Abstract
This article reviews the role of 18 F-2-deoxy- d -glucose (FDG) positron emission tomography (PET) imaging in staging, radiation therapy planning, and determination of prognosis and therapeutic response in patients with nonsmall cell lung cancer. In addition, radiotracers that interrogate different metabolic pathways, receptors, and targets to overcome the potential limitations of FDG-PET in staging, as well as early response evaluation and monitoring of response to targeted therapies, are reviewed.
18F-2-deoxy-D-glucose (FDG) positron emission tomography (18FDG-PET) imaging has a key role in staging, radiation therapy planning, and determination of prognosis and therapeutic response in patients with non-small cell lung cancer. FDG-PET-CT is not optimal in determination of T descriptors (additional small lung nodules, locoregional invasion, etc.) as respiratory motion and or low-radiation-dose imaging degrade image quality. Radiotracers interrogate different metabolic pathways, receptors, and targets to overcome the potential limitations of FDG-PET in staging, as well as perform early response evaluation and monitoring of response to targeted therapies. A PET-CT-defined tumor target is usually smaller than that defined by CT, and incorporation of PET-CT into radiotherapy planning can allow radiation-dose escalation without increasing side effects. FDG is the only Medicare-approved PET-CT tracer for evaluation of cancer. Novel PET radiotracers that interrogate different metabolic pathways beyond glycolysis, receptors, and targets are being evaluated in staging, response evaluation, and targeted therapy assessment.
Keywords
18 F-2-deoxy-D-glucose-positron emission, FDG-PET-CT, Lung cancer, Metabolic activity, Metabolic pathways, nonsmall cell lung cancer, PET-CT, PET radiotracers, Positron emission tomography imaging, prognosis, response, staging, T descriptors
Summary of Key Points
- •
18 F-2-deoxy- d -glucose (FDG)-positron emission tomography–computed tomography (PET–CT) is routinely used clinically for diagnosis, staging, and radiation treatment planning and may have a role in prognosis and monitoring of treatment response.
- •
FDG-PET–CT is not optimal in determination of T descriptors (additional small lung nodules, locoregional invasion, etc.) as respiratory motion and or low-radiation-dose imaging degrade image quality.
- •
FDG-PET–CT has superior accuracy compared with CT in detecting hilar (N1), mediastinal (N2, N3), and extrathoracic (N3) nodal metastasis.
- •
FDG-PET–CT has superior accuracy compared with CT in detecting M1b and M1c (extrathoracic) metastasis.
- •
FDG-PET–CT detection of occult metastasis (M1b/M1c) increases as T and N descriptors increase and impact on management is greater in patients with more advanced disease.
- •
FDG uptake threshold such as standardized uptake value (SUV) is unreliable in differentiating inflammatory from metastatic disease.
- •
FDG-PET prognostic information is not dependable and may be confounded by limitations of SUV reproducibility.
- •
FDG-PET–CT metabolic tumor volume and total lesion glycolysis (which take into account tumor size and uptake of FDG) may be important prognostic factors.
- •
FDG-PET–CT may allow an early and sensitive assessment of antitumor effect after therapy.
- •
FDG-PET–CT improves accuracy of target delineation in radiation treatment planning.
- •
A PET–CT-defined tumor target is usually smaller than that defined by CT, and incorporation of PET–CT into radiotherapy planning can allow radiation-dose escalation without increasing side effects.
- •
FDG is the only Medicare-approved PET–CT tracer for evaluation of cancer.
- •
Novel PET radiotracers that interrogate different metabolic pathways beyond glycolysis, receptors, and targets are being evaluated in staging, response evaluation, and targeted therapy assessment.
PET using the radiopharmaceutical FDG, a d -glucose analog labeled with fluorine-18, complements conventional radiographic imaging for the evaluation of patients with nonsmall cell lung cancer (NSCLC). FDG-PET has an important role in the staging of NSCLC according to the tumor, node, and metastasis (TNM) system and is routinely used to improve the detection of nodal and extrathoracic metastases. FDG-PET is also currently used to improve the planning of radiation therapy and is being evaluated for the assessment of prognosis and therapeutic response. By potentially allowing for an earlier and more sensitive assessment of the effect of antitumor therapy, FDG-PET may be predictive of the outcome of treatment and the survival of patients after treatment. This chapter will discuss the use of FDG-PET for the staging of disease, the planning of radiation therapy, and the assessment of outcome and prognosis, with an emphasis on the appropriate clinical use of FDG-PET for the treatment of patients who have NSCLC. In addition, the use of novel radiotracers that interrogate different metabolic pathways, receptors, and targets to overcome the potential limitations of FDG-PET in staging, as well as early response evaluation and monitoring of response to targeted therapies, will be reviewed.
