Fig. 8.1
Schematic representation of the impact of Bmal1 and Cry1/Cry2 on the circadian variations in blood pressure in mice. (a) Normal situation, (b) Bmal1 knockout mice, (c) Cry1/Cry2 knockout mice. (a) Normal situation. For explanations see text. (b) Impact of Bmal1 knockout. Note that the circadian rhythm disappears and BP decreases. (c) Impact of dual Cry1/Cry2 knockout. Note that the circadian rhythm disappears and BP increases
Sleep/Activity Cycle and Circadian Variations of Blood Pressure
As mentioned earlier, the sleep/activity cycle is one of the main determinants of the circadian variations of BP in humans. In fact, many factors explain the reduction of BP associated with sleep. This includes not only the reduction in physical activity, but also the change in body position (supine vs. standing), the reduction in mental stress and activity, and a lower activity of neuro-endocrine systems such as the autonomic nervous system. The reduction in physical activity is a well-recognized factor contributing to the decrease in night-time BP [4]. Indeed, physical activity is not only a determinant of BP variability during daytime accounting for 20–60 % of daytime variability, it is also a major factor affecting the reduction of BP during night-time as demonstrated recently using new techniques to evaluate physical activity such as actimeters [31, 32]. Thus, blunted or absent reductions of night-time BP have been reported in subjects physically active at night such as subjects working during the night or doing night shifts [33, 34]. Interestingly, an increased physical activity during daytime has been associated with a greater dipping of BP at night, an effect which has been attributed to a greater reduction in sympathetic tone during the night [31, 35]. Of note, physical activity appears to affect essentially the changes in night-time systolic BP and to a much lesser degree diastolic BP.
Beyond the lack of nocturnal physical activity, the quality of sleep per se has an important impact on the changes in BP measured during the night. In fact, the definition of the dipping of BP at night is highly dependent on the quality of sleep. The apparently poor reproducibility of the dipping pattern has been attributed in part to the impact of BP measuring devices on sleep quality [36]. Thus, when the dipping of BP at night was assessed on several occasions using short-term or long-term repeated ambulatory BP monitoring, about 20–40 % of patients classified as non-dippers actually changed category and became dippers on the second assessment [37–39]. The interference of monitoring devices with sleep is certainly contributing to this poor reproducibility, but many other factors may play a role as will be discussed below.
A lack of physiological decrease in night-time BP is a hallmark of patients with sleep disturbances such as sleep apnea . Normally during sleep, there is an inhibition of cardiovascular sympathetic tone resulting in a reduction in heart rate and BP [40]. In patients with obstructive sleep apnea, oxygen desaturation and carbon dioxide retention cause a peripheral vasoconstriction and a rise in systolic BP at the end of the apnea . When a new respiratory cycle starts with the resolution of the airway obstruction, there is an increase in venous return and cardiac output on a background of peripheral vascular vasoconstriction leading to marked increases in BP [41]. The elevated BP and sympathetic activity with each apneic event contribute to the lack of fall in night-time BP in patients with sleep apnea. Among the other factors that contribute to the development of a high BP at night and consequently hypertension in patients with sleep apnea, one must also cite an elevated muscle tone and micro-arousals [41].
Interestingly, the increased sympathetic nerve activity occurring during the night in patients with obstructive sleep apnea persists during daytime because of the activation of chemoreceptors, a decrease in baroreceptor reflex sensitivity, and endothelial dysfunction [42–46]. In addition to these cardiovascular factors, the stimulation of hormonal systems such as the rennin–angiotensin system, aldosterone, or endothelin appears to participate in the increase in night-time BP observed in patients with sleep apnea syndrome [47]. A summary of the pathophysiological link between obstructive sleep apnea and night-time BP and hypertension is presented in Fig. 8.2.
