Physics: Fundamentals of Ultrasound Imaging and Instrumentation
Victor Mor-Avi
Rajesh Jaganath
Lynn Weinert
Jim D. Thomas
1. Sound waves cannot travel through one of the following:
A. Water.
B. Air.
C. Metal.
D. Vacuum.
View Answer
1. Answer: D. Sound waves cannot travel in a vacuum, as pressure waves can be transmitted only through physical media consisting of molecules that interact with each other. Water, air, and metal are all such media and therefore sound waves can and indeed do travel in them.
2. Ultrasound is a pressure wave with a frequency above the range of human hearing, which is:
A. 200 Hz.
B. 2 kHz.
C. 20,000 Hz.
D. 200 kHz.
View Answer
2. Answer: C. The upper limit of the range of human hearing is 20,000 Hz or 20 kHz. There are animals that can hear sounds in different ranges than humans. For example, bats’ hearing includes sounds in a much higher frequency range. This is known as supersonic hearing. They produce sound waves, which then echo back to them by bouncing off objects so that they know how far something is, just like a sonar on a submarine.
3. The frequency of a sound wave is measured in hertz as the:
A. Inverse of the wavelength.
B. Maximal amplitude of particle vibration.
C. Number of times particles vibrate each second in the direction perpendicular to wave propagation.
D. Number of times particles vibrate each second in the direction of wave propagation.
View Answer
3. Answer: D. Frequency, in general, is measured in Hz (abbreviation for hertz), which is defined as 1/s. The frequency of a wave is defined as the number of times a particle in a conducting medium vibrates per unit time. Thus frequency is the inverse of the period. Since sound waves are pressure disturbance traveling in the medium in the direction of the particle vibrations, they are called longitudinal waves. In other words, sound waves are vibrations in the direction of wave propagation, and therefore this is the correct answer.
4. Ultrasound imaging is usually performed using frequencies in the range of:
A. 1-30 kHz.
B. Below 5 MHz.
C. Above 0.5 MHz.
D. 1-30 MHz.
View Answer
4. Answer: D. Ultrasound imaging is usually performed using frequencies in the range of 1-30 MHz (Fig. 1-15). The lower frequencies in this range are used to image large organs or deeper structures that require significant penetration depth, while the higher frequencies are used for smaller and more superficial structures that require less depth but better spatial resolution.
5. Assuming that sound velocity in muscle tissue is 1,600 m/s, the wavelength of a sound wave with the frequency of 1.6 MHz is:
A. 1 mm.
B. 1 cm.
C. 1 m.
D. 0.1 mm.
View Answer

5. Answer: A. Wavelength, λ, is defined as the distance a wave travels during a single cycle. Wavelength can be calculated as the product of velocity, c, and the period, T, or alternatively the ratio of velocity and frequency, f:

6. As an ultrasound wave travels through the human body, the type of tissue that results in the fastest loss of its strength is:
A. Fat.
B. Bone.
C. Lung.
D. Blood.
View Answer
6. Answer: C. Because of the high content of air and the abundance of highly reflective tissue/air interfaces, the sound waves dissipate in the lung so fast that the lungs are virtually opaque to ultrasound.
7. The main goal of the gel used during ultrasound imaging is to:
A. Disinfect the transducer.
B. Cool the transducer.
C. To numb the skin and thus reduce patient’s discomfort caused by pressure.
D. To improve the contact between transducer surface and the skin.
View Answer
7. Answer: D. The main goal of the coupling gel is to improve the contact between transducer surface and the skin by eliminating any tissue/air interfaces, which are highly reflective and thus prevent ultrasound transmission into the body.
8. Materials that respond to acoustic waves by generating electric signals and vice versa are known as:
A. Doppler crystals.
B. Acoustic coupling gels.
C. Piezoelectric crystals.
D. Chronotropic agents.
View Answer
8. Answer: C. Piezoelectric crystals are materials that respond to electric signals by vibrating and generating acoustic waves and, vice versa, respond to acoustic waves by generating electric signals. These materials are the basis for medical ultrasound imaging, which relies on transmitting waves by “exciting” the crystals in the transducer by an electrical stimulus, and then receiving the ultrasound waves reflected by structures inside the body, and translating them back into electrical signals that are used to form an image of the reflecting structures.
