Physiological Effects of Pneumothorax and Pleural Effusion
In this chapter, the effects of pleural air or pleural fluid on pleural pressures, pulmonary function and gas exchange, the diaphragm, the heart, and exercise tolerance will be discussed.
EFFECTS OF PNEUMOTHORAX ON PLEURAL PRESSURE
Normally, the pressure in the pleural space is negative with reference to the atmospheric pressure during the entire respiratory cycle. The negative pressure is due to the inherent tendency of the lungs to collapse and of the chest wall to expand. The resting volume of the lung, the functional residual capacity (FRC), is the volume at which the outward pull of the chest wall is equal, but opposite in direction, to the inward pull of the lung with the respiratory muscles relaxed. In Figure. 3.1, the FRC is at 36% of the vital capacity.
The pleural pressure is always less than the alveolar pressure and the atmospheric pressure owing to the elastic recoil of the lung. Therefore, if a communication develops between the pleural space and an alveolus or between the pleural space and the atmosphere, air will flow into the pleural space until a pressure gradient no longer exists or until the communication is sealed. Because the thoracic cavity is below its resting volume and the lung is above its resting volume, with a pneumothorax, the thoracic cavity enlarges and the lung becomes smaller.
When a pneumothorax is present, the pleural pressure increases as it does with the presence of a pleural effusion. However, with a pneumothorax the pressure is the same throughout the entire pleural space if it is not loculated. In contrast, with a pleural effusion there is a gradient in the pleural pressure due to the hydrostatic column of fluid. Accordingly, the pleural pressure with a pleural effusion in the dependent part of the hemithorax is much greater than it is in the superior part of the hemi thorax. The net result is that with a pneumothorax, the upper lobe is affected more than the lower lobe whereas with a pleural effusion the lower lobe is affected more than the upper lobes. The upper lobes are affected more with pneumothorax because the pressure in the apices is normally much more negative than that at the bases. With a pneumothorax the pleural pressures are only slightly negative so there are much greater changes in pleural pressure at the apex of the lung. One way to conceptualize the difference between air and liquid is to understand that with a pneumothorax the lung sinks to the bottom of the hemithorax because it is heavier than air, whereas with a pleural effusion, the lung rises to the top of the hemithorax because it is lighter than the fluid and is floating in the fluid (1).
EFFECTS OF PNEUMOTHORAX ON PULMONARY FUNCTION
When there is a communication between the alveoli and the pleural space or between the ambient air and the pleural space, air will enter the pleural space because the pleural pressure is normally negative. As air enters the pleural space, the pleural pressure gradually increases. Air will continue to enter the pleural space until the pleural pressure becomes zero or the communication is closed.
The influence of a pneumothorax on the volumes of the hemithorax and lung is illustrated in Figure 3.1. In the example, enough air entered the pleural space to increase the pleural pressure from to —2.5 cm
H2O at end expiration. The end-expiratory volume of the lung (point B) decreased from 36% to 11% of the vital capacity, whereas the end-expiratory volume of the hemithorax (point C) increased from 36% to 44% of the vital capacity. The total volume of the pneumothorax is equal to 33% of the vital capacity, of which 25% represents a decrease in lung volume and 8% represents an increase in the volume of the hemithorax. There is essentially no information available on the results of the pulmonary function tests of patients with pneumothorax since they rarely undergo pulmonary function testing while the pneumothorax is present.
H2O at end expiration. The end-expiratory volume of the lung (point B) decreased from 36% to 11% of the vital capacity, whereas the end-expiratory volume of the hemithorax (point C) increased from 36% to 44% of the vital capacity. The total volume of the pneumothorax is equal to 33% of the vital capacity, of which 25% represents a decrease in lung volume and 8% represents an increase in the volume of the hemithorax. There is essentially no information available on the results of the pulmonary function tests of patients with pneumothorax since they rarely undergo pulmonary function testing while the pneumothorax is present.
EFFECTS OF PNEUMOTHORAX ON BLOOD GASES
The main physiologic consequences of a pneumothorax are a decrease in the vital capacity and a decrease in PaO2. In the otherwise healthy individual, the decrease in the vital capacity is well tolerated. If the patient’s lung function is compromised before the pneumothorax, however, the decrease in the vital capacity may lead to respiratory insufficiency with alveolar hypoventilation and respiratory acidosis.