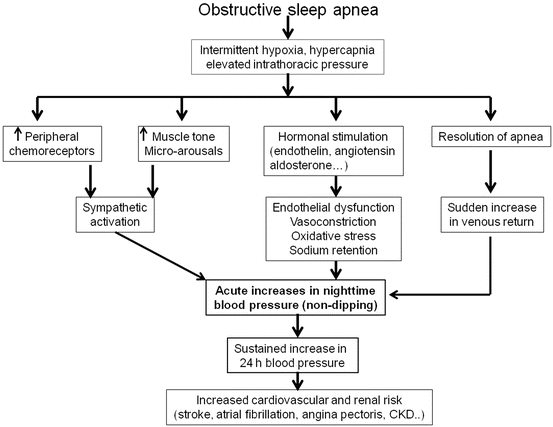
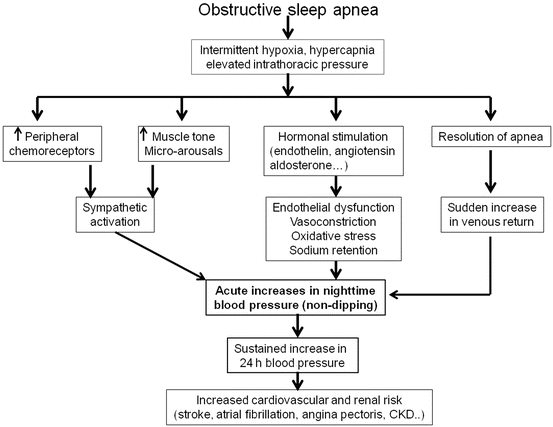
Fig. 8.2
Schematic representation of the pathophysiological link between obstructive sleep apnea and an altered circadian rhythm of blood pressure leading to a high cardiovascular risk
Neuro-hormonal Factors and the Circadian Rhythm of Blood Pressure
Most if not all hormonal systems are characterized by a certain rhythmicity of their secretion during the 24 h of a day. Neuro-hormonal systems have a well-known influence on blood pressure control mediated by direct vascular effects, but also by metabolic and renal effects such as the regulation of renal sodium and water balance. Hence, the day/night profile of neuro-hormonal systems may have an impact on the circadian variations of BP. The diurnal variations in plasma renin activity, aldosterone, angiotensin II, cortisol, ACTH, and melatonin have been well-studied in healthy subjects investigated in standard conditions, but also after sleep deprivation or shifts in sleeping hours. The goal of these studies was not only to characterize the 24 h profile of each of these hormones, but also to assess the precise role of sleep on the 24 h profile variations [48, 49]. Today, it is well established that plasma renin activity and plasma aldosterone and cortisol levels peak in the early morning upon awakening and decrease progressively during the day. Yet the factors contributing to the diurnal variations of these hormones appear to differ between the rennin–angiotensin–aldosterone system and the adrenocorticotrophic system. Indeed, whereas the former is essentially linked to the changes in sleep processes [48], the variations in plasma cortisol and ACTH are independent of sleep and appear to be modulated primarily by a circadian rhythmicity [49]. Other hormonal systems such as endothelin, atrial natriuretic peptide, or brain natriuretic peptide have been investigated as well and were found to follow a certain rhythm with differences between day and night concentrations. However, it seems that the circadian variations of these hormones are more prominent in disease states such as hypertension, chronic kidney disease, or obstructive sleep apnea than in normal healthy subjects [47, 50, 51]. Whether these systems participate in the generation of a circadian rhythm of BP is not clear and it may well be that most of these hormonal systems, with the exception of cortisol, rather follow the sleep/activity cycle of BP than determine a circadian rhythm of BP.
Among all neuro-endocrine systems, the sympathetic nervous system appears to be one of the main determinants of the circadian rhythm of BP. Both plasma epinephrine and norepinephrine follow a diurnal rhythm in humans, but once again it appears that the circadian variations in epinephrine and norepinephrine cannot be explained by a single controlling influence, the variations in norepinephrine being tightly linked to changes in posture and sleep [52], whereas the variations in plasma epinephrine appear to be under the control of a central oscillator [53]. The activity of the sympathetic nervous system increases markedly in the early morning and then fluctuates depending on posture, physical activity, and mental stress. The activity of sympathetic nervous system has been associated with the circadian variations in vascular tone [54] and the early morning rise in sympathetic tone has been related to the early morning BP surge, which is one of the factors implicated in the increased incidence of cardiovascular events (myocardial infarction, sudden death, stroke) observed in the early morning hours of the day [55–57].
Thus, taken together, the data available so far suggest that some of the neuro-hormonal systems such as the sympathetic nervous system or cortisol have a clear circadian rhythm, which appears to be independent of the sleep/activity cycle and may therefore influence directly the circadian rhythm of BP. As far as other hormonal systems are concerned, the variability of their day/night plasma concentrations appears to be a consequence of the circadian variations in sleeping and active periods rather than a cause of the circadian rhythm of blood pressure.
