Chapter 9
Placement of Central Catheters in Specific Circumstances
Several specific entities, some of which do not appear to be related to each other, are discussed in this chapter. These entities are of major concern because of abnormalities in hemostasis or immunity. Therefore, brief reviews of the hemostasis pathways and immunity/inflammation will be mentioned first, followed with a discussion of the entities.
BONE MARROW
The medullary cavity of certain flat bones (sternum, pelvis, ribs, and skull) contains red bone marrow/myeloid tissue. Components of myeloid tissue are: (1) a heterogeneous population of developing blood cells; (2) vascular connective tissue stroma supported by a meshwork of collagenic and reticular fibers; (3) fat storage cells; and (4) sinusoids (thin-walled vascular channels consisting of simple squamous endothelium that allow newly formed blood cells to enter the bloodstream).
All types of blood cells are derived from a single type of ancestral stem cell called the pleuripotential hemopoietic stem cell. Stem cells remain poorly differentiated and uncommitted except that they will form blood cells of some kind. They are able to proliferate extensively and renew themselves. The myeloid tissue contains a mixture of stem cell offspring at various stages of differentiation.
The hemopoietic stem cell has the potential to differentiate into several different lines of specialized cells. Initially, the progeny of stem cells become restricted to undergo lymphoid (producing lymphocytes and plasma cells) or myeloid (producing other types of blood cells, such as megakaryocytes, erythrocytes, granulocytes, and monocytes) differentiation. The restricted orders of stem cells then give rise to progenitor cells committed to single lines of lymphoid or myeloid differentiation.
IMMUNE SYSTEM
The immune system consists of complex interactions between antibody production, cellular immunity, and components of the complement system and phagocyte function.
Lymphocytes
As previously discussed, the bone marrow stem cells may become restricted to the lymphoid line of differentiation and then differentiate further into two divergent and immunologic active populations: B lymphocytes and T lymphocytes and their subsets. The lymphocytes continuously interchange between blood and lymph (recirculation) to bring them into contact with any foreign antigen present in the body. The cells have the unique capacity to recognize antigen and respond to it. The production of circulating immunoglobulins in response to an antigen is termed the humoral antibody response and is mediated by B lymphocytes and their progeny (plasma cells), although helper T cells are required for this response. When a small lymphocyte responds to the antigen for which it is programmed, it develops intense cytoplasmic basophilia, enlarges rapidly, duplicates its DNA, and embarks in a series of divisions that generates a whole clone of identically programmed cells. This clone of cells then may respond to the antigen or the antigen-bearing cells. T lymphocytes mediate a wide range of immunologic activities. Individual T cells are programmed during differentiation to express only a limited range of functions (i.e., cytotoxic cells, helper T cells, or suppressor T cells). The T lymphocytes’ importance in resistance to infection is through macrophage activation by lymphokines and cytotoxicity of virus-infected cells. T lymphocytes play a central role in resistance to infection by a variety of facultative intracellular microorganisms. Other functions of T lymphocytes include delayed hypersensitivity; immunologic memory; allograft rejection; destruction of neoplastic cells; and release of cytotoxic, chemotactic, and macrophage-reactive factors.
Inflammation
The acute phase of inflammation is of relatively short duration, lasting several hours, and is stereotypic, regardless of the nature of the injury. The main characteristics of acute inflammation are exudation of fluid and plasma proteins (edema) and the emigration of leukocytes from the bloodstream to inflamed tissues. Changes in vascular permeability and vasodilatation are responsible for the heat and redness associated with acute inflammation. The accumulation of leukocytes (predominantly neutrophils and monophils) is the most important feature of the inflammatory reaction. After 24 to 48 hours, the chronic inflammation response is less uniform and longer in duration. Lymphocytes and macrophages replace the short-lived neutrophils. Proliferation of blood vessels and connective tissue also takes place.
