS using EEG, we should always consider EEG as a good CNS monitoring but not a perfect one, since:
The EEG electrodes record the electrical activity of the neurons just under the scalp (i.e., the cortical neurons); however, these electrodes would not record the electrical activity of thalamus or the subcortical nuclei as well as the cortical neurons; this is why EEG electrodes, even if located directly on the cortical tissue, record mainly the neurologic activity of the cortex and do not assure ischemia prevention in the subcortical brain nuclei.
Conventional EEG has not been widely used for cardiac operations due to its technical limitations.
Though the EEG presentations in ischemic insult are often similar, it is not always the same; at times, the ischemic neurons are those which have inhibitory function and their ischemic presentation would be as overactivity of the CNS.
EEG could demonstrate the ischemic events; however, its role as a CNS monitoring is not to demonstrate the site of ischemia, the etiologic mechanism responsible for ischemia or the anatomic location of injury.
EEG is a “biorhythm” affected a number of factors like age, environment, and circadian variations, as cited by Constant et al.
In 2009, the American Society of Neurophysiological Monitoring (ASNM) has published the “Guidelines for intraoperative neuromonitoring using raw and quantitative electroencephalography” prepared by Isley et al.; the recommendations presented in this guideline are direct and decisive regarding perioperative EEG monitoring.
In patients undergoing cardiac surgery, especially those with higher risk of CNS injury (including the older patients), perioperative EEG could help in detection, monitoring, and prevention of CNS-related problems. The following are among the most common uses of perioperative EEG in cardiac surgery patients according to Isley et al. and Gugino et al.:
1.
Detection and documentation of any preoperative baseline CNS disorder; also, documentation of any possible new event in the perioperative period (including postoperative period).
2.
Baseline EEG (i.e., before anesthesia induction) should be documented as the baseline data especially in those at increased risk of CNS injury for comparison with later findings.
3.
Intraoperative EEG monitoring and postoperative EEG records both discover new findings and differentiate them from baseline abnormalities while detecting new findings; intraoperative or postoperative new findings should be assessed carefully and cautiously to find potentially treatable new findings.
4.
EEG could help us tailor the dosage of anesthetics and sedatives during intraoperative and postoperative period.
5.
Monitoring the efficacy of anticonvulsant therapies in patients having seizure in postoperative period.
6.
Acute hemodilution due to rapid postoperative bleeding (necessitating volume replacement with large volumes of crystalloids) or hemodilution during cardiopulmonary bypass is an example of state in which cardiac surgery patients are exposed to acute hemodilution; this hematocrit drop needs CNS monitoring to detect any possible regional or global ischemic insult associated with microcirculatory collapse, especially in patients at risk of CNS injury; these patients benefit from EEG monitoring.
7.
During the early period after rewarming from CPB, the brain neurons return to the normal temperature, while the CNS perfusing arteries remain partially in some degrees of spam; it means that during early rewarming period, brain oxygen demands would be more than oxygen supply which causes some degrees of brain ischemia; also, this phenomenon could be extended to the postoperative period in which EEG monitoring could help detect these ischemic periods.
8.
When hypothermia is administered during the perioperative period as a method of cerebral protection, EEG could help us monitor the efficacy of hypothermia presented as EEG silence.
9.
Hyperventilation in postoperative period causes hypocapnia which would in turn lead to cerebral arterial vasoconstriction and reduced CNS perfusion; monitoring the potential ischemic effects of this phenomenon could be done using EEG whenever the patient needs more vigorous care.
5.3.1 How EEG Works
EEG is the indicator for CNS activity, mainly the cortex and especially the postsynaptic activity of the cortical neurons; usually, the total brain electrical activity is recorded using the standard 10/20 electrodes placed on different parts of the scalp. EEG electrodes record the electrical activities of those cortical neurons located in the brain cortex just under the scalp; these neurons are “pyramids” with their direction being perpendicular to the scalp; in fact, the cortical neurons are the long ones with elongated axons located vertical to the scalp.
EEG is the summative activity of cortical neurons’ functions (both excitatory and inhibitory functions); in other words, postsynaptic electrical currents of millions of cortical neurons (called pyramidal cortical cells or Betz cells) are summated and accumulated together to create EEG waves; however, the axonal activity of the cortex does not contribute an important role in EEG wave production.