Most patients with a pneumothorax have a reduced PaO2 and an increased alveolar-arterial oxygen difference [P(A—a)O2]. In one series of 12 patients, the PaO2 was below 80 mm Hg in 9 patients (75%) and was below 55 mm Hg in 2 patients (2). In the same series, 10 of the 12 patients (83%) had an increased P(A—a)O2. As one would expect, patients with secondary spontaneous pneumothorax and those with larger pneumothoraces tended to have a greater decrease in the PaO2 (2). In the Veteran’s Administration (VA) cooperative pneumothorax study, blood gases were obtained in 118 patients with spontaneous pneumothorax; the mean PaO2 was below 55 mm Hg in 20 (17%) and below 45 in 5 (4%), and the mean PaCO2 exceeded 50 mm Hg in 19 (16%) and 60 mm Hg in 5 (4%) (3). Of course, the abnormalities in the blood gases may have been due at least in part to the underlying lung disease in this study (3). Similar findings are present in animals with pneumothoraces.
When a pneumothorax was induced in awake, standing dogs by the intrapleural injection of 50 mL/kg N2, the mean PaO2 fell from 86 to 51 mm Hg (4).
When a pneumothorax was induced in awake, standing dogs by the intrapleural injection of 50 mL/kg N2, the mean PaO2 fell from 86 to 51 mm Hg (4).
The reduction in PaO2 appears to be due to both anatomic shunts and areas of low ventilation-perfusion ratios in the partially atelectatic lung. When Norris et al. (2) gave 100% oxygen to their 12 patients, the average anatomic shunt was more than 10%. The larger pneumothoraces were associated with greater shunts (2). Pneumothoraces occupying less than 25% of the hemithorax are not associated with increased shunts.
In the study on dogs conducted by Moran et al. (4), the relative perfusion of the lungs was not altered when pneumothorax was induced, but the ventilation to the ipsilateral lung was reduced, resulting in low ventilation—perfusion ratios on the side with the pneumothorax. Anthonisen (5) reported that lungs with pneumothorax demonstrated uniform airway closure at low lung volumes, and he suggested that airway closure is the chief cause of ventilation maldistribution in spontaneous pneumothorax.
The PaO2 usually improves with treatment of the pneumothorax. In the animal study of Moran et al. (4) in which the mean PaO2 dropped from 86 to 51 mm Hg with the introduction of a pneumothorax, the PaO2 returned to baseline immediately after reexpansion. In humans treated for pneumothorax, the normalization of the PaO2 takes longer. Three patients with an initial anatomic shunt above 20% had a reduction of at least 10% in their shunt 30 to 90 minutes after the removal of intrapleural air, but it still remained above 5% in all patients (2). Three additional patients with anatomic shunts of 10% to 20% had no change in their shunts when the air was removed (2). The delay in improvement in humans as compared with animals may be related to the duration of the pneumothorax.
When a tension pneumothorax is produced in animals spontaneously breathing room air, there is a profound deterioration in the oxygenation status. In one study in goats, the mean PaO2 fell from 85 to 28 mm Hg, whereas in monkeys the PaO2 fell from 90 to 22 mm Hg before the animals became apneic (6). There was a linear reduction in the PaO2 as the volume of pleural air was increased (6). The reduction in the PaO2 appeared to be due to the continued perfusion of the side with the pneumothorax despite decreased ventilation (6). The cardiac output was relatively well preserved in the animals with a tension pneumothorax (6). When the air is evacuated from the pleural space in experimental animals with tension pneumothorax, the oxygenation status returns to normal almost immediately (6).
EFFECTS OF PNEUMOTHORAX ON DIAPHRAGMATIC FUNCTION
To my knowledge there have been no studies evaluating the effects of a pneumothorax on diaphragmatic function. I would anticipate that the presence of a pneumothorax would have less effect on the diaphragmatic function than would a pleural effusion of comparable volume, since the pleural pressure would increase much more with the pleural fluid. The diaphragmatic inversion that is seen relatively frequently with a pleural effusion is not seen with pneumothorax. With a tension pneumothorax, the diaphragm may be displaced inferiorly because of the increased pleural pressure but the functional significance of this displacement is not known.
EFFECTS OF PNEUMOTHORAX ON EXERCISE TOLERANCE
There have been no studies on the effects of a pneumothorax on the exercise tolerance of either animals or man. However, it would be anticipated that the exercise tolerance would be markedly impaired since many patients are dyspneic at rest.