Neutrophils
Neutrophils have only one known function: to destroy microorganisms. The steps in neutrophils’ response in host defense against invading microbes are mobilization, adherence, locomotion, Chemotaxis, phagocytosis, and intracellular killing. Microbial invasion is followed by mobilization of both mature and round (band) forms of neutrophils from the bone marrow, resulting in the “left shift” present in many acute bacterial infections. The neutrophils adhere to the vascular endothelium and enter the tissue by locomoting between the endothelial cells (diapedesis). Once in the tissues, Chemotaxis (directed migration) occurs as the neutrophils move in the direction of increasing concentrations of attractants (chemotactic factors). The most important chemotactic agents for the neutrophils (and also monocytes) are bacterial products, components of the complement system, and products of the lipooxygenase pathway of the arachidonic acid metabolism. As the neutrophils accumulate at the site of inflammation, contact with the microorganisms initiates phagocytosis. Many microorganisms resist ingestion by the neutrophils and can be engulfed only after being opsonized. Opsonins are antibodies or components of the complement system that coat the microbial surface and render it more ingestible. Opsonizing antibodies act as ligands between organisms and phagocytic cells. Neutrophils have specific membrane receptors for these opsonins, which enhances their initial attachment and ultimately ingestion. The microbes are engulfed by the neutrophils and destroyed by a variety of mechanisms, including a variety of oxygen-dependent and oxygen-independent microbicidal systems.
Complement System
Complement is a generic term for a group of distinct proteins that normally exist in plasma in inactive precursor forms but are activated during immunologically induced inflammation. This system functions by mediating a series of biologic reactions, all of which serve in the defense against microbial agents. The complement system is activated by antigen–antibody complexes or by such stimuli as bacterial endotoxins. When activated, these components have the ability to increase vascular permeability, attract leukocytes, opsonize microbial agents prior to phagocytosis, immobilize cells at the site of inflammation, and impair cell membrane function (which may lead to osmotic lysis of target organisms and cell death).
STATES OF IMMUNODEFICIENCY
As described, the immune system consists of multiple components, including antibody production, cellular immunity, and components of the complement system and phagocytic function. Immunodeficiency can result from disruption of any of these components in the complex chain of events. The following lists of causes of immunodeficiency are organized according to their functional deficiency.
Antibody-Deficiency Disorders
Considered as a whole, immunoglobulin deficiencies are not uncommon, occurring in about 1 in 600 in the general population. Several or only one of the immunoglobulin classes can be affected, and antibody production can be totally or partially disrupted (agammaglobulinemia versus hypogammaglobinemia). Clinically, infections in these patients frequently involve the sinopulmonary tract, ears, meninges, and skin. Bacteremia with metastatic spread of infection is not uncommon. Severe viral infections are uncommon. Recurrent infections with high-grade extracellular encapsulated pathogens (pneumococci, streptococci, and Haemophilus organisms) are typical entities in this category, which includes the following:
• Congenital X-linked hypogammaglobulinemia (Bruton type): This condition is marked by nearly complete absence of B cells in the circulation and becomes apparent at 5 to 6 months of age.
• Common variable immunodeficiency: This deficiency does not become apparent until age 15 or older. The presenting disease is often chronic progressive bronchiectasis. The B cells are either absent or unable to differentiate nto immunoglobulin-producing plasma cells.
• Transient hypogammaglobulinemia of infancy.
• Selective immunoglobulin A (IgA) deficiency: Most affected subjects are well clinically but may have sinopulmonary infections, atopy, gastrointestinal disorders, autoimmune disease, and malignancy.
Cellular Immunodeficiency Disorders
Acquired immunodeficiency syndrome (AIDS) is the most common acquired cellular immunodeficiency disorder; the human immunodeficiency virus (HIV) impairs normal T-cell function. Other causes of secondary immunodeficiency include chemotherapy, radiation therapy, and bone marrow transplantation (BMT). Congenital thymic hypoplasia (DiGeorge syndrome) is a rare condition that is a relatively pure form of T-cell immunodeficiency. Because of a hypoplastic thymus, these infants do not develop T cells and lack any form of cell-mediated immunity. The serum immunoglobulin concentrations are usually normal or elevated in these disorders, but B-cell function is compromised because of a deficiency of T-helper cells. Clinical characteristics of these conditions include recurrent infection with low-grade or opportunistic infectious agents (fungi, viruses, Pneumocystis), diarrhea, and a high incidence of malignancy.