9. Doppler effect refers to:
A. Change in strength of a sound wave reflected by a moving target.
B. Change in frequency of a sound wave reflected by a moving target.
C. Change in shape of a sound wave reflected by a moving target.
D. Loss of ultrasound energy as a result of wave dissipation by flow.
View Answer
9. Answer: B. Doppler effect refers to change in the frequency of a sound wave reflected by a moving target. We are all familiar with the Doppler effect from our daily life: sounds coming from a moving object have higher pitch when the object approaches us than when the same object moves away from us. This is how we can tell if a train is approaching the station or leaving before we can actually see it (Fig. 1-16).
10. Doppler angle is the angle between:
A. The flow and the long axis of the left ventricle.
B. The ultrasound beam and the long axis of the left ventricle.
C. The flow and the transmitted ultrasound beam.
D. The flow and the central axis of the transducer.
View Answer
10. Answer: C. Doppler angle is the angle between the direction of flow (in Fig. 1-17, flow through the tricuspid valve indicated by the blue arrow) and that of the ultrasound beam (green line). The orientation of either the ventricle (long axis indicated by the pink line) or the transducer (central axis indicated by the brown line) has no role in the interaction between ultrasound and moving blood cells that reflect ultrasound at a frequency that depends on the direction of blood flow along the beam.
Like the train, blood cells moving away from the transducer reflect sound with lower frequency than those moving toward the transducer. What determines whether the former or the latter is the case is the angle between the flow and the direction of the transmitted beam: when the angle is <90 degrees, then the flow is away from the transducer, and vice versa, when the angle is >90 degrees, then the flow is toward the transducer.
11. A positive Doppler shift indicates that the reflector is moving:
A. Faster than the sound wave propagates.
B. Directly toward the transducer.
C. Directly away from the transducer.
D. So that the angle between the direction of the beam and the direction of motion is >90 degrees.
View Answer
11. Answer: D. A positive Doppler shift indicates that the reflector is moving so that the angle between the transmitted beam and the direction of flow is >90 degrees; that is, the reflectors are getting closer, but not necessarily moving directly toward the transducer.
12. A Doppler shift of zero indicates that the reflector is stationary or:
A. Moving in a direction perpendicular to the beam.
B. Moving in a direction parallel to the beam.
C. Moving in a direction perpendicular to the central axis of the transducer.
D. Moving too fast to register.
View Answer
12. Answer: A. A Doppler shift of zero indicates that the reflector is stationary or moving in a direction perpendicular to the beam. Importantly, when the Doppler angle is 90 degrees, the flow is neither toward nor away from the transducer, it is perpendicular to the beam and thus will produce no Doppler shift or, in other words, will reflect ultrasound at the same frequency that was transmitted.
13. Time gain compensation is part of the ultrasound image formation aimed at correcting intensity for variations in the extent to which different media result in ultrasound ____.
A. scattering
B. absorption
C. reflection
D. attenuation
View Answer
13. Answer: D. The combined result of ultrasound scattering, absorption, and reflection is attenuation. Time gain compensation aims at providing a correction for the loss of intensity (or attenuation) by all these different mechanisms. This is done assuming that attenuation in different types of tissue in the heart is the same, which is a reasonably accurate assumption. However, it may become quite inaccurate when there are materials with drastically different acoustic properties such as contrast agents that cause much stronger attenuation. This is the reason why acoustic shadowing artifacts are frequently seen distal to contrast filled blood pools, such as ventricles or atria.
14. The strength of the transmitted ultrasound wave is controlled by adjusting the:
A. Time gain compensation controls.
B. Compression control.
C. Power control.
D. Overall gain control.
View Answer
14. Answer: C. The strength of the transmitted ultrasound wave is controlled by adjusting the power control. Gain control determines to what extent the received signal is amplified, and the compression determines the dynamic range of received signals that are used to create the image. Time gain compensation has nothing to do with the strength of the transmitted power: It is part of postprocessing of the reflections designed to correct for beam attenuation as it travels through the body.
15. The spatial resolution of an ultrasound image is defined as the:
A. Smallest distance between two objects that allows distinction between them.
B. Size of the smallest object that can be clearly visualized in its entirety.
C. Smallest cluster of pixels that can define a single object.
D. Smallest difference in the size of an object that can be visually detected.
View Answer
15. Answer: A. The spatial resolution of an ultrasound image is defined as the smallest distance between two objects that allows distinction between them. This is the definition of spatial resolution. Understandably, the spatial resolution also determines the size of the smallest object that can be visualized, but the change in the size of an object is certainly not the definition of resolution.