EEG waves have the following main electrical characteristics:
1.
Amplitude: amplitude of waves is decreased with increasing age as a result of aging; the electrical amplitude of EEG waves is in the range of 10–100 μV, about 100 times less than electrical amplitude of electrocardiography.
2.
Frequency: the number of times in each second that a wave recurs, presented as waves per second, i.e., Hz; detailed description could be found in Table 5.3.
Table 5.3
A summary of EEG waves and their brief characteristics
Wave category | Symbol | Frequency | Voltage | Related activity | Clinical equivalent | |
---|---|---|---|---|---|---|
1. | Beta waves | β | 12.6–25 Hz, in some references 13–30 Hz | In adults 10–20 μV | Cortico-cortical network | Fully awake patient with open eyes |
2. | Alpha waves | α | 7.6–12.5 Hz, in some references 8–13 Hz | 30–50 μV; in adults: 10–20 μV | Corticothalamic network | Awake patient but eyes are closed equals drowsy state |
3. | Theta waves | θ | 3.6–7.5 Hz, in some references 4–7 Hz | 50–100 μV; in adults: 10–20 μV | Corticothalamic activity and limbic activity | Stage 2 of sleep (light sleep) |
4. | Delta waves | δ | 1.5–3.5 Hz, in some references 0.5–3 Hz | 100–200 μV | Corticothalamic dissociation | Deep sleep and coma |
5. | Gamma waves | γ | 25.1–55 Hz, in some references 30–50 Hz | Corticothalamic perception | Involved in the process of perception |
3.
Time: the horizontal axis of EEG is always time.
4.
Symmetry is an index of normality; even when the patients are anesthetized, the two hemispheres present symmetric EEG changes while pathologic states disturb symmetry.
The EEG electrodes record the “voltage difference” between each of the two different electrodes. The difference in electrode voltages is demonstrated against a time scale as the following pattern:
If the difference between the 1st and the 2nd electrodes is negative, it would appear as above the scale deflection (i.e., up deflection) on EEG.
If this difference between the 1st and the 2nd electrodes is positive, it would appear as below the scale deflection (i.e., down deflection) on EEG.
The EEG electrodes could be metal discs called “cup” electrodes made of “tin, silver, or gold.” Also, needle electrodes are available used when sterile application of EEG electrodes is mandatory like neurosurgical operations, though their use should be limited to “obligatory” conditions. The third type of electrodes is called “silver-silver chloride” electrodes. Whatever type of electrode is used, the electrode montage should be from a constant type, with appropriate quality to prevent artifacts and, also, enough gel to guarantee low amount of impedance. We also should be sure that electrodes be well attached.
Another important point is that EEG connection wires and cables should not be in contact or in the vicinity of other cables to improve EEG signal quality. Also, it is recommended to use EEG leads as shielded leads.
Usually, 2–8 channels are used for intraoperative or postoperative CNS recording; even at times, three electrodes are used on the frontal area, two of them for recording the neuronal electrical activity as “differential amplifier with voltage difference” and the 3rd electrode used as the “mandatory reference signal” electrode. However, some references stress on using not less than eight channels.
The function of EEG is divided into “few seconds” interval; this is why EEG waves are taken in defined time periods called epochs. These time periods are usually 2–4 s. The electrical activity waves are taken during these time epochs and are then analyzed by the device microprocessor to be demonstrated as final EEG waves on a time-based scale.