Combined Immunodeficiency Disorders
Patients with combined immunodeficiency disorders suffer from antibody deficiency as well as cellular immunodeficiency. Entities in this category include the following:
• Severe combined immunodeficiency disorders, such as reticular dysgenesis and “Swiss-type” immunodeficiency, are due to a defect in relatively primitive hematopoetic precursor cells, resulting in profound deficit of both T cells and B cells. As a result, these infants have a marked susceptibility to infection and rarely live past infancy.
• Partial combined immunodeficiency disorders include ataxia telangiectasia and Wiskott–Aldrich syndrome. Ataxia telangiectasia patients initially present with ataxia during the first year of life and later in childhood suffer from recurrent susceptibility to infection. Telangiectasia of the conjunctiva and the skin may appear anytime from birth to several years of age. Increased susceptibility to sinopulmonary infections begins at about age 3 years. There is a selective IgA deficiency and variable degrees of T-cell deficiency. The cellular immunity becomes increasingly impaired with the passage of time, and patients are susceptible to viral and bacterial infections. Wiskott–Aldrich syndrome involves both cellular and humoral abnormalities. Patients display the triad of eczema, recurrent pyogenic infections, and thrombocytopenia. Children with this disorder commonly die within the first decade of life.
Complement Deficiencies
The complement system plays a critical role in the normal inflammatory reaction. Activation of the complement system (classic pathway or alternative pathway) involves a complex interaction between a variety of proteins. Deficiency of any component in this system can result in increased susceptibility to infection. Some patients with sickle cell anemia or patients who have had splenectomy have an unexplained defect in the alternative pathway of the complement system that may be linked to the susceptibility of these patients to infection by encapsulated organisms.
Phagocytic Dysfunction
The neutrophils, monocytes, and macrophages are the final link in the complex inflammatory reaction. Every event that has taken place during inflammation has occurred with the ultimate goal of bringing these phagocytes into contact with the offending microbial organisms such that these cells may kill the organisms. Again, the process of mobilization, adherence, locomotion, Chemotaxis, phagocytosis, and intracellular killing is complex, and defects can occur at any point during the process from a variety of causes:
• Disorders of production result in a decrease in the number of circulating neutrophils. Neutropenia resulting from toxic depression of the bone marrow due to radiation or chemotherapy is common.
• Defects in adherence may be due to diabetes mellitus, acute alcohol intoxication, corticosteroids, and leukemia.
• Defects in Chemotaxis may be due to complement deficiency, cell-derived agent deficiencies (e.g., lymphokines), or the production of inhibitors such as in patients with Hodgkin’s disease or sarcoidosis. Chediak–Higashi syndrome and diabetes mellitus result in intracellular defects, which decrease Chemotaxis.
• Defects in phagocytosis are most often due to opsonin defects, such as complement or immunoglobulin deficiencies.
• Defects of microbicidal activity involve abnormalities of either oxidative burst or granule function.
• Chronic granulomatous disease is a syndrome characterized by abnormal neutrophil oxidative metabolism, resulting in phagosomes lacking the microbicidal activity of superoxide and anion and hydrogen peroxide.
HEMOSTASIS
Hemostasis may be defined as the process by which the vascular system maintains its integrity as a closed system. Injury to soft tissues and to the endothelium of a blood vessel initiates a complex series of events that ultimately leads to formation of a clot. There are three basic phases in hemostasis: (1) vascular spasm, (2) formation of a platelet plug, and (3) blood coagulation. Vascular spasm occurs immediately after injury and results in decreased blood flow to the site of injury. Platelets play a critically important role in primary hemostasis and in initiating blood coagulation. The intact vascular endothelium is non-reactive to platelets; however, after an injury to the vessel wall, collagen fibrils of the vascular endothelium are exposed and platelets from the circulating blood adhere to the site of injury within seconds. The adherent platelets become activated and undergo the so-called platelet-release reaction. Released platelet factors, among other effects, activate the coagulation factor X to initiate the intrinsic coagulation sequence. Concomitantly, the release of tissue factors from injured cells participates in the activation of the extrinsic coagulation system.