EFFECTS OF PNEUMOTHORAX ON CARDIAC FUNCTION
The presence of a small-to-moderate pneumothorax has very little influence on cardiac function. When Moran et al. (4) introduced 50 mL/kg N2 into the pleural spaces of dogs, the cardiac output was not significantly affected. However, the presence of a tension pneumothorax in an animal can cause a marked reduction in cardiac output. Carvalho et al. (7) produced right-sided tension pneumothoraces with mean pleural pressures of +10 and +125 cm H2O in 10 mechanically ventilated adult sheep. In these animals, the mean cardiac output fell from 3.5 L/minute to approximately 1.2 L/minute and the mean blood pressure fell from 80 mm Hg to less than 40 mm Hg with a pleural pressure of +25 cm H2O.
The development of a tension pneumothorax in humans is also associated with impaired hemodynamics. Beards and Lipman (8) recorded the hemodynamics of three patients who developed a tension pneumothorax while on mechanical ventilation. With the development of the tension pneumothorax, the mean cardiac
outputs that were 7.3, 4.8, and 3.6 L/minute/m2 at baseline fell to 3.0, 3.1, and 1.4 L/minute/m2, respectively. The mean arterial pressures that were 97, 96, and 68 mm Hg fell to 33, 68, and 57 mm Hg, respectively. The probable mechanism for the decreased cardiac output is decreased venous return due to the increased pleural pressures. Moderate increases in the pleural pressure with a pneumothorax in conjunction with thoracoscopy have little influence on the cardiac output. Ohtsuka et al. (9) studied the hemodynamics while the lung was hemicollapsed and CO2 was infused at a pressure of 8 to 10 mm Hg. The mean cardiac index was virtually the same before and after 30 minutes of CO2 infusion (1.98 vs. 1.95 L/minute/m2) (9).
outputs that were 7.3, 4.8, and 3.6 L/minute/m2 at baseline fell to 3.0, 3.1, and 1.4 L/minute/m2, respectively. The mean arterial pressures that were 97, 96, and 68 mm Hg fell to 33, 68, and 57 mm Hg, respectively. The probable mechanism for the decreased cardiac output is decreased venous return due to the increased pleural pressures. Moderate increases in the pleural pressure with a pneumothorax in conjunction with thoracoscopy have little influence on the cardiac output. Ohtsuka et al. (9) studied the hemodynamics while the lung was hemicollapsed and CO2 was infused at a pressure of 8 to 10 mm Hg. The mean cardiac index was virtually the same before and after 30 minutes of CO2 infusion (1.98 vs. 1.95 L/minute/m2) (9).
EFFECTS OF EFFUSION ON THE PLEURAL PRESSURE
When pleural fluid is present, its volume must be compensated for by an increase in the size of the thoracic cavity, a decrease in the size of the lung, a decrease in the size of the heart, or a combination of these changes (1). Since the thoracic cavity, lungs, and heart are all distensible objects, the volume of each is dependent on the pressure inside minus the pressure outside. The presence of pleural fluid increases the pleural pressure. Since the distending pressure of the thoracic wall is the atmospheric pressure minus the pleural pressure, an increase in the pleural pressure will lead to an increase in the distending pressure of the thoracic cavity and an increase in the volume of the thoracic cavity. The distending pressure of the lungs is the alveolar pressure minus the pleural pressure. Therefore, an increase in the pleural pressure will lead to a decreased lung volume. Since the distending pressure of the heart is the intracardiac pressure minus the pleural pressure, an increase in the pleural pressure will lead to a decrease in the size of the heart.
The pleural pressure is normally negative. However, when more than minimal pleural fluid accumulates, the pleural pressure becomes positive. When there is sufficient pleural fluid such that the lung is separated from the chest wall, there is a vertical gradient of 1 cm H2O/cm vertical height due to the weight of the fluid (10). If there is a hydrostatic column 40 cm high in a hemithorax, then the pressure at the bottom of the column would be expected to be approximately 40 cm H2O. When pleural pressures are measured in patients with pleural effusions, the mean pressure is not particularly high. We measured the pleural pressure in 52 patients with significant pleural effusions (median amount of fluid greater than 1,000 mL). Overall, the mean pleural pressure was approximately zero, but there was a wide range in the pleural pressures from —21 to +8 cm H2O (Fig. 3.2) (11). Pleural pressures of —5 cm H2O and less were seen only with a trapped lung or with malignancy. Villena et al. (12) measured the pleural pressure in 61 patients and reported that the initial pleural pressure ranged from —12 to +25 cm H2O. The mean pressure in the patients was approximately +5 cm H2O (12). The probable reason that the pleural pressures were not more positive in the two studies is that the thoracentesis needle was inserted closer to the superior than the inferior aspect of the hydrostatic column produced by the pleural effusion. With a pleural effusion, pleural pressures can at
times be quite positive. Neff and Buchanan (13) reported that the initial pleural pressure was 76 cm H2O in a patient with a pleural effusion secondary to pneumothorax therapy for tuberculosis many years earlier.
times be quite positive. Neff and Buchanan (13) reported that the initial pleural pressure was 76 cm H2O in a patient with a pleural effusion secondary to pneumothorax therapy for tuberculosis many years earlier.