EEG electrode attachment order on the scalp is named montage of electrodes. In the standard system of the electrodes, there are four main anatomic landmarks used for electrode attachment over the scalp in different directions:
One nasion (anterior)
One inion (posterior)
Two preauricular points
The location of electrodes is named according to the above anatomic locations and also the standard coding system which uses anatomic, alphabetic, and numeric items (i.e., anatomic and alphanumeric recording); this standard system helps us differentiate the location of any abnormal wave and also to compare similar locations on two hemispheres (Fig. 5.1):
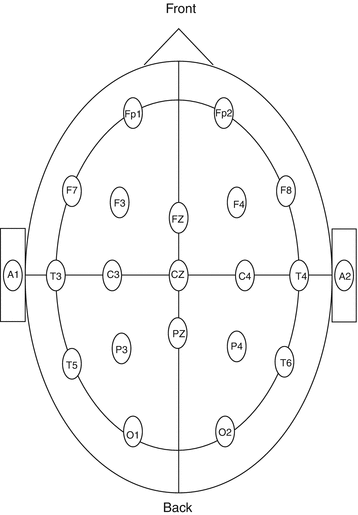
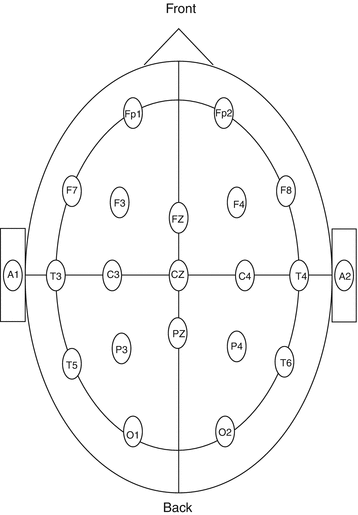
Fig. 5.1
The standard 10/20 electrode system
F for frontal electrodes.
T for temporal electrodes.
O for occipital electrodes.
P for parietal electrodes.
C for central electrodes.
A for auricular electrodes.
M for mastoid electrodes.
Even numbers as subscripts demonstrate right hemisphere.
Odd numbers as subscripts demonstrate left hemisphere.
Z as subscript demonstrates midline electrodes (z: zero).
5.3.2 Frequency of EEG Waves and the Changes During Wakefulness, Sleep, and Anesthesia
EEG rhythms during wakefulness, sleep, and anesthesia are the result of balance between cortex and thalamus, depending on the level of consciousness or the stage of anesthesia. However, EEG waves are classified as the five frequency bands; their frequencies are a bit different between different authors; however, according to Gugino et al. the frequencies are classified as demonstrated in Table 5.3 and also, described in the following paragraphs.
5.3.3 Normal and Abnormal EEG
Normal and Abnormal EEG in normal EEG, symmetry between hemispheres is clearly evident; also, the EEG waves are according to the category mentioned above without any spike waves; the spike waves suggest epileptic activity.
However, abnormal EEG may be mainly due to ischemia, infarct, epilepsy, or tumor. For example, these are a number of well-known findings in pathologic EEG:
Asymmetric patterns between hemispheres (could be sign of ischemia or arterial occlusion).
Spikes.
Decreased frequency.
Decreased voltage.
Also, if normal waves are seen in abnormal states, it would be suggestive of a pathologic state (like abnormal appearance of delta waves which could be suggestive for a brain lesion).
5.3.4 EEG in Cardiac Surgery Patients
The role of EEG in cardiac surgery ICU could be among the following:
As a continuous monitoring device and a real-time neurologic assessment tool and at the same time, to monitor CNS like the other major organ systems which should be monitored in the perioperative period; this is especially important when the hemodynamic status is not stable and there is the risk for impaired CNS perfusion.
As a diagnostic tool, for example, in detection of epilepsy, ischemic or hemorrhagic CNS events, coma, brain death, and drug toxicities or to rule out decreased level of consciousness due to residual anesthetic effects from other etiologies of decreased consciousness.
As a therapy tailoring method, dosage titration tool, or drug adjustment scale, for example, as a tool in clinical approval of the anticonvulsant drugs’ efficacy or making any needed change in their dose or in barbiturate-induced or hypothermia-induced coma, for objective approval of their efficacy and confirmation of barbiturate or hypothermia-induced cortical silence.
Also, as discussed in Chap. 10 of this book, cardiac surgery patients tolerate a considerable effect on their CNS due to the process of the disease and the therapeutic approaches. For example, in cardiac surgery patients, postoperative cerebral edema and decreased CNS oxygen delivery due to edema is often seen in the early postoperative days. This finding is seen in nearly all cardiac procedures, though the frequency is not always the same, with the following characteristics:
EEG findings in cardiac surgery patients are similar to findings in those affected with organic brain syndrome.
The postoperative changes are more frequent in the left hemisphere.
These EEG findings are more common after more invasive, complex procedures; so, the prevalence is higher in valve replacement compared with valve repair or CABG.
More common in on pump CABG’s compared with off-pump CABG’s, however, seen even in off-pump patients.
During periods of postoperative CNS ischemia, EEG waves change to ischemic pattern whenever cerebral blood flow (CBF) is <22 mL/100 g/min.