Activation of platelets occurs by stimulation of receptors on the platelet surface by subendothelial collagen (other nonphysiologic surfaces, including glass, will also activate platelets). The discoid-shaped platelet responds by changing its shape to a more spheric form with filamentous cytoplasmic extensions and undergoes a release reaction. The release of storage granules, which contain adenosine diphosphate (ADP), serotonin, thromboxane A2, biogenic amines, and potassium, serves to induce further platelet aggregation, secretion, and vasoconstriction. During the platelet-release reaction, glycoprotein Ib surface receptors on the platelet interact with Von Willebrand factor (vWF) and the exposed collagen, making the platelet “sticky.”
The combination of platelet “stickiness” and the recruitment of additional platelets leads to the formation of an initial clot. The release of ADP modifies the surface of the platelet so that fibrinogen can attach to the heterodimer complex formed by glycoproteins IIb and IIIa on the platelet surface. This linkage of platelets creates a hemostatic plug; however, if not reinforced, the initial clot soon will begin to degenerate.
Secondary hemostasis occurs when internal and external coagulation pathways are initiated. As proteins present on the surface of the platelet come into contact with the damaged endothelial wall, factors VII and XII are activated. The intrinsic pathway is initiated by the activation of factor XII (the Hageman factor), which is converted into a proteolytic enzyme. The Hageman factor also activates the complement system. The cascade of the intrinsic pathway proceeds over a span of several minutes to the development of factor Xa.
The extrinsic pathway is triggered by the introduction of tissue factors containing thromboplastin into the blood. Tissue factor complexes with factor VII to activate it, and the resultant complex interacts with factor X to form factor Xa. Thereafter, with the facilitation of calcium ions and phospholipids, this prothrombin activator complex converts prothrombin to thrombin as the two pathways converge.
Thrombin is a serine protease that splits the fibrinogen molecule. The resulting fibrin molecules associate side by side to form a noncovalently linked fibrin polymer, which is highly susceptible to the proteolytic enzymes present in the plasma and is not sufficiently stable for normal hemostasis. Fibrin stabilization is achieved following activation of factor XIII by thrombin in the presence of calcium. The clot thus formed is significantly more effective in maintaining hemostasis. Incorporated into the clot are factors needed for later clot degeneration (fibrinolysis). The clotting system and the fibrinolytic system exist in a homeostatic balance.
Disorders of Primary Hemostasis (Platelet Dysfunction)
Platelet-associated bleeding disorders and hypercoagulable states can arise from several factors, either quantitative or qualitative. Platelets (thrombocytes) are fragments of megakaryocytes, the large cells in the bone marrow, which descended from pluripotent stem cells. The megakaryocytes shed platelets, which measure 2 to 6μ in diameter and have a life span of 8 to 10 days. A normal platelet count is 150,000 to 400,000/mm3 of blood.
Quantitative Platelet Disorders
Thrombocythemia results from an overproduction of platelets, and it occurs in patients with myeloproliferative disorders, usually as a result of abnormal stem cell reproduction, including polycythemia vera, chronic myelogenous leukemia, and idiopathic refractory sideroblastic anemia. Thrombocytosis is also an entity with overproduction of platelets, but it is not a primary disorder, such as thrombocythemia; rather, it is a reactive disorder secondary to an underlying malignancy or to a nonmalignant disease. Thrombocytosis may be due to production of plasma platelet-stimulating factor in response to a variety of factors, including malignancy, inflammation, and others. Splenectomy also can result in thrombocytosis as the spleen removes platelets from the blood; when the spleen is absent, the platelet count increases and may cause a hypercoagulable state. Both thrombocythemia and thrombocytosis exist when the platelet count is greater than 400,000/mm3 and may present with diffuse thromboses, such as venous thrombosis, pulmonary embolism, transient ischemic attacks, or myocardial infarction. A severe condition exists if the count is greater than 800,000/mm3. Therapy usually is directed at correcting the underlying disorder.
