Fig. 7.1
Flow through tubes. (a), laminar flow. (b), transitional flow. (c), turbulent flow. (d), with turbulent flow, a higher pressure is required to maintain the flow rate, and the relationship between driving pressure and flow is non-linear
Airway Anatomy
The upper airway is made up of three sections: the anatomic spaces of the nose, mouth and pharynx; the larynx; and the trachea. The larynx extends from the root of the tongue to the trachea and consists of three parts: the supraglottis, which includes the epiglottis and the false vocal cords; the glottis, which includes the vocal cords and surrounding structures; and lastly the subglottis, a 1.5–2-cm segment below the cords that is completely surrounded by the cricoid cartilage and below which the airway becomes the trachea.
The trachea can be divided into two segments by the thoracic inlet; the shorter cervical trachea extends for the first 2–4 cm from just below the cricoid cartilage to the thoracic inlet. The intrathoracic trachea then continues for a further 7–9 cm until dividing into the right and left main stem bronchi at the main carina. The wall of the trachea contains up to 22 horseshoe-shaped cartilaginous rings that line the anterior and lateral walls and give the tracheal its shape. A membranous component which does not contain any cartilage completes the posterior wall.
The length and diameter of the trachea is roughly proportional to the height of the individual. In the adult male, the trachea is approximately 10–13 cm long with external diameters of 2.3 cm coronally and 1.8 cm sagittally. In women, corresponding tracheal dimensions are 2.0 and 1.4 cm. There are approximately 2 C-shaped rings per cm. The trachea has inherent flexibility and some elasticity; this significantly reduces with age as calcification of the rings occurs. In addition in the elderly as the vertebral height is reduced and kyphosis occurs, the trachea may take on a more horizontal course which can result in more likelihood of extrinsic compression. The cross-sectional horseshoe shape of the trachea can also be markedly altered in the elderly, in particular in those with COPD in whom the distal trachea becomes flattened from side to side, taking on a so-called sabre-sheath shape.
Trachea: Anatomic Relationships
When considering ways in which the trachea can get compressed or obstructed, it is worth reviewing the anatomic relationships of the trachea along its length. Throughout its course the trachea lies in close contact with the oesophagus which runs slightly to the left and behind the airway. Anteriorly, the thyroid isthmus crosses over the superior part of the trachea at the level of the second and third tracheal rings. At the tracheal midpoint, the brachiocephalic artery crosses over from the aortic arch to the right side. On the left lateral side, the aortic arch makes an impression on the left lower tracheal wall. The tracheal bifurcation is at the level of the fifth thoracic vertebra. Mediastinal lymph nodes lie adjacent to the airway wall in paratracheal, pre-tracheal and subcarinal locations.
Lower Airway Anatomy
Below the carina, the airways divide approximately 22–25 times before terminating in alveolar sacs, the functional unit of ventilation. The first 16–18 divisions make up the conducting airway passages of the human adult lung, and the remaining six or seven divisions are considered the respiratory zone composed of respiratory bronchioles from which alveoli bud from the walls. Finally, the airways terminate in the alveolar ducts which are lined with alveoli.
Physiology of Breathing
During inspiration, the thoracic cavity volume increases mainly due to diaphragmatic contraction and flattening and, to a lesser extent, through the action of the external intercostal muscles which raise the ribs, drawing air into the lung and down to the terminal bronchioles. This part of inspiration occurs with large velocity, and the pressure required to move gas along is very small as the airways are uniquely designed to conduct air to the terminal bronchioles. For instance, a flow rate of 1 l/s can be achieved with a pressure drop along the airway of less than 2 cm water. Thus, with the normal swings in intrathoracic pressure during resting ventilation of 3 or 4 cm water (change in intrapleural pressure from +2 cm to−2 cm water), air can move with considerable velocity down to the respiratory zones. Once air arrives there, the cross-sectional area of the acini, or functional respiratory units, is so large (50–100 m2) and the distances so short that there is a rapid fall in velocity of inhaled air and rapid diffusion within the respiratory units occurs.
Accessory muscles of inspiration include the scalene muscles which elevate the first two ribs as well as the sternomastoids which can elevate the sternum to some extent. Associated small muscles that play a minor role include the alae nasi, which cause flaring of the nostrils, and some of the extensor neck muscles, all of which try to increase the diameter of the upper airway passages.
The compliance of the lung reflects the change in volume for unit pressure, and in health, the lungs are naturally very distensible with a compliance of about 200 l/cm water. Lung compliance increases in diseases such as COPD where there is reduction in lung elasticity due to tissue destruction and emphysema. Reduced compliance is associated with conditions that make the lungs stiff, such as pulmonary oedema and pulmonary fibrosis.