During early stages of ischemia, frequency of waves decreases while the voltage is preserved.
If more severe ischemia occurs, both wave frequency and voltage are depressed.
These changes are especially seen as significant decrease “in the beta and the alpha 2 bands.”
EEG amplitude drop >30 % or duration of EEG changes >30 s have been cited as important indicators of ischemia by Florence et al.
The effects of anesthetics, analgesic agents, temperature variations, and blood pressure fluctuations in postoperative period should be considered.
(Zeitlhofer et al. 1988; Chabot and Gugino 1993; Hauser et al. 1993; Newburger et al. 1993; Chabot et al. 1997; Nuwer 1997; Pua and Bissonnette 1998; Sebel 1998; Gugino et al. 1999, 2001, 2004; Jacobson and Jerrier 2000; Johansen and Sebel 2000; Rasmussen et al. 2002; Zimpfer et al. 2002; Grimm et al. 2003; Florence et al. 2004; Freye 2005b; Freye and Levy 2005; Jameson and Sloan 2006; Markowitz et al. 2007; Williams and Ramamoorthy 2007; Nelson et al. 2008; Isley et al. 2009; Palanca et al. 2009; Brown et al. 2010; Chakravarthy et al. 2010; Golukhova et al. 2010; Poe et al. 2010; Golukhova et al. 2011; Constant and Sabourdin 2012; Futier et al. 2012)
5.4 Monitoring Depth of Anesthesia (Including Bispectral Analysis Index)
Bispectral analysis index could help us deliver an appropriate level of anesthesia and/or sedation. During postoperative period, during the time interval from patient transfer to ICU until tracheal extubation, the overall patient status (including hemodynamic, pulmonary, hematologic, and consciousness) is not yet prepared for extubation. Meanwhile, the residual effects of anesthetics are usually vanished in this time, and the patient needs some degree of sedation.
Besides, frequently it happens in a number of patients that some invasive procedures are needed (like intubation, orotracheal suctioning, central line insertion, and chest tube change), or less frequently, it happens that more time is needed for full recovery: some need prolonged intubation and mechanical ventilatory support or hemodynamic support, mandating additional sedation/analgesia. Level of sedation/analgesia should be monitored to deliver adequate analgesic agents while preventing over-administration. Bispectral analysis index monitor could help us in such cases to improve sedation/analgesia level.
Depth of anesthesia scoring for bispectral analysis index is defined as following:
>80: awake
60–80: sedation state
40–60: surgical anesthesia
<40: deep anesthesia
In some studies, bispectral analysis index is considered as the “most widely used method at the present time” for monitoring sedation as part of an integrated monitoring approach for assessment of sedation/analgesia in critical patients. Also, bispectral analysis index is demonstrated to be a “valid measure of wakefulness after cardiac surgery.”
Another important issue is the legal aspects of delivering appropriate level of sedation/analgesia to create both patient satisfaction and amnesia and so prevent patient recall (Simmons et al. 1999; Drummond 2000; Riker et al. 2001; Brocas et al. 2002; Frenzel et al. 2002; Courtman et al. 2003; Deogaonkar et al. 2004; Watson and Kane-Gill 2004; Fraser and Riker 2005; Freye 2005b; Hernandez-Gancedo et al. 2006; Payen et al. 2007; Palanca et al. 2009; Lamas and Lopez-Herce 2010).
5.5 Evoked Potentials
Evoked potentials are used as monitoring devices to check the functional integrity of the central and peripheral nervous system, especially the different neural circuits and pathways. Although both EEG and evoked potentials assess the electrical activity of the nervous system, there are some differences between these two neural monitoring, discriminating evoked potentials from EEG:
Evoked potentials have lower-voltage amplitude compared to EEG.
Evoked potentials assess the neurologic response to a stimulus (sensory or motor).
Evoked potentials are not limited to the cortical areas of the nervous system; in other words, evoked potentials monitor cortical areas, deeper neural structures of the CNS, spine, and also peripheral nervous system.
In periods when EEG is flat (like therapeutic hypothermia), evoked potentials could still work and monitor the functional integrity of the nervous system.
Evoked potentials are divided into three main categories:
1.