16. The spatial resolution of an ultrasound image is equal to the:
A. Gap between two adjacent pixels.
B. Twice the wavelength.
C. Size of a pixel in the relevant direction.
D. One-half of the wavelength.
View Answer
16. Answer: C. While spatial resolution along the ultrasound beam is directly related to wavelength, it is affected by other factors in other directions. However, it can be easily determined by the size of a pixel in the relevant direction, if that is known. The gap between two adjacent pixels is a nonsensical answer designed to confuse you, since there is no gap between adjacent pixels.
17. The temporal resolution of a sequence of ultrasound images is defined by the:
A. Shortest duration of an event that can be detected with confidence.
B. Shortest time in which image information can change completely.
C. Shortest time between two events that allows distinction between them.
D. Shortest time in which pixel values can change.
View Answer
17. Answer: C. Similar to spatial resolution, temporal resolution of a sequence of ultrasound images is defined by the shortest time between two events that allows distinction between them. Similarly, temporal resolution determines the shortest duration of an event that can be detected, but “with confidence” is a subjective term that makes answer (A) incorrect. Answers (B) and (D) are nonsense.
18. The following phrase is meaningless:
A. Spatial resolution.
B. Temporal resolution.
C. Frequency resolution.
D. Contrast resolution.
View Answer
18. Answer: C. The meaningless phrase is “frequency resolution,” while the other three phrases are legitimate, frequently used metrics of image quality that tell us the following (see Table 1-1):
Table 1-1. Types of Image Resolution | ||||||
---|---|---|---|---|---|---|
|
19. The temporal resolution of a sequence of ultrasound images is equal to the:
A. Inverse of transducer frequency.
B. Inverse of frame rate.
C. One cycle of the ultrasound wave.
D. Inverse of the number of frames in the sequence.
View Answer
19. Answer: B. The temporal resolution of a sequence of ultrasound images is equal to the inverse of frame rate. For example, a rate of 20 frames/second corresponds to 1/20 second = 0.05 seconds = 50 ms. The inverse of the transducer frequency is a period (duration of a single cycle) of the ultrasound wave and is in the order of magnitude of microseconds. The temporal resolution of a sequence of images is nowhere near this: it is hundreds of thousands of times longer. Answer (D) is nonsense, as one can create a sequence of any number of frames, which has nothing to do with temporal resolution. Please note that a higher frame rate means that the minimum time between two events that the images can depict (see the previous answer) is shorter. In this regard, the definition of resolution is a bit deceiving (and potentially confusing), because smaller numbers mean better resolution, contrary to our intuition, which may lead us to think that bigger numbers should mean higher resolution. This is the case not only with temporal resolution (in this question), but resolution in general: it is better the smaller the number is. For instance, spatial resolution of 0.1 mm is better (or higher) than 10 mm. Similarly, for temporal resolution, 0.1 second is better (or higher) than 10 seconds.
20. The dynamic range of echoes displayed on the screen is adjusted by the:
A. Time gain compensation controls.
B. Compression control.
C. Transmit power control.
D. Overall gain control.
View Answer
20. Answer: B. The dynamic range of echoes displayed on the screen is adjusted by the compression control. This control can be used to include or suppress weak echoes.
21. As the frequency of ultrasound increases, the maximum imaging depth in the human body:
A. Increases.
B. Decreases.
C. Remains unchanged.
D. May increase or decrease depending on the mechanical index used.
View Answer
21. Answer: B. Sound waves of higher frequencies dissipate in conducting media faster than those with lower frequencies, because of a variety of mechanisms. Thus, of two sound waves transmitted with identical intensities but at different frequencies, the intensity of the wave with the higher frequency that reaches a certain depth is smaller than that of the wave with the lower frequency. In other words, increased frequency translates into smaller imaging depth.
22. Increasing which of the following can best compensate for image losses due to attenuation?
A. Dynamic range.
B. Gain.
C. Frame rate.
D. Depth.
View Answer
22. Answer: B. Attenuation is the loss of signal that can occur because of factors such as absorption with increasing depth and tissue density. Increasing the gain amplifies the return signal. The increase in amplification can come at the expense of greater noise.