Sodium Balance and the Circadian Rhythm of Blood Pressure
The renal excretion of water, sodium, and other solutes has also been shown to follow a circadian pattern with higher excretion rates during daytime than during night-time [58]. Recently, Firsov et al. have also demonstrated in mice that renal sodium excretion follows a circadian rhythm and that this rhythm is under the influence of circadian clocks [59]. Their data suggest that the circadian clock affects BP, at least in part, by exerting dynamic control over renal sodium handling. The same authors found that many major parameters of kidney function, including tubular reabsorption and secretion, exhibit strong circadian oscillations that might contribute to the circadian variations in BP [60].
One of the first observations relating sodium excretion and the dipping pattern of BP was made by Uzu et al. in a small group of 42 patients with essential hypertension characterized for their salt sensitivity [61]. In this study, the authors first show that the fall in BP during the night is blunted in salt-sensitive patients and that sodium restriction using a low sodium diet can shift the circadian rhythm of blood pressure from a non-dipper to a dipper pattern [61]. In a subgroup of the same patients, the administration of a thiazide diuretic, which induced a negative sodium balance, also reversed the non-dipping pattern of night-time BP [62]. We assessed whether the night-time BP and the dipping are associated with the circadian pattern of sodium excretion in 325 individuals of African descent from 73 families. In each subject, ambulatory BP and daytime and night-time urinary electrolyte excretion were measured simultaneously. In this study, we found indeed that the capacity to excrete sodium during daytime was a significant determinant of nocturnal BP and dipping [63]. Moreover, these data provide some insights on the lack of reproducibility of the dipping pattern in individuals. Indeed, if the night-time dip is influenced by urinary sodium excretion during the day, the decrease in night-time blood pressure will depend on the daytime sodium intake which may vary considerably from day to day. Hence, dietary factors will directly influence the reproducibility of the dipping profile of an individual. Taken together these findings indicated that the ability to excrete sodium during the day is an important determinant of night-time blood pressure as well as of the dipping pattern at least in the normotensive and hypertensive population and particularly among salt-sensitive hypertensive patients.
Later on, the impact of renal function on the night/day ratio of blood pressure and urinary water and electrolyte excretion was investigated. Interestingly, Fukuda et al. found that in patients with renal diseases and a reduced renal function, the lower the glomerular filtration the higher the day/night ratio of blood pressure, urinary sodium excretion, and urinary protein excretion [64]. In this population, the night/day ratio increases mainly in order to eliminate sodium and osmoles rather than water [65]. These data emphasize the importance of glomerular filtration, which affects the capacity to excrete sodium as a determinant of night-time BP. These observations have been summarized in a hypothesis paper of the renal mechanisms of the dipping pattern of BP [66]. According to this hypothesis, the increase in night-time blood pressure represents the classical pressure–natriuresis phenomenon whereby sodium balance is maintained in patients with a limited capacity to excrete salt (Fig. 8.3). In line with this hypothesis, additional observations were made indicating that patients with renal dysfunction require a longer duration until BP falls during the night and that a delayed decrease in night-time BP can be considered as a marker of excessive sodium retention [67, 68] (Fig. 8.4).