When pleural fluid is removed with thoracentesis, the volume removed is compensated for by an increase in the volume of the lung, an increase in the volume of the heart, and/or a decrease in the volume of the hemithorax. When the volume of these organs changes in this manner, the pleural pressure must decrease. When the pleural pressure is monitored during pleural fluid removal, there is tremendous variability in its changes from patient to patient (11,12).
The elastance of the pleural space has been defined as the change in pleural pressure (cm H2O) divided by the amount of fluid removed (liters) (11). The larger this number the greater the pleural pressure change per unit volume change. In our original series of 52 patients, the pleural space elastance varied from 2 to more than 150 cm H2O/L with a mean elastance of approximately 15 cm H2O/L (11). Patients with trapped lungs due to malignancy or benign disease had pleural space elastances that exceeded 25 cm H2O/L. Villena et al. (12) reported similar values for pleural space elastances. If one looks at the plot of the pleural pressure versus the volume of fluid removed (Fig. 3.3), the elastance tends to be higher during the latter part of the thoracentesis (11,12).
Clinically, it is useful to measure the pleural pressure and calculate the pleural elastance during a thoracentesis. The demonstration that the pleural elastance is greater than 25 cm H2O/L establishes the diagnosis of trapped lung (11,12). Thoracentesis can be continued safely as long as the pleural pressure remains above —20 cm H2O and the patient does not develop chest tightness or pernicious coughing (11,12). Indeed, on several occasions I have removed more than 5,000 mL pleural fluid from patients when the pleural pressure remained above —20 cm H2O and the patients suffered no ill consequences.
Measurements of the pleural space elastance appear to be useful in predicting whether a pleurodesis will be successful (14). The theory is that if the pleural pressure falls rapidly when fluid is removed from the pleural space, then the negative pleural pressure will make it difficult to create a pleurodesis because the two pleural surfaces will be difficult to be kept together (which is necessary to create a pleurodesis). Lan et al.
(14) measured the change in pleural pressure after 500 mL of pleural fluid had been withdrawn in 65 patients with a pleural malignancy. They then inserted a chest tube and continued to drain the pleural space until (a) the drainage was less than 150 mL/day, (b) the drainage was less than 250 mL/day for four consecutive days, or (c) the drainage had continued for 10 days. After one of the three criteria was met, they attempted pleurodesis if the lung had expanded. They reported that the lung did not reexpand (trapped lung) in 11 of the 14 patients who had a pleural elastance greater than 19 cm H2O/L (14). Pleurodesis was attempted in the other three patients with a high pleural elastance and it failed in all three. In contrast, only 3 of 51 patients with pleural elastance less than 19 cm H2O/L had a trapped lung, and pleurodesis was successful in 42 of 43 patients (98%) who returned for evaluation (14).
(14) measured the change in pleural pressure after 500 mL of pleural fluid had been withdrawn in 65 patients with a pleural malignancy. They then inserted a chest tube and continued to drain the pleural space until (a) the drainage was less than 150 mL/day, (b) the drainage was less than 250 mL/day for four consecutive days, or (c) the drainage had continued for 10 days. After one of the three criteria was met, they attempted pleurodesis if the lung had expanded. They reported that the lung did not reexpand (trapped lung) in 11 of the 14 patients who had a pleural elastance greater than 19 cm H2O/L (14). Pleurodesis was attempted in the other three patients with a high pleural elastance and it failed in all three. In contrast, only 3 of 51 patients with pleural elastance less than 19 cm H2O/L had a trapped lung, and pleurodesis was successful in 42 of 43 patients (98%) who returned for evaluation (14).