Staging of Lung Cancer
Size, Location, and Locoregional Invasion (T Descriptor)
FDG-PET is used together with CT because the integration of metabolic activity with the high-spatial resolution of CT is important for the evaluation of tumors in terms of size, location, and the degree of locoregional invasion (T descriptor) as well as for the determination of the anatomic location of regions of focally increased FDG uptake. It is important to be aware that the relatively poor spatial resolution of PET limits its utility for the evaluation of the primary tumor. However, the CT component of integrated PET–CT also has shortcomings in terms of its ability to accurately demonstrate T descriptors such as the presence of additional small lung nodules and locoregional invasion as it is often performed during respiration and with a low-radiation-dose imaging protocol, both of which can compromise image quality. Nevertheless, FDG-PET improves the detection of nodal and distant metastases and frequently alters the treatment of patients. Accordingly, this review of TNM staging will focus on the important role of PET imaging for the detection of nodal and distant metastases at the time of initial staging according to the seventh edition of the American Joint Committee on Cancer TNM staging system and will reference the proposals for the forthcoming eighth edition when applicable.
Regional Lymph Nodes (N Descriptor)
The presence and location of nodal metastasis (N descriptor) are important when determining the treatment of and prognosis for patients with NSCLC and, because these descriptors adequately predict prognosis, they will be maintained in the forthcoming eighth staging system. Lymph node maps, in which the node stations are numbered according to anatomic structures, are commonly used in an attempt to ensure uniformity when designating the clinical and pathologic extent of nodal metastases. There is no universally accepted nodal map and the International Association for the Study of Lung Cancer has proposed a new lymph node map that reconciles the differences among the currently used maps. However, while a precise and universally accepted nomenclature to describe lymph node involvement is essential for selecting appropriate therapy and assessing the outcomes of treatment, a major shortcoming of clinical staging is the use of nodal size to detect metastatic disease. Toloza et al., in a meta-analysis of 20 studies (3438 patients) that was performed to evaluate the use of CT for staging of the mediastinum, found that the use of a short-axis diameter of more than 1 cm as the threshold for the detection of nodal metastasis was associated with a pooled sensitivity of 57% and a pooled specificity of 82%.
FDG-PET is more accurate than CT for staging of the mediastinum in terms of nodal involvement, and FDG-PET is being increasingly integrated into both surgical and radiation oncology treatment strategies for patients with NSCLC ( Fig. 22.1 ). Birim et al., in a meta-analysis of 17 studies (833 patients) in which PET was compared with CT for nodal metastasis detection in patients with NSCLC, reported that the overall sensitivity and specificity of FDG-PET for detecting mediastinal lymph node metastases were 83% (range, 66–100%) and 92% (range, 81–100%), respectively, whereas those of CT were 59% (range, 20–81%) and 78% (range, 44–100%), respectively. In addition, improvement in the accuracy of nodal metastasis detection has been reported in association with the use of integrated PET–CT as compared with the use of CT ( p = 0.004) and PET ( p = 0.625) separately. However, in a recent study of 159 patients with NSCLC, the use of PET–CT for mediastinal N determination was associated with low sensitivity and accuracy. Based on the evaluation of 1001 nodal stations (723 mediastinal, 148 hilar, and 130 intrapulmonary), the sensitivity, specificity, and accuracy of PET–CT for the detection of mediastinal nodal metastasis were 45.2%, 94.5%, and 84.9%, respectively. The sensitivity of PET–CT for the detection of malignant involvement was 32.4% (12 of 37) for nodes measuring less than 10 mm and 85.3% (29 of 34) for nodes measuring 10 mm or more. Although infrequently addressed, the timing of PET–CT imaging may affect N determination. Ideally, PET imaging for the evaluation of nodal metastasis in patients being evaluated for surgical resection should be performed in close temporal relationship to the anticipated date of resection. In this regard, there may be a meaningful association between the sensitivity of PET–CT and the time from imaging to resection. Booth et al. reported that the sensitivity and accuracy of PET–CT for N2 nodal detection were 64% and 94%, respectively, when performed less than 9 weeks before pathologic sampling, compared with 0% and 81%, respectively, when performed 9 weeks or more before pathologic sampling.