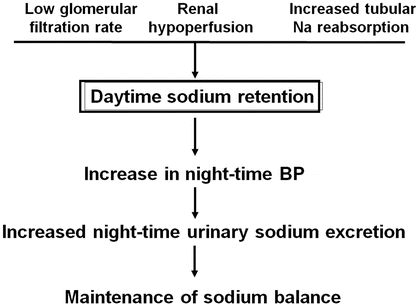
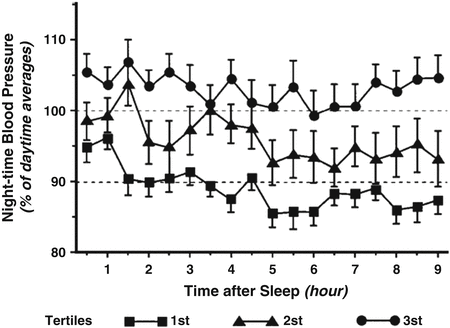
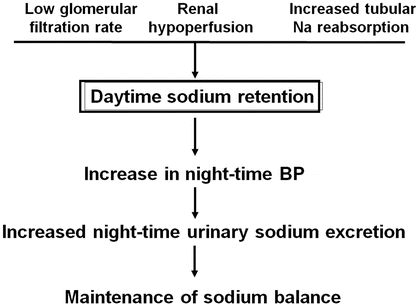
Fig. 8.3
Schematic representation of the effect of a reduced renal capacity to excrete sodium on night-time blood pressure according to the pressure–natriuresis relationship
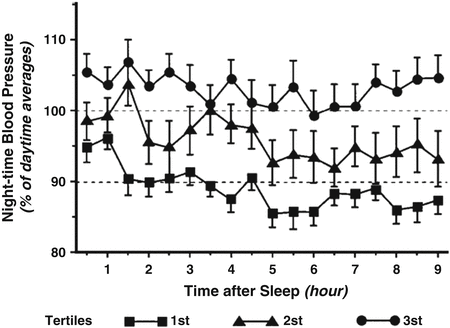
Fig. 8.4
Duration until nocturnal mean arterial pressure begins to fall to <90 % of daytime in patients with chronic kidney disease divided into three tertiles according to creatinine clearance (tertile 1 had the highest clearance and tertile 3 the lowest)—From reference [67])
Yet, whether these renal mechanisms account for the non-dipping pattern frequently observed in an unselected population has not been conclusively demonstrated so far. Nevertheless, renal sodium retention is a clinical characteristic of several disease conditions associated with a blunted or absence of fall in BP during the night. As shown in Table 8.1, a non-dipping profile of 24-h BP has been reported in several clinical conditions. These include secondary forms of hypertension such as malignant hypertension, primary hyperaldosteronism, Cushing syndrome, or pheochromocytoma [69, 70]. A blunted decrease in night-time blood pressure has also been described in patients with a reduced renal function such as patients with diabetic and nondiabetic nephropathies, congestive heart failure, organ transplantation, or elderly patients [69]. At last, drugs like cyclosporin and non-steroidal anti-inflammatory agents have been shown to increase night-time blood pressure and to blunt the nocturnal dip [69]. These various clinical conditions share a common feature: they are all associated with a reduced capacity of excreting sodium either because of a reduction in glomerular filtration rate (primary or secondary) or because of an increased tubular sodium reabsorption as observed in primary hyperaldosteronism or following the administration of drugs such as NSAIDs, COX-2 inhibitors.
Table 8.1
Diseases associated with an increased renal reabsorption of sodium and impaired decrease in blood pressure during the night (non-dipping)
Malignant hypertension |
Obstructive sleep apnea syndrome |
Pheochromocytoma |
Toxemia of pregnancy |
Cushing’s syndrome |
Diabetes (type 1 and 2) |
Chronic kidney disease |
Renal and cardiac transplantation |
Congestive heart failure |
Elderly patients with a reduced glomerular filtration |
Drugs such as non-steroidal anti-inflammatory agents or calcineurin inhibitors |
Overall, these data tend to confirm that sodium intake, and in particular the capacity to excrete salt, is an important determinant of the physiological control of the circadian variations in BP in humans.
Metabolic and Environmental Factors Contributing to the Circadian Variations in Blood Pressure
The impact of sodium intake and excretion on the diurnal variations of BP actually points out the potential roles of the feeding/fast cycle and of the metabolic processes on BP variability [71]. Indeed, depending on the time of food intake, the time-pattern of sodium excretion may vary from day to day or between populations. Moreover, the involvement of gastrointestinal hormones and metabolic factors may vary. The role of the feeding/fasting cycle on BP variability has retained relatively little attention so far. In rats, studies have shown that restricted feeding resulted in an essentially complete loss of coupling of cardiovascular variability to the light cycle. Instead, rats showed increased BP and heart rate and behavioral activity associated with the availability of food [72]. This is not entirely surprising as changes in feeding regimens can profoundly alter the circadian rhythms of body temperature, hormone release, behavioral activity, and peptide levels in the brain [72]. Yet similar observations have not been done in all species and whether the feeding schedule indeed plays a role in variations of BP during day- and night-time remains unclear. Nonetheless, it is clear that several hormonal factors regulating the metabolism such as leptin, adiponectin, insulin, and glucagon follow a circadian rhythm that appears to be independent of plasma glucose concentrations and hence centrally mediated [73, 74]. Whether the circadian production of these metabolic hormones affects BP directly is uncertain. However, disruption of the normal rhythm of some of these hormones has been associated with the development of obesity and metabolic syndrome, which in turn may lead to hypertension and an abnormal dipping of BP during the night.
