Fig. 37.1
Chemical structure of porfimer sodium
(a)
Meso-tetrahydroxyphenylchlorin (mTHPC) is a highly active photosensitizer that requires a very low drug dose and is activated by light of wavelength of 648 nm.
(b)
5-Aminolevulinic acid, when administered orally, is metabolized by tissues to a photosensitizer, protoporphyrin IX (PpIX). It is cleared rapidly from the body and the skin photosensitivity effects last for 24 h.
(c)
Lutetium texaphyrin is a synthetic water-soluble compound that is activated by red light in the wavelength range of 720–260 nm, giving greater depth of penetration. It is being studied for cervical, prostate, and recurrent breast cancers.
(d)
Tin ethyl etiopurpurin is a chlorin photosensitizer that has been studied in recurrent cutaneous metastatic breast carcinoma and showed good response without any photosensitive reactions.
(e)
Photochlor or 2-[1-hexyloxyethyl]-2-devinyl pyropheophorbide, an HPPH, is a chlorin-type photosensitizer that absorbs light at 665 nm, giving a better penetration, that is being studied in phase I/II trials for local or advanced lung cancer.
(f)
Mono-l-aspartyl chlorine e6 (NPe6) or talaporfin sodium has been studied in early superficial squamous cell carcinoma of the lung and in central airway lesions greater than 1 cm in diameter. Light activation can be performed at 4 h from administration of the drug and the skin photosensitivity lasts less than 2 weeks. Being activated with light at 664 nm wavelength, it penetrates deeper and can be effective against more invasive tumors.
Light Source
Conventional lamps with filters were initially employed for photoactivation in PDT. While they were relatively inexpensive and easy to use, they produced a significant amount of heat and wide spectra of wavelengths, making calculations of delivered light doses difficult. Lasers, on the other hand, produce a monochromatic light of a specific wavelength, simplifying dosimetry calculations and allowing light transmittal through a flexible optical fiber. Various types of lasers have been used in PDT. Argon dye lasers can be used to activate different photosensitizers because of the capability to alter the wavelength of the light produced by adjusting the filters. Potassium-titanyl-phosphate (KTP) dye laser is readily available in surgical settings and can be fitted with a PDT dye unit (Fig. 37.2). The unit itself can be expensive and bulky. Diode lasers employ semiconductor diode technology. Their main advantages are the compact nature of the laser units and use of standard electrical outlets, making them relatively more portable. The disadvantages are the limited power output and the limitation of a particular laser unit to light of one specific wavelength. A new diode-based system (Diomed Ltd., Andover, MA) is commercially available, compact, and less expensive and is commonly used in clinical settings (Fig. 37.3).
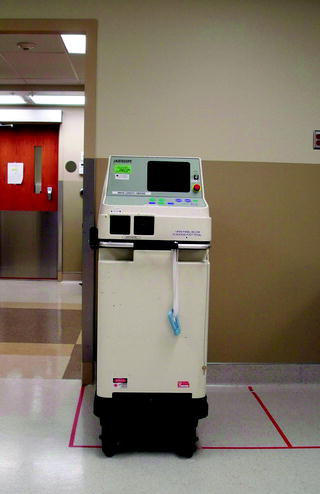
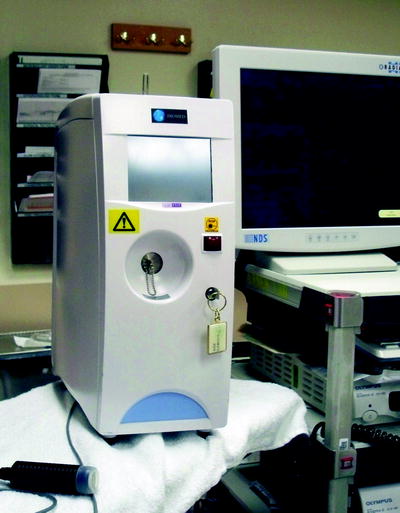
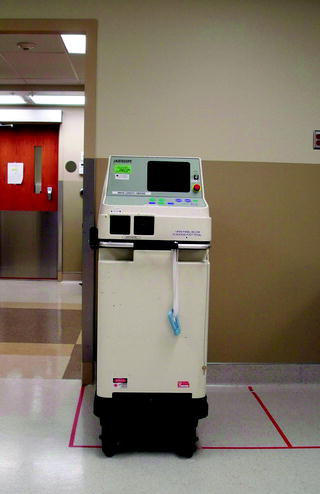
Fig. 37.2
Potassium-titanyl-phosphate (KTP) dye laser unit
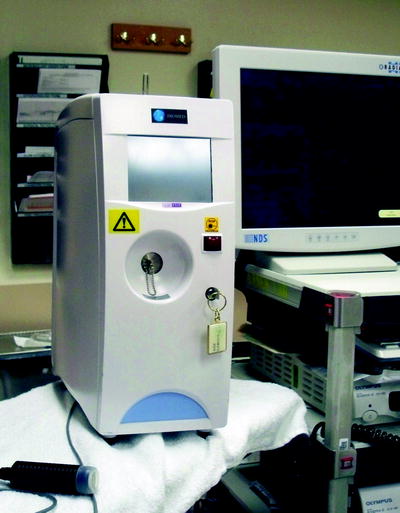
Fig. 37.3
Diode-based laser unit (Diomed Ltd., Andover, MA)
Mechanism of Action
Following intravenous administration, the photosensitizer agent concentrates in tumor tissue, skin, liver, and spleen. The precise reason for this selective retention is unclear and is thought to be secondary to a lower pH or a high concentration of low-density protein receptors on the tumor cells. The exact mechanism of action of PDT is not fully understood and the reason for tumor death is thought to be multifactorial. Upon exposure, porfimer sodium absorbs the light and forms a porphyrin excited state and generates singlet oxygen. The resulting superoxide and hydroxyl radical formation causes apoptosis and cell death. In addition, PDT induces small vessel thrombosis mediated by thromboxane A2 and leads to ischemic necrosis within the tumor. Photodynamic therapy also induces a tumor-specific cytotoxic immunity that can provide a long-term suppression of tumor growth.