Despite the superior accuracy of FDG-PET–CT over CT in terms of N determination, a limitation is the overlap in the appearance of malignant and benign lymph nodes as microscopic nodal metastases normally are not FDG-avid, whereas inflammatory lymph nodes can be FDG-avid. The use of an FDG uptake threshold such as the maximum standardized uptake value (SUV max ) to differentiate inflammatory from metastatic nodal disease has limited utility for clinical N determination as numerous factors, including the time to imaging after FDG administration and the type of scanner and image reconstruction algorithm used, can affect the thresholds selected. We are not aware of any prospective multicenter trial that has validated an FDG uptake threshold, and visual interpretation currently tends to be more accurate than SUV quantification. When mediastinal lymph nodes are FDG-avid as determined on the basis of visual analysis or SUV, the number of false-positive results due to infectious or inflammatory etiologies is too high to allow for a confident diagnosis of nodal metastasis ( Fig. 22.2 ). In this regard, because the positive predictive value of FDG-PET is not optimal for diagnosing nodal metastasis, invasive sampling should be performed to confirm pathologically involved nodes (pN) disease when the PET and CT findings are indicative of nodal metastasis or when the CT and PET findings are incongruent. In addition, even if PET–CT is negative for mediastinal nodal metastasis, the need for histologic confirmation persists because of the low sensitivity and accuracy of PET–CT for intrathoracic nodal staging. However, it is important to emphasize that the precise role of FDG-PET with regard to invasive nodal evaluation is unclear. Although not universally accepted, the American College of Chest Physicians evidence-based practice guidelines recommend that invasive confirmation of the mediastinal nodes is not needed in patients with a peripheral clinical stage I NSCLC if PET of the mediastinum is negative. Furthermore, a meta-analysis of 10 studies (1122 patients) indicated that occult nodal metastasis is not infrequent in patients with clinical stage T1-2N0 NSCLC but that PET and CT provide a favorable negative predictive value for the detection of mediastinal metastasis (0.94 for T1 disease and 0.89 for T2 disease), suggesting a low yield from invasive clinical staging for this subgroup of patients.

In an attempt to determine the need for invasive sampling after PET and CT imaging, de Langen et al. performed a meta-analysis to evaluate the association between the size of mediastinal nodes and the probability of malignancy. The authors reported a 5% posttest probability for N2 disease in patients with a negative result on FDG-PET imaging in whom the mediastinal nodes measured 10 mm to 15 mm on CT and suggested that these patients should be treated with thoracotomy. In comparison, they reported a 21% posttest probability for N2 disease in patients with a negative result on FDG-PET imaging in whom the lymph nodes measured more than 16 mm on CT and suggested that these patients should have mediastinoscopy prior to thoracotomy. For patients with a positive result on FDG-PET, the posttest probability of malignancy was 62% when the nodes measured 10 mm to 15 mm on CT and 90% when the nodes measured more than 16 mm. Although the authors did not suggest any management strategies regarding histologic confirmation of nodal metastasis when the result on FDG-PET is positive, N2 and N3 disease should be histologically confirmed in patients who are potentially eligible for resection or adjuvant therapy.
The expanded role of nonsurgical management for patients with early-stage NSCLC and the definitive use of FDG-PET–CT to detect mediastinal nodal metastasis in high-risk patients or patients with advanced disease who are being evaluated for nonsurgical management underscore the importance of accurate nodal evaluation. In this regard, there have been attempts to improve the detection of mediastinal nodal metastasis when using FDG-PET–CT in the staging algorithm. The SUV max of the primary tumor has been used to predict the likelihood of microscopic nodal metastatic disease and to improve the accuracy of N staging. Trister et al. reported that a high SUV for the primary tumor was an independent predictor of occult mediastinal nodal metastasis in patients with clinical stage I and II NSCLC and recommended invasive staging of the mediastinum when the SUV is more than 6. In addition, in a study of 265 patients with NSCLC, Miyasaka et al. reported that the SUV max of the primary tumor was a significant predictor of pathologic nodal involvement, with pN1-2 disease being detected in 25 (41%) of 61 in whom the SUV max was more than 10, compared with only 26 (12.7%) of 204 patients in whom it was less than 10 ( p < 0.0001). The detection of nodal metastasis also may be improved by the use of point spread function (PSF) reconstruction along with PET–CT scanners. PSF reconstruction recently became commercially available, and, as a result of improved image contrast and reduced image noise, may be more sensitive for the detection of small-volume nodal metastases. Lasnon et al. reported that PSF PET had higher sensitivity (97%), negative predictive value (92%), and negative likelihood ratio (0.04) than did conventional iterative reconstruction ordered subset expectation maximization PET (78%, 57%, 0.31, respectively) for nodal evaluation in patients with NSCLC. While improved sensitivity increases the likelihood of false-positive results, the authors concluded, on the basis of the significant improvements in sensitivity ( p = 0.01), negative predictive value ( p = 0.04), and low negative likelihood ratio that were observed in association with PSF reconstruction, that preoperative invasive nodal staging may be omitted in cases in which the result of PSF FDG-PET–CT is negative. A potential further advance in nodal assessment is the use of an artificial neural network (ANN). Toney and Vesselle recently reported that an ANN overcame the subjectivity associated with the interpretation of PET, outperformed an expert FDG-PET–CT reader in terms of accuracy, and differentiated malignant and benign inflammatory lymph nodes with overlapping appearances on PET–CT. The ANN used four FDG-PET–CT-derived input parameters (primary tumor SUV max ; tumor size; node size; and FDG uptake at the N1, N2, and N3 stations) and correctly predicted the N descriptor in 99.2% of cases, compared with 72.4% for the expert reader.