Airway Resistance and Flow
Resistance can be defined by the mathematical formula:
2O/L/s. Resistance to airflow into the lungs as they expand is contributed to by intrinsic pulmonary resistance (e.g. elasticity) of the lungs and chest wall but mainly is accounted for by airway resistance, which makes up about 80% of the total resistance. As discussed above, the cross-sectional area of the lower airways is so great, and the branch points so numerous that resistance is almost negligible at the lower levels. Thus, most of the overall airway resistance comes from the upper airways from the level of the trachea to about the 7th generation bronchi. Within these larger airway tubes, resistance to flow is inversely related to the radius of the tube to the 4th power. Therefore, in the presence of fixed obstruction that narrows the airway radius by half, for example, a tracheal obstruction that reduces the lumen to 1 cm, a 16-fold increase in airway resistance results.

Air obeys the principles of fluid dynamics as it moves along the airways from higher- to lower-pressure areas. Flow can occur in three different patterns within the airways: laminar, turbulent and transitional with eddy formation at branch points or when encountering irregularities along the airway surface (Fig. 7.1). Laminar flow is streamlined with air in the centre of the tube moving fastest, and increasing driving pressures will increase flow proportionately. With turbulent flow, a higher pressure is required to maintain the flow rate, and the relationship between driving pressure and flow is non-linear (Fig. 7.1d). In normal conditions in most of the bronchial tree, the airflow is transitional.
Whether flow will be laminar or turbulent also depends to a large extent on the Reynolds number, Re:


Measurement of Airway Obstruction: Pulmonary Function
Airflow is typically measured in the pulmonary function laboratory using the spirometer and through the generation of flow-volume loops. A flow-volume loop is created when the patient inhales deeply to a total lung capacity (TLC) and then forcefully exhales until the lungs have been emptied to the residual volume (RV) followed by rapidly inhalation again to reach the TLC. A typical normal loop is shown in Fig. 7.2a. The upper portion is the expiratory limb and has been well studied and found to contain a wealth of information on airflow obstruction, in particular for small airway disease in COPD. The inspiratory portion gets less attention but as discussed below can be particularly useful in observation of large airway obstruction. It should be noted however that forced inspiratory manoeuvres in particular are effort dependent and that reaching acceptability and repeatability criteria such as they exist for the inspiratory limb of the loop can be difficult. Additionally, most of the studies on the use of flow-volume loops in airway obstruction which are outlined below are observational and limited to small numbers of patients.
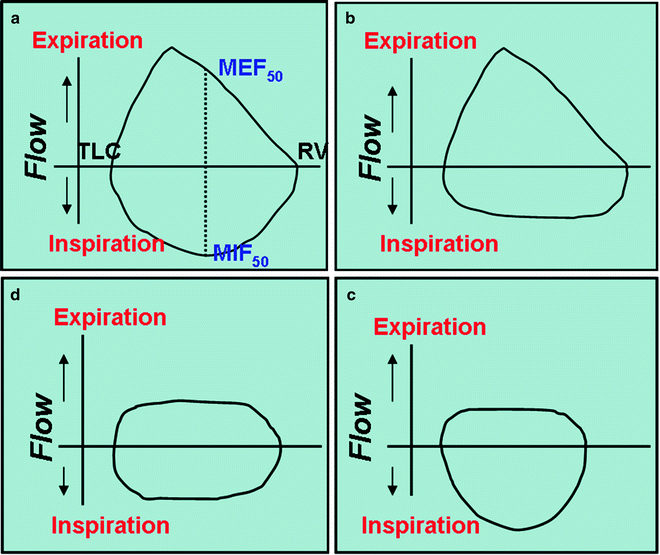
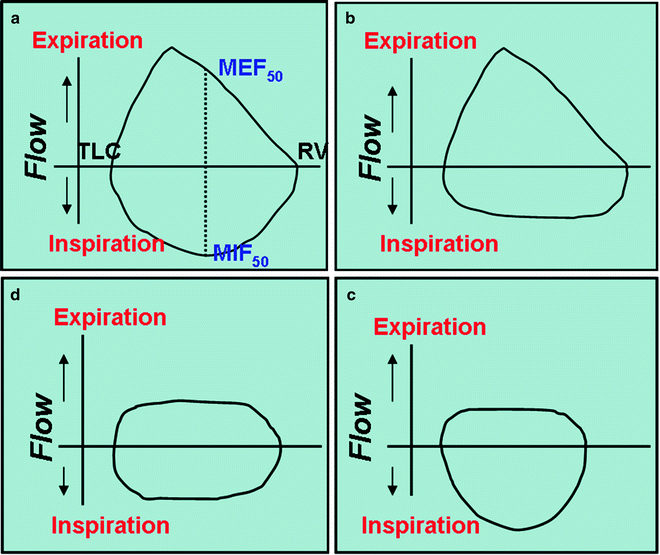
Fig. 7.2
Flow-volume loops. (a), normal. (b), variable extrathoracic obstruction. (c), variable intrathoracic obstruction. (d), fixed airflow obstruction
Flow-Volume Loops
The seminal work on the use of flow-volume loops in airway obstruction was published by Miller and Hyatt in the late 1960s. They proposed a classification based on pressure changes around the site of obstruction and identified three patterns from the contours of the flow-volume loop: (1) variable intrathoracic, (2) variable extrathoracic and (3) fixed obstruction. For these studies, they simulated airway obstruction by having normal subject’s breath through tubes of increasing resistance (smaller diameter) and found that flow rates plateaued during inspiration and expiration and that plateaus were reached at lower flow rates as the resistances were increased Fig. 7.3.