Somatosensory-Evoked Potential (SSEP): Somatosensory-Evoked Potential (SSEP) which monitors the functional integrity of the ascending pathways, from the peripheral receptors (median or ulnar nerve for upper extremity and posterior tibial nerve or peroneal nerve for lower extremity) up to the multiple spinal segments and then to the contralateral thalamus, reaching finally to cortex; a subtype of this monitoring modality is called visual-evoked potential (VEP) which incorporates visual stimuli as the sensory input.
2.
Motor-Evoked Potential (MEP): Motor-Evoked Potential (MEP) which monitors the motor pathway activated in response to electrical stimulus, from the cortical areas down to the related nuclei and corticospinal tracts and finally to motor units.
3.
Auditory-Evoked Potential (AEP): Auditory-Evoked Potential (AEP) which objectively monitors the neural pathway involved in hearing from cochlea in ear to the auditory (8th cranial) nerve to brainstem then related brain ganglia and finally to related cortical areas; the first milliseconds of this monitoring controls the brainstem function involved in auditory pathway and is called brainstem auditory-evoked response (BAEP).
The above modes have a relatively wide application in perioperative care, including cardiac surgical procedures. Their application in the postoperative period of cardiac surgery patients could be very useful and help us gather important objective data; however, this is not a common practice; one could stress on the following as the main indications of indications of evoked potentials in postoperative period:
During periods of postoperative-controlled (therapeutic) hypothermia, EEG becomes flat; however, evoked potentials could monitor functional integrity of the nervous system even at such states.
Monitoring the physiologic integrity of the neural system while the patient is hemodynamically unstable, deeply sedated, has altered consciousness state, or is comatose; possibly other nervous system monitoring could not assess the functional status of the patients in these periods of time.
In summary, evoked potentials could help the clinicians in assessment of neural system integrity with objective and reproducible data which are a new window besides the routine CNS monitoring; possibly, their postoperative application in cardiac surgery patients would be more common in future years (Hill and Chiappa 1994; Nuwer et al. 1995; Sloan 1995, 2004; Rodriguez 2004; Freye 2005a, b; Toleikis 2005; Sloan and Jameson 2007; Amantini et al. 2008; Martin and Stecker 2008; Sloan et al. 2012).
5.6 Cerebral Oximetry
Cerebral oximetry is a relatively new technology for cerebral monitoring though it has passed more than 35 years from the first publication regarding the in vivo application of the technique; however, it has been used in a number of procedures including cardiac surgery. Successful previous studies have shown the use of this monitor in both animal models and in human studies involving some high-risk procedures like cardiac and vascular surgery and in patients with underlying acute cerebral events though there are still some controversies.
The technology of “near-infrared spectroscopy” (NIRS) was described for this purpose in 1977 by Professor “Frans Jöbsis.” NIR light has a specific characteristic which is the power to penetrate a wide range of body tissues (including bone) and, unlike pulse oximetry, utilizes the reflection phenomenon of light rather than the process used in pulse oximetry; transmission of light from a small part of the body (e.g., a finger) is the technology used in pulse oximetry; in other words, this technology utilizes passage of near-infrared (NIR) light in the range of 700–1,000 nm, through skull and underlying tissues using self-adhesive optodes used on the scalp above the eyebrows; part of the NIR light is absorbed by biologic chromophores, especially two main chromophores oxyhemoglobin (OHb) and deoxyhemoglobin (HHb) and cytochrome oxidase, and the rest of the light is returned back; the returned portion of NIR light is used for data collection, processing, calculation, and demonstration of the absorbed fraction of light in each of the above chromophores; in this process, the “modified Beer-Lambert Law” is used for calculations; finally these data are processed by the machine software to demonstrate the figures of rSO2 on the monitor screen. NIR light is produced by “light-emitting diodes” (LEDs) and absorbed by silicon photodiodes. In summary, its mechanism is by differential absorption of near-infrared spectroscopy (NIRS) in the range of 700–1,000 nm, through skin and bone.
One of the key features of cerebral oximetry by NIRS is that rSco2 figures are in appropriate range only if the brain tissue receives adequate oxygenated blood, which mandates not only adequate cerebral oxygenation but also appropriate state of cerebral hemodynamic, patency of cerebral arteries, and efficient cerebral venous drainage. Possibly this is why in some high-risk patients like cardiac surgeries (including CABG patients), regional cerebral oxygen saturation monitoring (rSco2) prevents episodes of severe cortical hypoxia; so, application of this monitor has the potential to significantly reduce the incidence of major organ dysfunction and “improve outcome,” though some controversies exist yet and there are studies questioning the effect of cerebral oximetry application on patient outcome (especially the systematic review recently published by Zheng et al. (2013).