Distant Metastasis
Distant metastases (M descriptor) are common in patients with NSCLC at the time of presentation and are currently subclassified into M1a metastases (additional nodules in the contralateral lung, malignant pleural effusion, pleural nodule[s], pericardial nodule[s]) and M1b (extrathoracic) metastases. The forthcoming eighth edition proposals for the M descriptor maintain the M1a descriptor but the M1b will now be assigned to cases with a single extrathoracic metastasis, and M1c to those with multiple extrathoracic metastases in one or more organs.
Although FDG-PET–CT can be useful in the evaluation of the intrathoracic and extrathoracic metastases, the role of FDG-PET in detecting M disease is not clearly defined. For instance, patients with early clinical stage (T1 N0) NSCLC have a low incidence of occult metastasis; thus extensive evaluation for metastasis in these patients may not be warranted. Viney et al. performed a randomized controlled trial to determine the appropriate role of FDG-PET in the clinical treatment of patients who have early stage NSCLC. In that study, 183 patients with early-stage lung cancer (>90% of who had T1 2N0 involvement) were assigned to conventional workup (92 patients) or to conventional workup and PET (91 patients). Compared with conventional staging, PET confirmed staging in 61 patients, staged the tumors as benign in two patients, and upstaged the tumors in 22 patients, including 11 patients with N2 nodal metastatic disease and two patients with pleural metastasis. Distant metastases were rarely detected with PET (2 of 91 patients; <5%). Overall, the results of PET could have resulted in a change in treatment for 26% of the patients, with the avoidance of thoracotomy in 11 of 91 patients and with 13 of 91 potentially receiving neoadjuvant chemotherapy or chemoradiation therapy. However, because the general policy of the participating surgeons was to operate on patients with apparently completely resectable stage IIIA disease without any further evaluation, PET resulted in further investigation or other management changes in only 12 patients (14%).
In patients with more advanced disease, whole-body FDG-PET–CT has a greater impact on the accuracy of staging as well as on management. The American College of Surgeons Oncology Group reported that PET had a sensitivity, specificity, positive predictive value, and negative predictive value of 83%, 90%, 36%, and 99%, respectively, for M1 disease. Whole-body PET imaging stages intrathoracic and extrathoracic disease in a single study and detects occult extrathoracic metastases in up to 24% of patients who are selected for curative resection ( Fig. 22.3 ). The incidence of detection of occult metastases has been reported to increase as the T and N descriptors increase (from 7.5% in early-stage disease to 24% in advanced disease). In two studies with a relatively high proportion of patients with more advanced lung cancers that were considered to be resectable on the basis of standard clinical staging, PET imaging prevented nontherapeutic surgery in one of five patients. A more recent prospective study, similar to the PLUS multicenter randomized trial conducted by van Tinteren et al. but evaluating PET–CT rather than dedicated PET, demonstrated that 52 (63%) of 83 patients in the PET–CT group underwent surgery, with 13 (25%) of 52 thoracotomies being futile. By contrast, in the conventional staging group, 73 (80%) of 91 patients underwent thoracotomy, with 38 (52%) of 73 thoracotomies being futile.

The widespread use of FDG-PET has changed the imaging algorithm that is used to detect metastases to specific organ sites, particularly the osseous skeleton, the adrenal glands, and extrathoracic lymph nodes. In this regard, FDG-PET–CT is particularly effective for detecting bone metastasis. A meta-analysis of 17 studies demonstrated that the pooled sensitivity and specificity for the detection of bone metastasis were 92% and 98%, respectively, for FDG-PET–CT, compared with 86% and 88%, respectively, for bone scintigraphy. As a result, FDG-PET–CT has to a large extent replaced 99mTc-methylene-diphosphonate (MDP) bone scintigraphy for the evaluation of possible bone metastasis in patients with NSCLC ( Fig. 22.4 ). Specifically, 99mTc-MDP bone scintigraphy now has no additional utility for patients with NSCLC if FDG-PET is performed as part of the staging algorithm. In fact, discordant findings of skeletal metastasis between 99mTc-MDP scintigraphy and FDG-PET–CT have been reported to occur in 20% of patients with NSCLC. This discordance is in large part due to the ability of FDG-PET to detect early bone metastasis and the failure of 99mTc-MDP scintigraphy to detect early neoplastic infiltration of bone marrow. FDG-PET–CT is also useful for detecting adrenal metastasis and for distinguishing benign from malignant adrenal masses that are detected with CT. A meta-analysis of 21 studies (1391 lesions), including five studies that specifically focused on patients with lung cancer, demonstrated that FDG-PET had a combined sensitivity and specificity of 94% and 82%, respectively, for the detection of adrenal metastasis in patients with lung cancer. Similar to the assessment of bone metastasis, FDG-PET has changed the imaging algorithm used to evaluate an indeterminate adrenal mass detected with CT and is now often used as the definitive imaging modality rather than magnetic resonance imaging (MRI), particularly when the adrenal mass is small ( Fig. 22.5 ). In fact, adrenal masses can be characterized with use of FDG-PET, and subsequent imaging is usually unnecessary. If an adrenal mass in a patient with potentially resectable NSCLC has normal FDG uptake on PET, curative resection should be considered without further evaluation. If an adrenal mass has increased FDG uptake, biopsy should be performed to confirm metastatic disease. FDG-PET–CT has limitations in the evaluation of liver and brain metastases. Specifically, because of the high background uptake of FDG by normal brain tissue, the ability to detect brain metastasis is not optimal. MRI is the current standard of care for patients with NSCLC who are undergoing evaluation of possible brain metastases, and FDG-PET–CT lacks the sensitivity and specificity to replace this diagnostic test. Similarly, FDG-PET–CT has a limited role in the detection of hepatic metastases. Although FDG-PET–CT has a high specificity for the detection of occult hepatic metastases, it has a low sensitivity, and, accordingly, FDG-PET is not routinely used for this purpose.