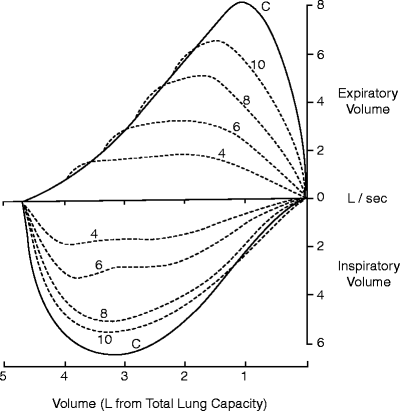
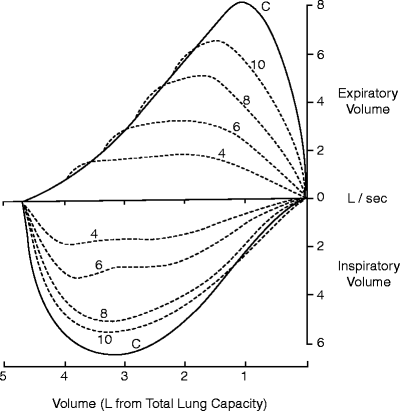
Fig. 7.3
Flow-volume loops obtained from breathing through progressively smaller orifices
During normal respiration, there are changes in transmural pressure along the tracheal length that impact on the calibre of the large airways. The extrathoracic airway is surrounded by positive atmospheric pressure, tending to collapse the airway during inspiration when tracheal pressure is negative. At the same time, the intrathoracic airway is surrounded by pleural pressure which becomes more negative during inspiration helping to keep these airways open. Thus, obstruction within the extrathoracic airway will impact on flow to a greater extent during inspiration (Fig. 7.2b, variable extrathoracic obstruction), whereas obstruction within the intrathoracic airway will affect flow more during expiration when the transmural airway pressure increases and these airways are tending to collapse (Fig. 7.2c, variable intrathoracic obstruction). If the obstruction is stiff or circumferential, these dynamic changes do not impact on the flow as it is limited throughout the cycle and this can be seen as plateauing of both the inspiratory and expiratory limbs of the flow-volume loop (Fig. 7.2d, fixed obstruction). Where there is unilateral main bronchus obstruction, the maximum inspiratory flow tends to be higher at the beginning than towards the end of the forced inspiration because of a delay in gas filling.
Since these original observations by Miller and Hyatt, a number of other criteria based on visual inspection of the flow-volume loop have been proposed as useful although non-specific for the detection of upper airway obstruction. These include biphasic shape and oscillations in the expiratory or inspiratory curves (Table 7.1).
Table 7.1
Proposed criteria for distinguishing upper airway obstruction on pulmonary function tests
Visual criteria reported by | Reported by |
Presence of a plateau | Miller and Hyatt 1969 |
Biphasic shape | |
Presence of oscillations | |
Quantitative | |
Increased PEFR:PIFR | Nairn 1963 |
Increased MEF50 to MIP50 | Jordanoglou and Pride 1968 |
FIF 50% ≤ 100 L/min | Rotman 1975 |
FEF 50%/FIF 50% ≥ 1 | Rotman 1975 |
FIF25-75/FEF25-75 <1 | Owens 1983 |
FEV1/PEFR ≥ 10 ml/l/min – the peak flow is proportionately more reduced by upper airway obstruction than is the FEV1 | Empey 1972; Rotman 1975 |
FEV1/FEV0.5 ≥ 1.5 – the FEV0.5 is proportionately more reduced by upper airway obstruction than the FEV1 | Yernault 1973; Rotman 1975 |
DLCO single breath unchanged | Sackner 1972
![]() Stay updated, free articles. Join our Telegram channel![]() Full access? Get Clinical Tree![]() ![]() ![]() |