23. Decreasing which of the following increases the contrast of the image?
A. Dynamic range.
B. Gain.
C. Power.
D. Baseline shift.
View Answer
23. Answer: A. Dynamic range, also called compression, describes the difference between the highest and lowest amplitude signals received. The differences in signals are fit into a gray-scale range that is then displayed. Increasing the dynamic range results in more shades of gray, which, for instance, may be helpful in differentiating endocardial borders. At lower dynamic range, a higher black-white contrast is produced.
24. The energy of the transmitted ultrasound wave can be changed by adjusting which of the following?
A. Gain.
B. Dynamic range.
C. Pulse repetition frequency.
D. Power.
View Answer
24. Answer: D. Increasing the power increases the energy delivered to the tissues. Visually, this can create similar changes compared to adjusting the gain, which increases the amplitude of the signal returning to the machine. However, unlike gain, increasing the power can result in more heat being transmitted to tissue.
25. Filtering eliminates “ghosting” artifact by removing which of the following?
A. Low-velocity signals.
B. Acoustic shadowing.
C. Mirroring.
D. Aliasing.
View Answer
25. Answer: A. When imaging higher-velocity regions, movement of cardiac structures produces low-velocity signals that appear on the screen as color and may make it harder to interpret the area of interest. Filtering can remove this artifact, called “ghosting,” from the screen.
26. With time gain compensation, the machine is generally preset to perform which of the following actions?
A. Decrease signal in near field, increase signal in far field.
B. Decrease signal in near field, decrease signal in far field.
C. Increase signal in near field, increase signal in far field.
D. Increase signal in near field, decrease signal in far field.
View Answer
26. Answer: A. With increasing depth, a signal becomes increasingly attenuated. Time gain compensation accounts for this signal loss by effectively increasing gain in parallel with an increase in depth. Many modern systems automatically account for this and so the knobs should be left in the neutral position to start and then adjusted.
27. To better assess rapidly moving structures, a sonographer should perform which of the following actions?
A. Narrow scan sector and decrease imaging depth.
B. Narrow scan sector and increase imaging depth.
C. Widen scan sector and decrease imaging depth.
D. Widen scan sector and increase imaging depth.
View Answer
27. Answer: A. With moving structures, a higher frame rate is desired to improve temporal resolution. Some machines allow for a direct increase in frame rate while with others this can be achieved by narrowing the scan sector and decreasing the imaging depth.
28. To reduce aliasing on color-flow Doppler, a sonographer should perform which of the following actions?
A. Reduce the angle between the ultrasound beam and direction of flow.
B. Reduce the color scale.
C. Baseline shift away from the direction of flow.
D. Increase the depth of the image.
View Answer
28. Answer: C. Aliasing occurs when the Doppler shift of high-velocity flow exceeds the Nyquist limit (1/2 the pulse repetition frequency). Changing the baseline shifts the display scale to allow velocities to be displayed up to twice the original Nyquist limit. Increasing the angle between ultrasound beam and direction of flow can also reduce aliasing though at the expense of velocity underestimation. Imaging at an increased depth will decrease the pulse repetition frequency and increase aliasing. In addition, increasing the color scale can also reduce aliasing if it had previously been set lower than dictated by the imaging depth.
29. If a rapidly moving structure such as a cardiac valve appears to be moving in slow motion, which one of the following may be set too high?
A. Frame rate.
B. Sector size.
C. Nyquist limit.
D. Persistence.
View Answer
29. Answer: D. Images can be averaged together to create a smoothing effect by increasing persistence or they can be left unadjusted. Lower persistence maintains temporal resolution and can keep a structure from appearing as though it were moving in slow motion.
30. Image resolution for a region of interest can be improved by which of the following?
A. Increasing the read zoom.
B. Increasing the write zoom.
C. Increasing the sector width.
D. Increasing the dynamic range.
View Answer
30. Answer: B. Increasing the write zoom improves image resolution by increasing both line density and the number of pixels in a given area. Read zoom, on the other hand, only magnifies the image without a change in resolution. Reducing the sector width while maintaining a fixed number of scan lines will also improve spatial resolution. Changing the dynamic range impacts how gray scale is presented on the display but not image resolution. Image resolution can also be improved for a given region by changing the focal point on the display.