Whole-body FDG-PET imaging improves the accuracy of staging in patients with NSCLC. However, focal increased uptake of FDG in extrathoracic lesions that are unrelated to the primary NSCLC can mimic distant metastasis. Accordingly, all extrathoracic FDG-avid lesions that potentially would alter patient management should be further imaged or biopsied to confirm the diagnosis of distant metastasis. The rationale for this management approach is supported by the results of a prospective study that was performed to assess the incidence and diagnosis of a single site of extrapulmonary accumulation of FDG in patients with newly diagnosed NSCLC. Of the 350 patients in the study group, 72 had solitary FDG-avid lesions. Sixty-nine of these patients underwent biopsy of the lesion; of these, 37 (54%) had a solitary metastasis, whereas 32 (46%) had a lesion that was unrelated to the NSCLC, such as a benign tumor or inflammatory lesion (26 patients) or a clinically unsuspected second malignancy or recurrence of a previously diagnosed carcinoma (6 patients).
Prognosis
The widespread use of CT and the increase in lung cancer screening programs have resulted in the detection of small lung cancers, typically adenocarcinomas, with indolent to aggressive malignant behavior. A recent multicenter study involving 610 patients with clinical stage IA lung cancer validated the ability of FDG-PET–CT together with high-resolution CT to predict the malignant behavior and prognosis of early adenocarcinomas of the lung ( Fig. 22.6 ). The diameter of the primary tumor was 20 mm or less in 354 patients and more than 20 mm in 256 patients. The mean duration of follow-up after surgery was 41.8 months, and the rate of disease recurrence was 9.5% (58 patients). A significant difference in recurrence-free survival was identified between tumors with an SUV max of 2.9 or less and those with an SUV max of more than 2.9 (5-year recurrence-free survival ratio, 95% compared with 72%; p < 0.001). In addition, SUV max was a significant prognostic factor for cancer-specific survival ( p < 0.001). Furthermore, when combined with a high-resolution CT ground-glass opacity ratio {(1 – [maximum dimension of solid component of tumor on lung windows/maximum dimension of tumor on lung windows]) × 100}, the data were especially useful for predicting the malignant grade of tumors and patient prognosis. The prediction of the biologic behavior of small adenocarcinomas is important for the selection of the appropriate surgical option. In this regard, the frequency of lymphatic, vessel, or pleural invasion was only 2% among tumors with an SUV of 2.9 or less and a ground-glass opacity ratio of 25% or more, and the 1% incidence of nodal metastasis or recurrence in this group suggests that sublobar resection, rather than lobectomy, could be considered as definitive management. The use of SUV to identify patients with clinical stage IA lung cancers that are appropriate for limited resection was also supported by a report involving 183 patients with clinical stage IA NSCLC who were evaluated with PET–CT and underwent resection. The 5-year recurrence rate was 0% for patients with a corrected SUV (the ratio of the tumor SUV max to the liver SUV mean ) of less than 1.0, compared with 22.9% for those with a corrected SUV of 1.0 or more, and the 5-year cancer-specific survival rates for these groups were 100% and 88.7%, respectively.