Interestingly, some specific components of the nutrition may have an impact on BP and its diurnal rhythm. Thus, in a recent cross-sectional study, a high reported caffeine intake was associated with a lower prevalence of hypertension only in nonsmokers [75]. In a subsequent analysis of the same subjects, it appeared that caffeine induced a more pronounced reduction in night-time BP than daytime, thus reinforcing the dipping pattern [76]. It is also worth noting that the benefits of caffeine consumption on BP are probably mediated by the renal properties of caffeine metabolites on adenosine receptors, which induce a diuresis and natriuresis. This observation reemphasizes the role of the kidney in the regulation of the circadian pattern of BP.
The circadian variations of BP blood pressure are also influenced by the environment, which induces short-term as well as long-term fluctuations of BP. Thus beyond the light cycle, BP variability is modulated by the external temperature, the altitude and latitude, and the air quality. Studies focusing on external temperature and weather conditions have clearly shown a seasonality of diurnal BP changes and the complexity of the interactions between weather and BP [77, 78]. A more recent study conducted in 1897 patients referred to a hypertension unit using simultaneously a device that enables to monitor personal-level environmental temperature and a 24-h ambulatory BP monitor has actually reported that temperature not only modulates the seasonal variations of BP, but also the everyday BP rhythm, Thus, the authors found that air temperature measured at personal level negatively affects daytime systolic BP, whereas seasonality mainly affects night-time SBP and morning BP surge [79]. These data indicate that external temperature can modify the variability of BP during the day and the night and thereby modulate the circadian rhythm of BP.
Air pollution has been associated with BP in several studies and positive associations between particulate matter and BP have been reported [80, 81]. Recently, we have analyzed the association of exposure to particulate matter with aerodynamic diameters <10 μm (PM10) on the day of examination and ≤7 days before with ambulatory blood pressure and with sodium excretion in 359 adults from the general population using multiple linear regression [82]. We found that short-term exposure to PM10 was significantly associated with higher night-time SBP, DBP, and a blunted nocturnal SBP dipping in adults from the general population. The mechanism whereby air pollution increases BP appears to be linked to an increase in renal sodium reabsorption by the proximal, and interestingly, the sodium reabsorption precedes the increase in night-time BP. Unfortunately, this study investigated only the short-term effects of air pollution on BP and the real impact on a long-term exposure to air pollution remains to be investigated. In any case, air pollution has been clearly associated with an increased risk of cardiovascular events and reduction in air pollution may be associated with a reduced incidence of complications associated with hypertension.
Conclusions
The physiological control of the circadian variations of BP is very complex and involved many apparently redundant systems. Yet experimental studies on the clock control systems have revealed that the day/night variations in BP are very well preserved and maintained throughout species. The recognition of the various factors that can modulate or blunt the circadian rhythm of BP, and in particular abolish the night-time fall in BP leading to a non-dipping pattern, is of high clinical relevance. Indeed, some of these factors can be corrected, for example, by treating sleep apnea or by reducing salt intake leading to a recovery of the normal circadian rhythm of BP. Moreover, a great interest for chronotherapy has emerged in recent years. This therapeutic approach emphasizes the need to maintain or restore a normal day/night rhythm of BP by prescribing drugs not only in the morning, but also in the evening. At last, the first evidence suggesting that patients with normal circadian variations in BP may develop less cardiovascular and renal complications may be strong incentive to pay more attention to the physiological factors involved in the circadian variability of BP.
References
1.