On the other hand, thrombocytopenia is defined as a platelet count of less than 150,000/mm3 and is the most frequent platelet abnormality associated with malignancies. Patients with 40,000 to 60,000 platelets per mm3 have an increased incidence of postsurgical and post-trauma bleeding, whereas patients with fewer than 20,000 platelets/mm3 may have spontaneous bleeding. Thrombocytopenia may be caused by sequestration of platelets by the spleen (hypersplenism). Several drugs, including chemotherapeutic agents, and radiation therapy are common causes of thrombocytopenia in patients with malignancies. In fact, bone marrow suppression is a major dose-limiting effect of chemotherapy. Antimetabolites and antimitotic agents also cause generalized bone marrow hypoplasia or aplasia. Alcohol and estrogens may cause a decreased megakaryocyte production, and hence thrombocytopenia. Aspirin, furosemide, heparin, penicillin, and phenytoin also are associated with a decreased platelet survival time. Multiple blood transfusions also may result in thrombocytopenia. Although stored blood is viable for 21 days, the platelets lose their effectiveness after 24 hours of storage. Neoplasms such as multiple myeloma, lymphoma, leukemia, or any metastatic carcinoma involving the bone marrow displace the megakaryocytes with malignant cells, thus producing thrombocytopenia. Viral and bacterial infections decrease platelet production by releasing endotoxins, resulting in damaged platelets and decreased platelet survival time.
Idiopathic thrombocytopenic purpura (ITP) is a platelet dyscrasia. The mechanism of platelet destruction is unknown but is most likely due to binding of an immunoglobulin G (IgG) autoantibody to circulating platelets causing destruction of the platelets. ITP may be associated with pregnancy, malignant disease, HIV infection, and autoimmune diseases, such as systemic lupus erythematosus (SLE).
Qualitative Platelet Disorders
Several rare hereditary disorders can affect platelet adhesion or aggregation characteristics. Bernard–Soulier syndrome, Glanzmann thombasthenia, and storage pool disease are several such conditions.
An acquired platelet dysfunction may be associated with uremia, liver disease, anemia, autoimmune diseases, multiple myeloma, and myeloproliferative disorders. The mechanism of dysfunction varies but may include: (1) interference with the platelet membrane receptor, (2) inhibition of prostaglandin pathways, and (3) inhibition of platelet phosphodiesterase activity. Aspirin produces an acquired platelet dysfunction by irreversibly inactivating cyclo-oxygenase, an enzyme required for the synthesis of prostaglandins. The platelet abnormalities persist until the defective platelets are replaced by new, unaffected platelets. Nonsteroidal anti-inflammatory agents (NSAIAs) bind with the cyclo-oxygenase, but the defect is reversible, lasting approximately 24 hours.
Disorders of Secondary Hemostasis (Coagulation)
Abnormalities of coagulation can be congenital or acquired. Usually, congenital defects involve only a single factor, whereas acquired defects involve multiple factors.
Congenital disorders should be apparent from a history of bleeding problems and include hemophilia (a deficiency or abnormality of factor VIII procoagulant activity); Christmas disease (an abnormality of factor IX); von Willebrand disease (an abnormality of vWF); congenital deficiencies of factor II, factor V, factor VII, factor X, factor XII, and factor XIII (all rare); and afibrinogenemia.
The most common acquired disorders are associated with liver disease that results in decreased synthesis of vitamin K–dependent factors (II, VII, IX, and X) plus factor V, fibrinogen, and possibly other factors. Acquired disorders of hemostasis also may be secondary to the production of antibodies that bind to coagulation factors. Examples include inhibitors of factor VIII procoagulant activity and factor V. Most common acquired bleeding problems are complications of anticoagulation therapy with warfarin or heparin.
MALIGNANCIES
Central venous catheters are an essential device in the treatment of patients with malignancies; they provide reliable and long-lasting venous access for different purposes. Most of the chemotherapeutic agents and other drugs administered to these patients are toxic and sclerosing to the endothelium and result in thrombosis of peripheral veins. The prolonged contact with the endothelium in small, low-flow veins increases the risk of thrombosis. Peripheral veins are thus quickly depleted.
Central venous catheters allow infusion into high-flow veins such as the superior vena cava (SVC) and into the right atrium (RA); so the agents are diluted rapidly and therefore less likely to damage the endothelium with the resultant thrombosis. Furthermore, multiple blood transfusions require the placement of dependable high-flow catheters. Moreover, the development of new cocktails of agents and the success of BMT have allowed a more aggressive therapy, and an increased number of immunosuppressed and thrombocytopenic patients need reliable central venous accesses. Unfortunately, placement of central venous catheters in these immunocompromised patients is risky. An indwelling catheter breaking the epidermal barrier increases the risk of infection and sepsis. Data from the National Cancer Institute show that the risk of bacteremia in oncology patients who are not neutropenic is increased 40-fold by the sole presence of an indwelling catheter.