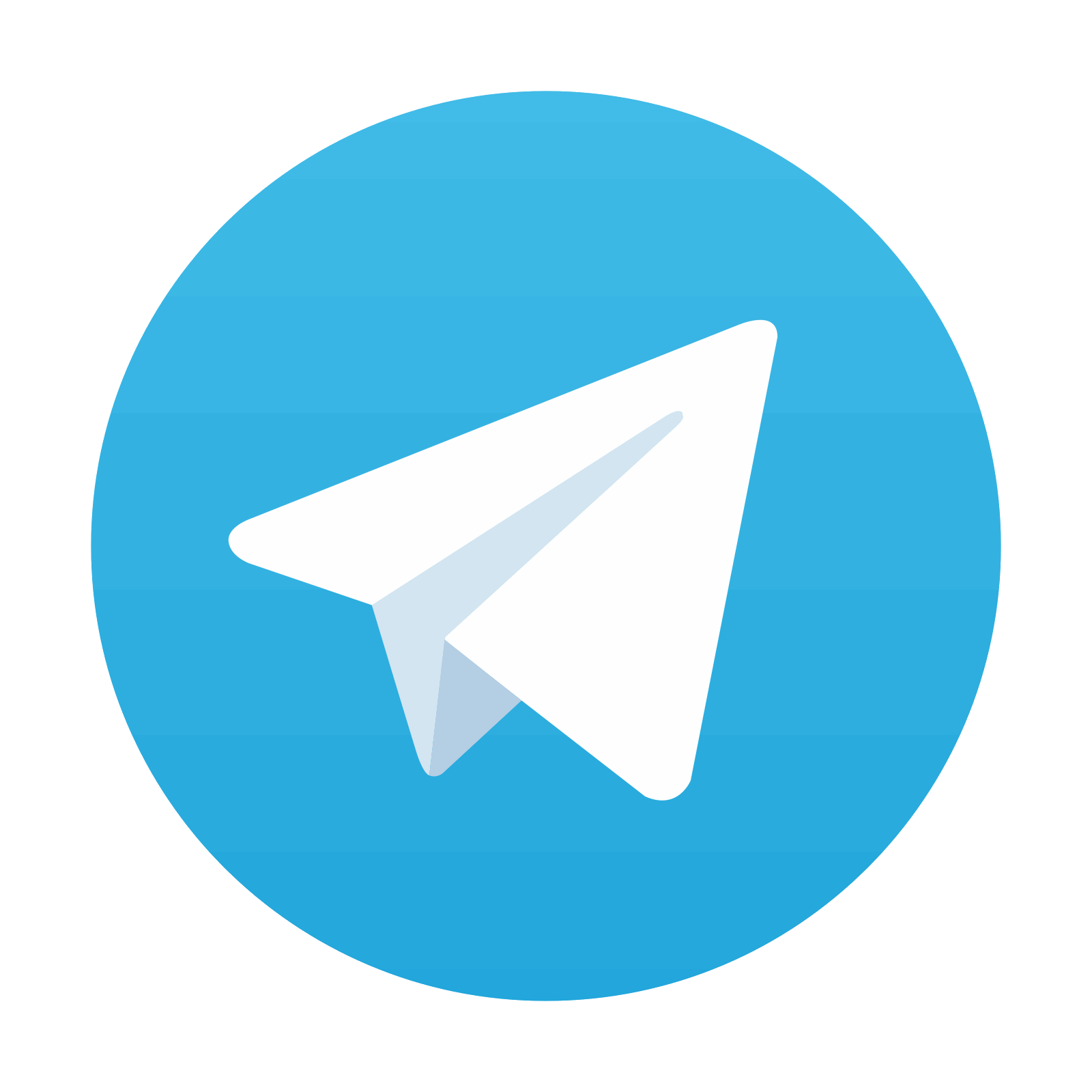
Stay updated, free articles. Join our Telegram channel

Full access? Get Clinical Tree
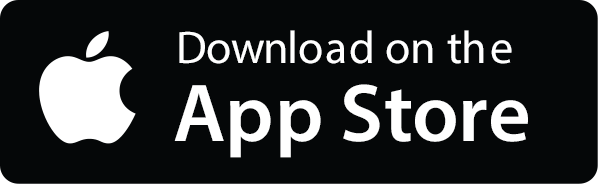
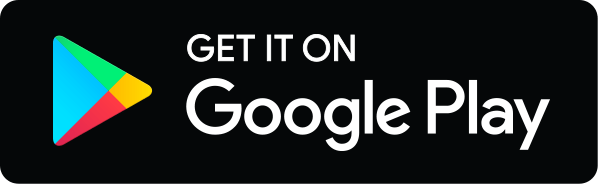