A number of practical notes for utilization of this monitoring are:
Monitors the in vivo state of the CNS.
Monitoring is noninvasive, real time, and portable.
Measures both oxygenation and perfusion of the CNS (i.e., mandates appropriate hemodynamic of the CNS).
Monitors not only the CNS status but also the hemodynamic status since it monitors both oxygenation and perfusion of the CNS.
A drop more than 20 % from baseline or an absolute decrease below 50 % in measured rSco2 values is considered as cerebral hypoxia.
Could be used for preoperative, intraoperative, or postoperative monitoring (all over the perioperative period).
Does not need pulsatile flow like pulse oximetry for monitoring during CPB.
Is one important member of the “multimodal CNS monitoring” in cardiac surgery.
Fine attachment and correct positioning of the probes is very important for its application, and this is a very important application point which could bias the measurements.
For patients receiving hemodynamic support by ECMO, cerebral oximetry is a very useful and promising CNS monitoring; also, if the patient would be under non-pulsatile flow with ECMO, this device could be more practical than many other CNS monitors.
Could be used as a predictor for successfulness of extubation in cardiac surgery patients.
Its application in high-risk patients (like the elderly and the patients undergoing surgery with underlying cerebral vascular disorders) is recommended more than the others.
Underlying diseases like decreased cardiac output, pulmonary problems, anemia, and underlying disorders of the cerebral vessels are considered as confounders which could decrease the rSco2 readings and create bias.
If a decrease in rSco2 is observed, the following steps are suggested by Murkin et al. and Denault et al. as a useful approach for relieving the etiologic disorder causing cerebral ischemia:
1.
Control head position; if head is rotated extensively, turn it to normal position.
2.
Check the possibility of arterial or venous occlusion due to arterial or venous cannula physical effects.
3.
Check for mean arterial pressure (MAP) and treat it if it is low.
4.
Control arterial saturation (by pulse oximeter or blood gas analysis); if it is low, rule out possible causes of systemic desaturation.
5.
Treat possible hyperventilation which could decrease arterial PaCO2, especially if it is below 35 mmHg.
6.
Treat possible anemia to increase hematocrit above 30 %.
7.
Assess the cardiac function including the situation of the heart using methods like echocardiography and also SjvO2; relieve potential underlying etiologies including treatment of failing heart.
8.
Check cerebral oxygen consumption; if it is increased, rule out and treat “convulsions” and/or “hyperthermia.”
9.
But if cerebral oxygen consumption is normal, rule out increased “intracerebral hypertension” and/or “cerebral edema”; for this purpose, use the help of imaging modalities.
(Rolfe 2000; Ferrari et al. 2004; Casati et al. 2005, 2006; Polito et al. 2006; Denault et al. 2007; Hoshi 2007; Murkin et al. 2007; Wolf et al. 2007; Wright 2007; Fischer et al. 2009; Hasegawa and Okita 2009; Huppert et al. 2009; Murkin and Arango 2009; Slater et al. 2009; Vohra et al. 2009; Brady et al. 2010; Erickson and Cole 2010; Fedorow and Grocott 2010; Green and Paklet 2010; La Monaca et al. 2010; Palombo et al. 2010; Sellmann et al. 2010; Svyatets et al. 2010; Veel et al. 2010; Andritsos et al. 2011; Bronicki and Chang 2011; Hoshi 2011; Lampe and Becker 2011; Lima et al. 2011; Radovanovic and Radovanovic 2011; Rao and Durga 2011; Roggenbach and Rauch 2011; Smith 2011; Cyrous et al. 2012; Ghosh et al. 2012; Hankey 2012; Kertai et al. 2012; Li 2012; Scheeren et al. 2012; Tsygan 2012; Foster et al. 2013; Hampton and Schreiber 2013; Moerman et al. 2013; Murkin 2013; Seule et al. 2013; Zheng et al. 2013; Zulueta et al. 2013)
5.7 Jugular Venous Oxygen Saturation (SjvO2)
Monitoring the CNS oxygenation status has been used for more than 60 years: Gibbs et al first in 1942 and then Datsur et al in 1963. Assessment of the jugular venous oxygen saturation (SjvO2) is an “indirect surrogate indicator for global oxygenation of the cortex” and also is an indicator of the balance between cerebral blood flow (CBF) and cerebral metabolism rate of oxygen (CMRO2). SjvO2 monitoring could be used to monitor the trend of change in the following items:
Oxygen uptake by the brain tissue (i.e., the difference between brain arterial and venous difference which equals CNS oxygen uptake).