Cancer chemotherapy utilizes cytotoxic drugs to arrest or shrink tumor growth. Clinically useful anticancer agents have a greater toxicity for malignant cells than for normal cells (selective toxicity). In part, selective toxicity occurs because of differences between malignant and normal cells, such as the proliferative capacity of the cells, although the differences between normal and malignant tissues may be slight. Many normal tissues have a high proliferative capacity; therefore, bone marrow cells, gastrointestinal epithelium, and hair follicle cells are seriously affected by the toxic effects of anticancer agents.
The toxic effect of chemotherapeutic agents and radiation therapy on the bone marrow creates special problems for central catheter placement:
1. Neutropenia results in an increased risk of catheter-related infection.
2. Catheter infection may be difficult to diagnose because of the inability of immunocompromised host to mount a suppurative response.
3. Decreased bone marrow megakaryocytes result in thrombocytopenia and increased risk of bleeding. Furthermore, a hematoma can further increase the risk of catheter-related infections because blood products are an excellent culture medium.
4. Catheter placement in cancer patients who have hypercoagulability has an increased risk of venous thrombotic complications.
Catheter-Related Infections
Neutropenia from chemotherapy results in a high rate of morbidity attributable to complications of infection. BMT recipients are especially susceptible to fungal and bacterial nosocomial infections during and after “conditioning” therapy, which includes cytotoxic chemotherapeutic agents with or without adjuvant radiation, depending on the primary disease and the type of transplantation to be administered. The neutropenia may last 4 to 58 days until engraftment, when the absolute neutrophil count increased to greater than 500/mm3. In profound neutropenia, the patient remains susceptible to these infections throughout the transplant procedure until a successful engraftment has occurred. After engraftment, infections may be associated with complications of BMT, especially graft versus host disease and immunosuppressive therapy, both of which markedly suppress the immune system.
Neutropenia is the most important risk factor for development of catheter-related infections.1 Neutropenia means an absolute count of fewer than 500/mm3. Severe neutropenia is fewer than 200/mm3. As previously described, the phagocytic action of neutrophils is essential in the complex inflammatory reaction and is the final link in the inflammatory reaction, with the offending microbial organisms being engulfed and destroyed by the neutrophils. The overall reported incidence of catheter-related infections is 0.7 to 3.3 per 1000 catheter days; however, an increased incidence has been reported in patients with neutropenia, especially in those who have undergone bone marrow transplantation.2–12
Morrison and colleagues13 showed that 61% of septic episodes in patients with central venous catheters occurred during the time patients were neutropenic. Fever is the hallmark of infection in these patients. Other signs and symptoms of infection may be notoriously absent in immunocompromised patients. Empiric broad-spectrum antibiotic therapy in febrile neutropenic patients is begun while awaiting the results of cultures. On the other hand, febrile cancer patients who do not have central catheters and adequate neutrophil counts are observed until the clinical examination and laboratory tests are completed because of the low incidence of serious infection in these patients.14–16 Other important considerations in cancer patients include suppression of lymphocyte function, compromise of mucosal barriers, poor nutrition, and altered bacterial colonization resulting from frequent hospitalizations and prolonged antibiotic therapy, even in the absence of neutropenia.
Neutropenia in cancer patients also may be due to bone marrow replacement by tumor cells and decreased production of neutrophils, most commonly seen in hematologic malignancies such as leukemia, lymphoma, myeloma, and other metastatic deposits.
Many infections in neutropenic patients will present as septicemia. It is critical to recognize the clinical signs and symptoms of hematogenous dissemination of infection and the diagnostic value of blood cultures. When one of these patients develops fever, as soon as cultures have been obtained,17 an empiric, wide-spectrum antibiotic therapy such as the combination of mezlocillin, cefazolin, and gentamicin18 must be instituted.