31. Increasing scan line density with a fixed sector width results in which of the following?
A. Increase in frame rate.
B. Increase in zoom.
C. Increase in temporal resolution.
D. Increase in spatial resolution.
View Answer
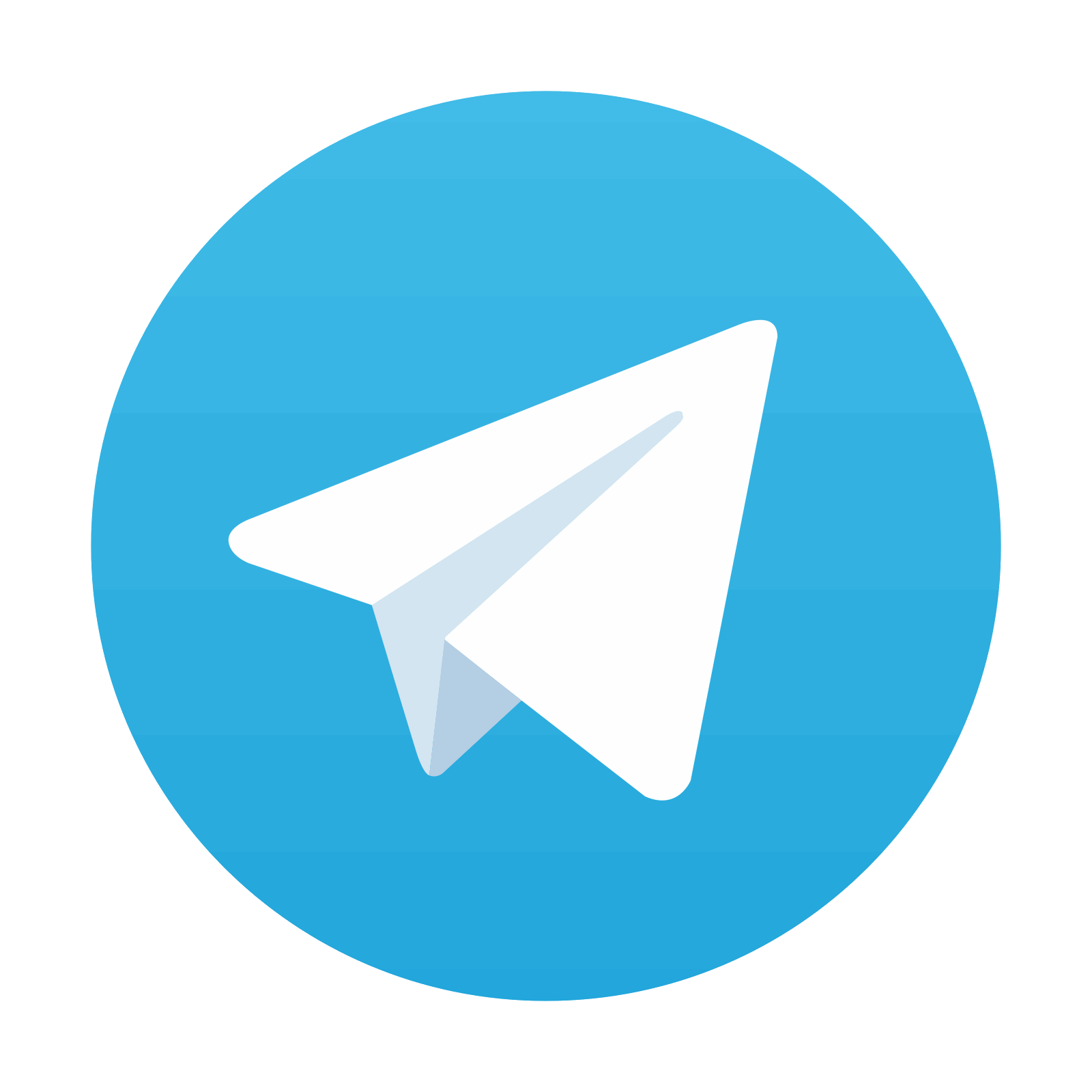
31. Answer: D. Increasing line density improves spatial resolution but at the cost of a decrease in frame rate and temporal resolution. In addition, the number of scanning lines is increased within a given sector.