The level of increased FDG uptake in the primary tumor at the time of diagnosis also has been used to predict prognosis, and this level may be prefaced on the correlation of the SUV max of the primary tumor with tumor differentiation, necrosis, pathologic type, size, and epidermal growth factor receptor (EGFR) protein expression. Bille et al. evaluated the prognostic significance of SUV max of the primary tumor in a study of 404 patients with NSCLC who underwent potentially curative resection after PET–CT. SUV max of the primary tumor was significantly associated with survival ( p = 0.00016). The median survival, 2-year survival, and 5-year survival rates were 26.4%, 88.4%, and 72.1%, respectively, for the 209 patients with an SUV max of less than 8.6, compared with 19.6%, 71%, and 47.8%, respectively, for the 195 patients with an SUV max of 8.6 or more. Because a high tumor SUV max potentially could allow more appropriate use of neoadjuvant and adjuvant therapy, a subgroup analysis of patients with stage I disease (for whom international guidelines do not recommend adjuvant treatment) was performed; this analysis showed that the SUV did not independently predict survival. However, patients with stage II, III, or IV disease who had received adjuvant chemotherapy had better survival if the SUV max was below 8.7, and the authors postulated that these patients would benefit most from targeted adjuvant therapy. These findings are consistent with the current consensus that adjuvant chemotherapy after complete resection can result in a significant and clinically meaningful survival advantage for patients with stage II to IIIA NSCLC although not for patients with stage 1B disease. However, Cerfolio et al., in a retrospective study of 315 patients who underwent complete resection of NSCLC, found that patients with stage IB and stage II disease who had SUV values that were greater than the median value for their respective stages had lower disease-free survival rates at 4 years. The differences in disease-free survival between patients with stage IB disease (92% for the low SUV group, compared with 51% for the high SUV group) and stage II disease (64% for the low SUV group, compared with 47% for the high SUV group) were significant ( p = 0.005 and 0.044, respectively). When the results were stratified according to stage, the actual 4-year survival rates for the low and high SUV groups were 80% and 66%, respectively, for patients with stage IB disease; 64% and 32%, respectively, for those with stage II disease; and 64% and 16%, respectively, for those with stage IIIA disease.
Performance status and stage are firmly established as prognostic factors for patients with NSCLC, and small studies have indicated that FGD-PET may also be useful for determining the prognosis for patients with early and advanced NSCLC. However, a prospective National Cancer Institute–funded American College of Radiology Imaging Network/Radiation Therapy Oncology Group cooperative group trial evaluating posttreatment FDG-PET at approximately 14 weeks after radiotherapy in patients with stage III NSCLC demonstrated limited utility for predicting the prognosis. Two hundred and twenty-six patients had pretreatment FDG-PET, and 173 patients had posttreatment FDG-PET. Pretreatment SUV peak and SUV max values were not associated with survival, although a posttreatment SUV peak value of more than 7 was significantly associated with survival ( p < 0.001). Overall survival (OS) based on the study’s prespecified posttreatment SUV peak cutoff value of 3.5 was not significantly different in patients with an SUV peak less than 3.5 compared with those with an SUV peak greater than 3.5 ( p = 0.29). The authors concluded that the use of a simple posttreatment SUV cutoff value of 3.5 after chemoradiotherapy is not useful for the clinical management of patients. The authors postulated that because patients with a high SUV (>7) after chemoradiotherapy have poor outcomes, this subpopulation could be considered for early additional treatment.
FDG-PET studies are often limited by small size, their retrospective nature, and variations in treatment protocols, particularly for patients with advanced-stage NSCLC. These factors, in part, may account for the contradictory reports in the literature regarding the applicability of prognostic information provided by FDG-PET for patients with NSCLC. Hoang et al. performed a retrospective review of 214 patients with advanced-stage NSCLC who underwent FDG-PET at the time of the initial diagnosis. Univariate and multivariate analysis provided no evidence that the survival times were significantly different for patient subgroups that were defined according to SUV max ; the median survival time was 16 months for the 106 patients in whom the primary tumor had an SUV max of less than 11.1, compared with 12 months for the 108 patients in whom it had an SUV max of 11.1 or more. In addition, improved outcomes may be confounded by PET-induced stage migration and selection bias. In a retrospective analysis involving 12,395 patients with NSCLC in the pre-PET and PET periods, there was a 5.4% decrease in the number of patients with stage III disease and an 8.4% increase in the number of patients with stage IV disease in the PET period, corresponding with an increase in PET use from 6.3% to 20.1%. The use of PET predicted better survival for patients with stage III and stage IV disease. These data support the notion that stage migration is at least partially responsible for an apparent improvement in the survival of these patients.