Arterial and venous difference of CNS blood gases and their related parameters.
Arterial and venous difference of CNS blood glucose levels.
Arterial and venous difference of CNS blood lactate levels; impaired CNS perfusion leads to decreased cerebral oxygenation which would activate anaerobic metabolism leading to increased lactate level in the jugular venous bulb blood samples.
The normal values for SjvO2 are lower than the normal values for global mixed venous oxygen saturation (which is usually measured by pulmonary artery catheter). This is due to the fact that oxygen uptake and consumption in the cerebral tissue is much higher than the global body oxygen uptake and consumption.
5.7.1 Contraindications for SjvO2 Catheter Insertion
According to Shaaban et al. absolute contraindications for SjvO2 catheter insertion are:
Injuries in the cervical spine
Bleeding diathesis
Local neck trauma
Local infection
Also, relative contraindications could be:
Compromised drainage of the cerebral venous system
Patients having tracheostomy
SjvO2 measurements are done either using the conventional technique with serial measurements of SjvO2 and other factors or using a fiberoptic catheter with near-infrared light in the catheter tip; however, this conventional technique has the limitation of being “point assessment” needing serial measurements but could not provide real-time data. On the other side, malpositioning of the catheter tip could cause reading errors or the near-infrared light of the fiberoptic catheter may be out of its defined range, and hence, misreading would occur. Also, rotation of the head to either side could affect the venous return and distort the measurements. The technology used in fiberoptic SjVO2 monitor is similar to the oximetry technology used for some types of pulmonary artery catheters.
5.7.2 Technique of Catheter Insertion
SjvO2 is assessed through a catheter introduced to the internal jugular vein using the following technique:
The technique is through the internal jugular vein, usually the right side.
Usually, the right IJV is the dominant vein for cerebral venous drainage; however, the mixing pattern between right and left hemispheres is not always the same.
SjVO2 catheter insertion is exactly the same as central venous catheter (CVC) insertion; the anterior triangle approach is the preferred one; however, the needle and guidewire direction should be a cephalic one (compared to CVC direction).
Seldinger technique is used for guidewire insertion.
After guidewire insertion, the catheter should be inserted and conducted upward until resistance is sensed, or in the awake patient, a sense of pressure in skull base is noted by the patient; also, Doppler sonography or sizing the inserted length of the catheter by an external sizer.
The catheter is sent from the right internal jugular vein over the guidewire to the cephalic direction.
The catheter tip is sent cephalad to the “common facial vein outlet”; from there, it is sent to the jugular bulb; the jugular bulb is the dilated portion of the internal jugular vein; it is located distal to the jugular foramen (i.e., the anatomic opening in the bony skull from which the jugular vein exits).
Inside the jugular bulb, the catheter tip should be positioned in the roof of the bulb; this is the site for SjVO2 measurements.
The location of the catheter tip should be documented by lateral or anteroposterior neck X-ray; it should be located at the level of the mastoid process; in lateral neck X-ray, this location is equal to the lower border of the first cervical spine or the inferior margin of the orbital rim; also, if we draw a line between the two mastoid processes, the tip of the catheter should be just cephalad to this line.
Another way is the surface landmarks: the surface landmark for jugular vein bulb is 1 cm anterior and 1 cm below the mastoid process.
The measurements made by the catheter should be calibrated, in vivo or in vitro, per information provided by the manufacturer.
If the catheter tip is misplaced and attached to the vessel wall or if the catheter tip has move in either direction (cephalic or caudal) more than 2 cm from the bulb of jugular vein, the readings would be biased due to venous sample “contamination” and would fall in the biased range of measurement.Stay updated, free articles. Join our Telegram channel
Full access? Get Clinical Tree