Most catheter-related infections can be controlled without removal of the catheter; however, if the patient’s condition deteriorates and besides fever there is refractory hypotension, septic emboli, or persistent positive blood cultures, the catheter must be removed.19,20 Riikonen and colleagues20 showed that 78% of documented septicemias were curable without the need for removal of the catheter in children who were hematology–oncology patients with neutropenia. Others have reported similar success in the treatment of catheter-related bacteremia with the use of empiric wide-spectrum antibiotic therapy. Catheter removal rates were between 22 and 68% in these studies.12,15,18,21–27
Coagulase-negative staphylococci, once considered a nonpathogenic bacteria, living on the skin and mucous membranes as harmless commensals, have become increasingly significant clinically, causing considerable morbidity and even mortality. These bacteria are the most common cause of nosocomial bacteremia in BMT patients, both before and after the neutropenic period.18,28–30 The worldwide emergence of methacillin-resistant coagulase-negative staphylococci has led to the inclusion of vancomycin in the initial empiric antimicrobial therapy in BMT patients, especially during the periods of febrile neutropenia.18,23 Some are reluctant to include vancomycin because of the risk of development of vancomycin-resistant enterococci.
Patients with Hodgkin’s disease, BMT recipients, and patients receiving remission maintenance therapy for acute lymphocytic leukemia also may have cellular immune dysfunction, which results in a different spectrum of infection than does neutropenia. Neutropenic patients receiving broad-spectrum antibiotics for a prolonged time develop fungal infections (Candida and Aspergillus organisms).
Bleeding Diathesis
Radiation and drug toxicity of the marrow-proliferating pool or marrow replacement by tumor also result in thrombocytopenia. Neoplasms involving the bone marrow displace the mature megakaryocytes with malignant cells. The toxic effects of chemotherapy deplete the radiosensitive stem cells. Bone marrow suppression is a major dose-limiting side effect of chemotherapy and affects the production of leukocytes and platelets most profoundly. Red blood cell production also is affected. Alkylating agents have the most prominent effect on the megakaryocyte line, and they cause severe hypoplasia and aplasia. Platelet counts typically fall 7 to 14 days after chemotherapy is begun and slowly recover 2 to 6 weeks later. Multiple myeloma and Waldenström macroglobulinemia, the monoclonal gamapathies, frequently are associated with bleeding diathesis. The monoclonal immunoglobulins interfere with platelet function, resulting in prolonged bleeding times and abnormal platelet aggregation tests.
Blood products often are given to patients with clotting abnormalities before central venous catheter placement because of the perceived increased risk of bleeding; however, the correction of coagulation abnormalities with transfusions of blood products has not been proven effective. Further, this practice has some disadvantages. An indicated procedure may be delayed while the abnormalities are corrected. Some operators may not place catheters because of the abnormalities of homeostasis, denying the patient needed access. In addition, blood products are costly and scarce. Furthermore, transfusion of blood products has risks of hepatitis, HIV, and cytomegalovirus transmission; fluid overload; and transfusion reactions.
Correcting abnormalities of homeostasis with blood-component transfusions is common; however, there are limited data on the efficacy of this practice. Recent literature suggests that the liberal use of blood products is not warranted and should be reserved only to correct severe hemostatic defects.31 Overall rates of bleeding complications of central venous catheter placement range between 0.0 and 2.87%.31–38 Deloughery and colleagues31 had a significant complication rate (22%) only in patients with severe hemostatic defects, including low platelet count and elevated prothrombin time (PT) and partial thromboplastin time (PTT). Several other studies also showed that severe thrombocytopenia is the only risk factor statistically associated with minor bleeding.31,33
Therefore, we can make the following recommendations after reviewing the most recent literature:
• Mild to moderate hemostatic defects need not be corrected with blood components. The costs and risks to the patients are too significant for the benefits received.
• Severe hemostatic defects should be corrected before catheter placement. Patients with platelet counts lower than 20,000/mm3 should receive transfusion of platelets because thrombocytopenia is the greatest absolute risk for bleeding. Multiple defects (platelet count less than 20,000/mm3, PT more than 29 seconds, PTT more than 64 seconds, uremia) warrant efforts to correct abnormalities. Unless placement of the catheter is urgent, alternatives to blood products, such as vitamin K and protamine, should be considered.
• Most bleeding complications can be controlled with manual pressure; usually, it is necessary to spend considerable time compressing.