A limitation of the studies in which the level of increased FDG uptake (as indicated by SUV) is related to survival is that the threshold SUV used for analysis ranges widely and reproducibility may not be robust. To improve reproducibility of SUV between scanners, some investigators have proposed that a ratio of tumor SUV max to either liver or blood SUV max be used rather than an absolute SUV max . The use of a ratio potentially overcomes the issue of the appropriate threshold SUV max to use for the purpose of predicting survival. Additionally, the ratio potentially can eliminate the effects on SUV related to the use of different data-acquisition and reconstruction-processing protocols at different institutions. Westerterp et al. reported differences in SUV quantitation of as much as 30% among three different institutions. Furthermore, variables such as the time between the administration of FDG and image acquisition, blood glucose level, and respiratory motion during image acquisition can affect SUV. The European Association of Nuclear Medicine has published procedural guidelines to provide a minimum standard for the acquisition and interpretation of PET and PET–CT in order to decrease the variability of SUV and to allow comparison of multicenter trials. A standardized PET protocol has been instituted in The Netherlands and serves as a model for wider application. The standardization includes (1) patient preparation, (2) matching of scan parameters such as scan time per bed position and image acquisition mode (two-dimensional or three-dimensional), (3) matching of image resolution by prescribing reconstruction settings, (4) matching of data-analysis procedures by defining volume-of-interest methods and SUV calculations, and (5) a quality-control procedure for verification of scanner calibration and the use of a National Electrical Manufacturers Association image-quality phantom for the verification of activity concentration recoveries. In a recent multi-institutional trial, a National Electrical Manufacturers Association body phantom was used to determine a correction coefficient for each scanner that was then used to standardize the SUV data. In addition, protocol-optimized images and compliance with European Association of Nuclear Medicine guidelines have been reported to allow for a reliable pretherapy and posttherapy evaluation when using different-generation PET systems.
While the quantitative analysis of PET for the determination of prognosis may be confounded by the limitations of SUV reproducibility, other proposed techniques, including dual-phase PET and assessment of metabolic tumor burden, may improve the ability to determine prognosis. Houseni et al. reported that the change in SUV max between early and delayed PET imaging during a single study was a strong independent predictor of prognosis for patients with adenocarcinoma of the lung. The median survival time was 15 months for patients in whom SUV max increased by more than 25% between the two time points, compared with 39 months for those in whom SUV max increased by less than 25%. In addition, FDG-PET–CT-assessed parameters reflecting metabolic tumor burden, such as metabolic tumor volume (MTV) and total lesion glycolysis (TLG), which take into account the size of the tumor as well as the uptake of FDG, are currently being investigated as prognostic factors. Im et al., in a recent meta-analysis of 13 studies (1581 patients) performed to evaluate the prognostic value of MTV and TLG, reported that lung cancer patients with high MTV had a worse prognosis with a hazard ratio (HR) of 2.71 (95% confidence interval [CI], 1.82–4.02, p < 0.00001) for adverse events and an HR of 2.31 (95% CI, 1.54–3.47, p < 0.00001) for death. Similarly, patients with high TLG had a worse prognosis with an HR of 2.35 (95% CI, 1.91–2.89, p < 0.00001) for adverse events and an HR of 2.43 (95% CI, 1.89–3.11, p < 0.00001) for death. Importantly, the prognostic value of MTV and TLG remained significant in a subgroup analysis according to TNM stage. Measurements of whole-body metabolic tumor burden with MTV and TLG have also been reported to be better prognostic indicators than SUV max and SUV mean in patients with NSCLC who are being assessed for surgical resection as well as for those with advanced disease who are receiving chemotherapy. In a retrospective study of 106 patients (including 19 with stage I–II and 87 with stage III–IV lung adenocarcinoma), the MTV and TLG of each malignant lesion were measured prior to treatment and were summated to give whole-body MTV and whole-body TLG values for each patient. Univariate survival analysis of patients with stage III–IV disease identified high whole MTV values (≥90) and high whole TLG values (≥600) as being significant predictors of poor progression-free survival (PFS; p < 0.001) and poor prognosis ( p < 0.001). Multivariate survival analysis identified high whole MTV values and high whole TLG values as being independent predictors of poor PFS ( p < 0.001) and prognostic predictors of poor OS ( p < 0.001). However, in a survival analysis of patients with stage I–II disease, MTV and TLG were not independent prognostic predictors. Similarly, in a retrospective study of 50 patients who underwent stereotactic body radiation therapy (SBRT) for stage I NSCLC, MTV and TLG were not correlated with OS. However, the role of volume-based parameters of FDG-PET–CT in patients with stage I NSCLC after SBRT is unclear and requires further evaluation. Another recent study of 88 patients with stage I NSCLC (including 68 patients with T1 N0 M0 disease and 20 with T2a N0 M0 disease) who had FDG-PET–CT and then SBRT indicated that MTV and TLG were significantly associated with disease-free survival.
Because of the inherent limitations of data obtained from retrospective studies with small numbers of patients, the role of FDG-PET in the treatment of NSCLC remains unclear. Cerfolio et al. posed several questions regarding the appropriate use of FDG-PET for patients with NSCLC that are worthy of consideration: (1) Should patients with an early clinical-stage NSCLC and a primary tumor with a high SUV undergo more extensive imaging prior to resection to detect occult metastases? (2) Would a patient with an early-stage lung cancer who has a high SUV benefit more from adjuvant therapy than one with a low SUV, and would such a patient also benefit from neoadjuvant therapy? (3) Should the SUV be considered together with the clinical stage when determining therapy? and (4) Should patients with a high SUV have more intensive postoperative surveillance? While the definitive answers to these questions require multi-institutional prospective randomized trials, the evolving experience with FDG-PET indicates that FDG-PET will have an expanded role in the treatment of NSCLC.