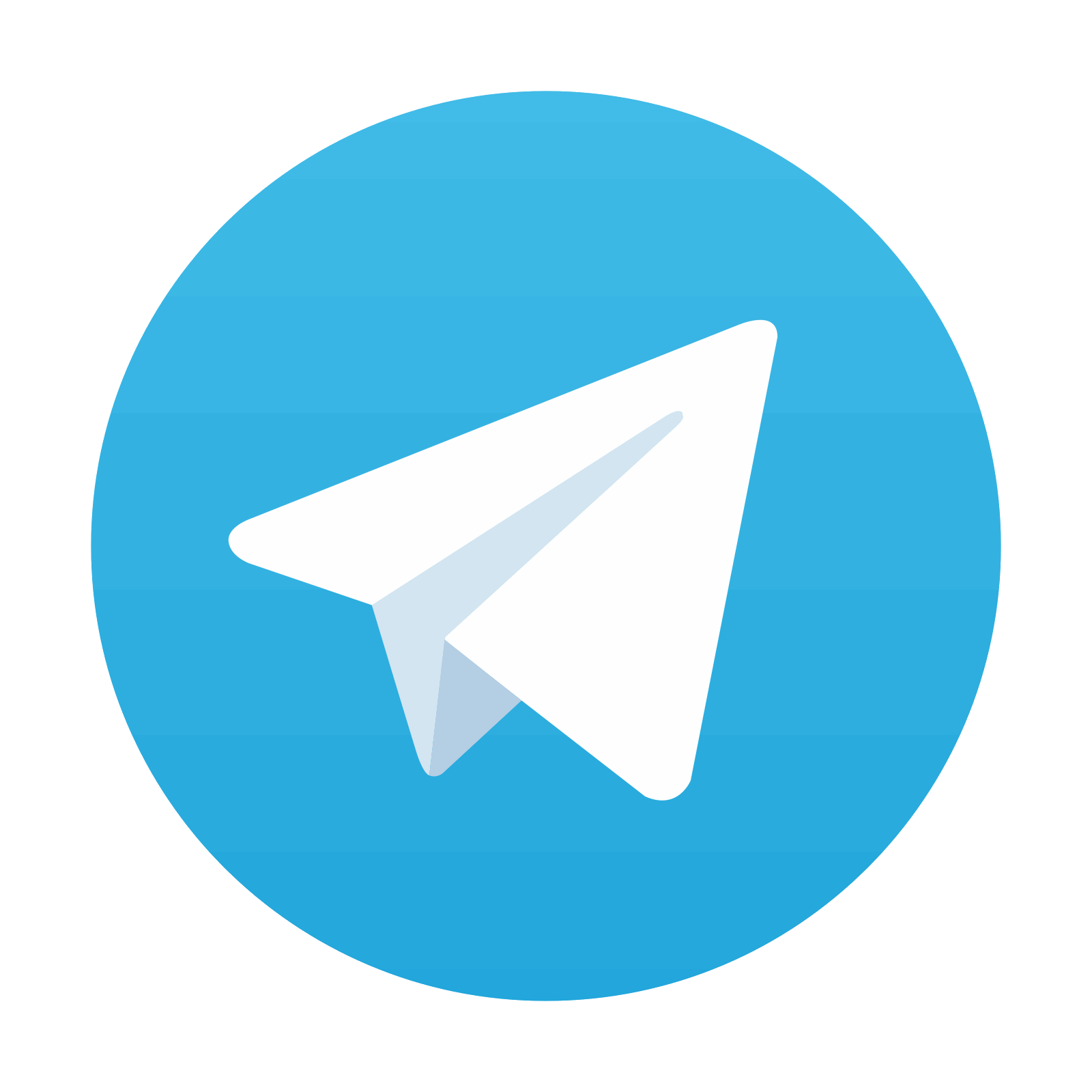
Stay updated, free articles. Join our Telegram channel

Full access? Get Clinical Tree
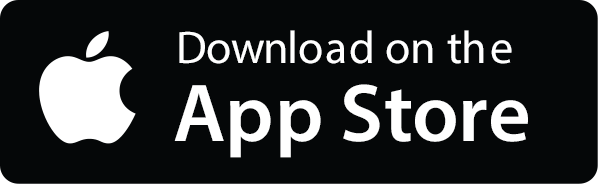
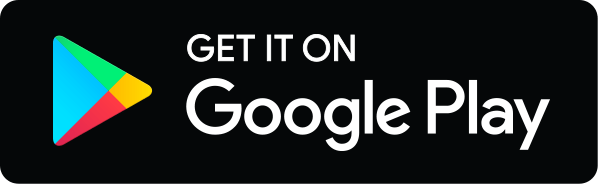