Gachon F, Nagoshi E, Brown SA, Ripperger J, Schibler U. The mammalian circadian timing system: from gene expression to physiology. Chromosoma. 2004;113(3):103–12.CrossRefPubMed
< div class='tao-gold-member'>
Only gold members can continue reading. Log In or Register a > to continue
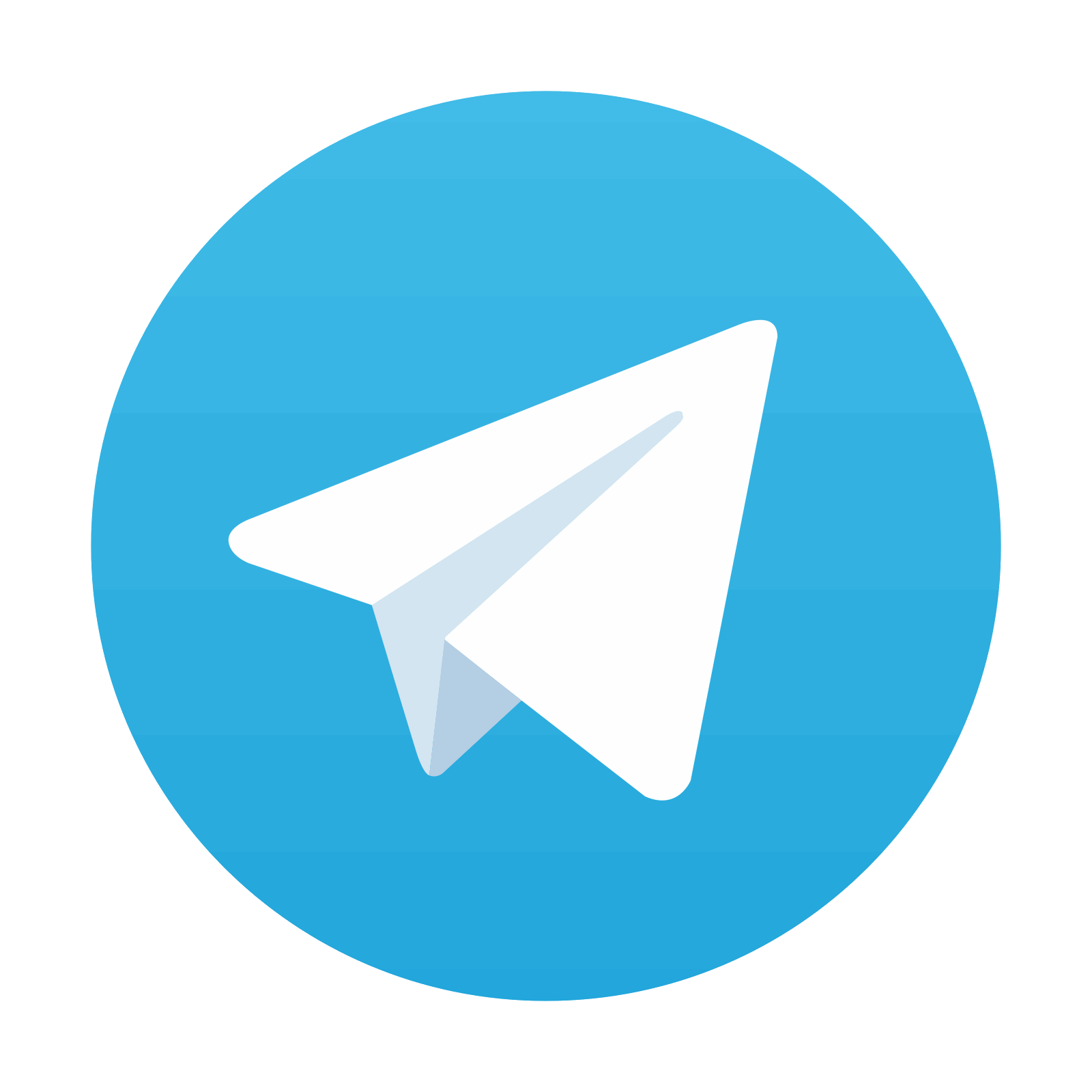
Stay updated, free articles. Join our Telegram channel

Full access? Get Clinical Tree
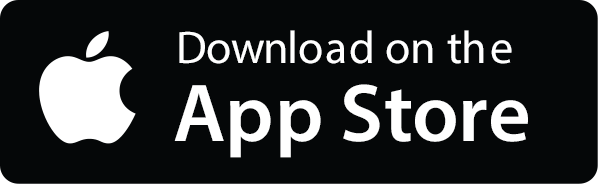
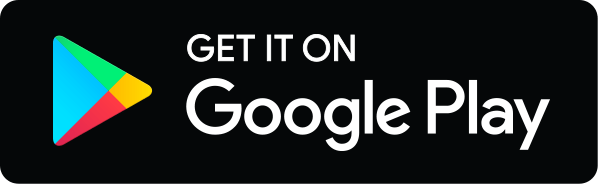