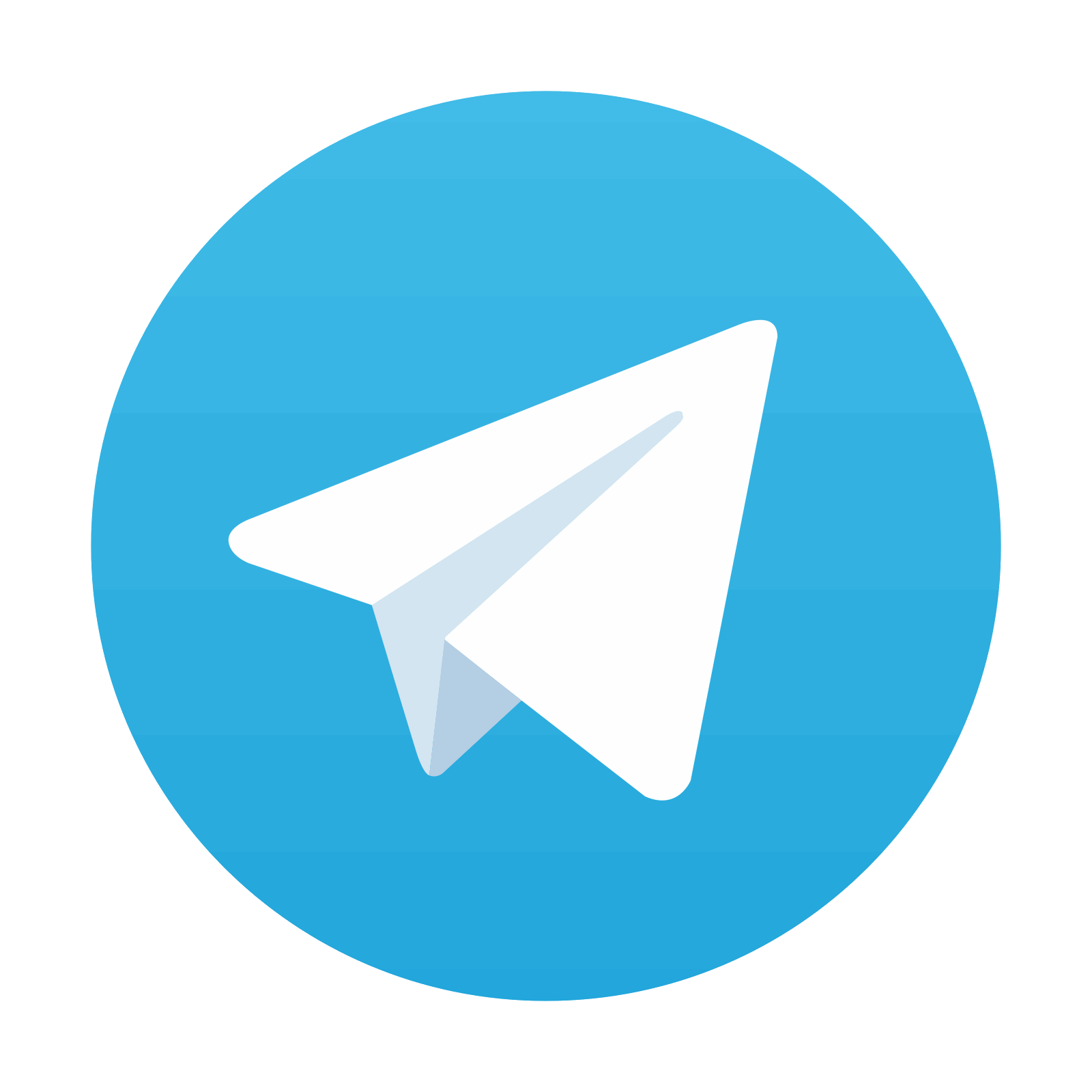
Stay updated, free articles. Join our Telegram channel

Full access? Get Clinical Tree
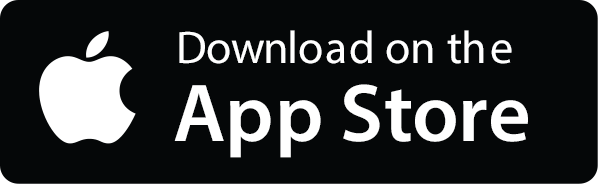
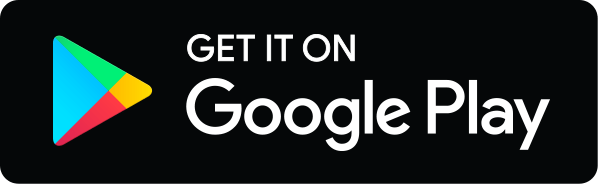