• Ultrasound-guided puncture of the veins decreases the risk of inadvertent arterial puncture.
• Experience of the operator placing the catheter is a major factor in bleeding complications.31 Only operators with experience should place catheters in these high-risk patients.
• Bleeding time is not reliable for assessing the risk of perioperative bleeding and should not be used.39
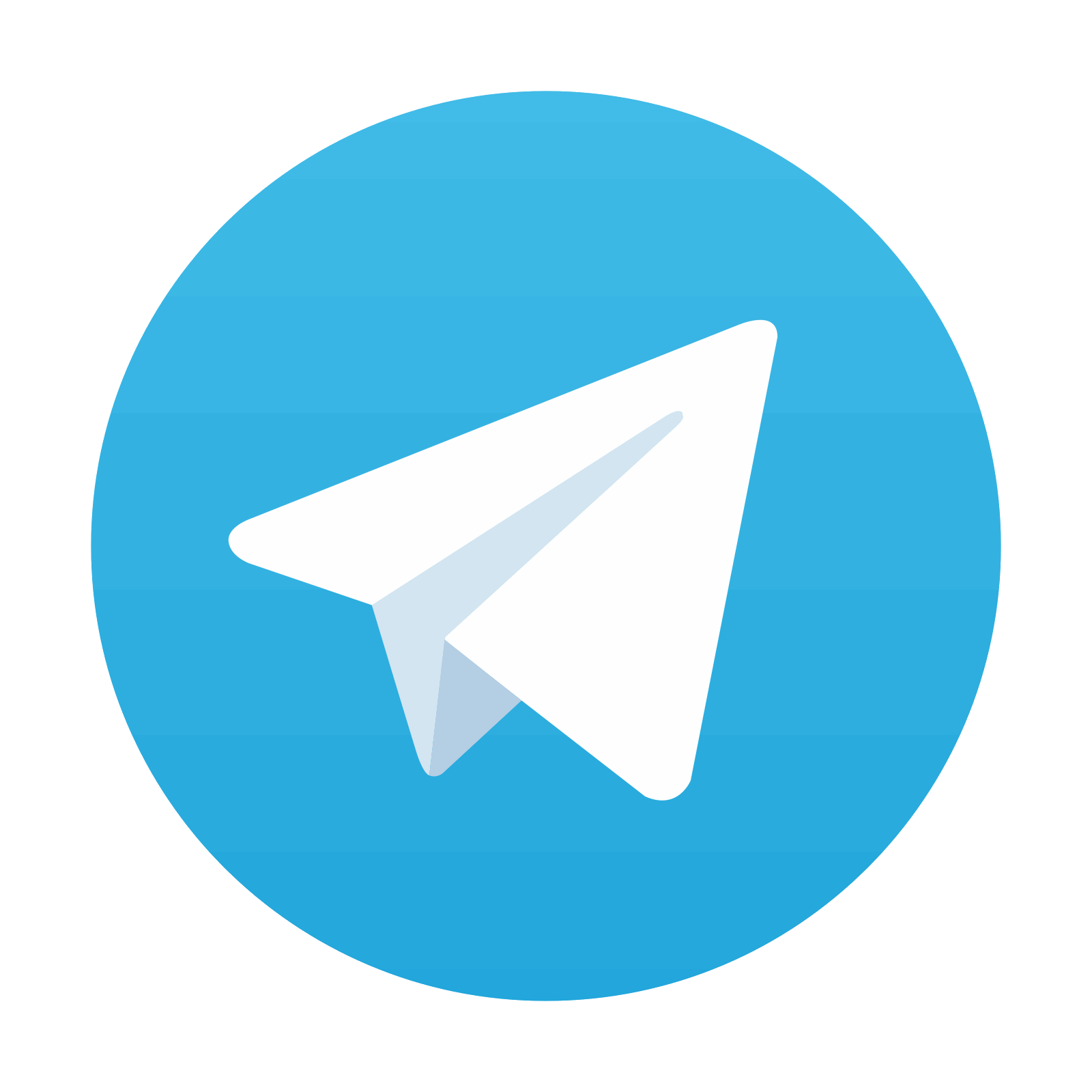