With the second-generation photosensitizers that are more specific in their effects on tumor, a better understanding of the precise mechanism of action of PDT is anticipated.
Clinical Technique
Drug Administration
Photofrin (Axcan Pharma, Birmingham, AL) is the commercially available porfimer sodium that is used in PDT. The drug is available in 75-mg vials and should be reconstituted according to the package insert prior to administration. Once reconstituted, it should be protected from bright light and administered immediately.
The recommended dosage is 2 mg/kg body weight, and it is a single dose given as a slow intravenous injection administered over 3–5 min. Care should be taken to avoid extravasation at the injection site. If extravasation does occur, the area must be protected from direct sunlight. Photosensitivity precautions discussed below must be enforced immediately after administration of the drug.
Photoactivation
Activation of the photosensitizer is performed 40–50 h after administration of the drug. Following the peak plasma concentration after intravenous administration, the drug is distributed into the extravascular compartment including liver, spleen, kidneys, as well as tumors, over the next 24 h. The drug is excreted in bile, and 28 % of the drug is eliminated by 72 h with decreasing concentrations in liver, spleen, and kidneys. Due to selective retention, the concentration of the drug in tumors remains elevated for longer periods, and for this reason, photoactivation is recommended at 40–50 h. If necessary, photoactivation may be performed as early as 24 h following administration of the drug.
Flexible bronchoscopy is performed under conscious sedation or general anesthesia. If possible, tumor debulking should be performed while taking care to avoid excessive bleeding. A cylindrical diffuser laser fiber is then advanced into the airway through the working channel of the bronchoscope to activate the drug (Fig. 37.4). The choice of the length of the diffuser depends on the length of the tumor to be treated. If the tumor is longer than the length of the fiber or if multiple endobronchial lesions are present, PDT can be performed on different segments of the tumor sequentially using the same fiber, during the same procedure. In clinical trials, a light dose of 175–300 J/cm was tested, and the efficacy and safety were similar between the lower and the higher doses. A light dose of 200 J/cm (400 mW/cm) of diffuser length is recommended by the FDA for endobronchial tumors. The diode laser is calibrated such that the total power output at the fiber tip occurs with an exposure time of 500 s. For noncircumferential endobronchial lesions, the tumor can be impaled with the fiber tip to provide intralesional activation without exposing the normal bronchial mucosa to light (Figs. 37.5 and 37.6).
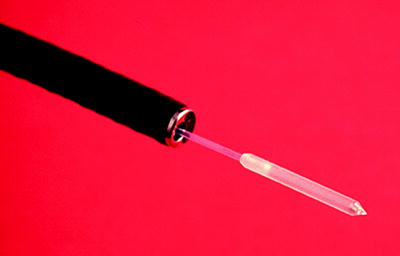
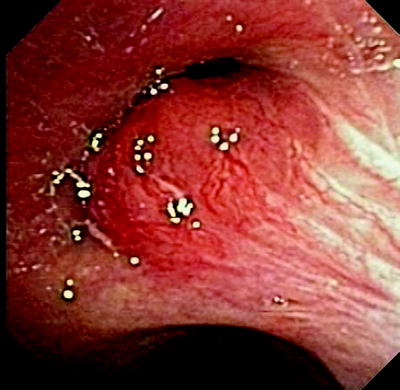
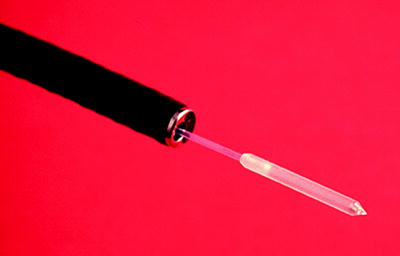
Fig. 37.4
Flexible cylindrical diffuser laser fiber (Courtesy: Armin Ernst)
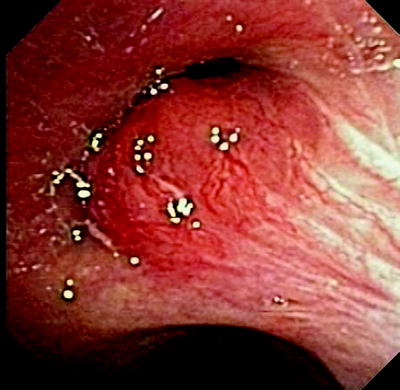
Fig. 37.5
Endobronchial malignant lesion at the secondary carina in the right lung
Debridement
The tumor undergoes necrosis after activation of PDT and necrotic debris accumulates at the site over the next 48 h. A second bronchoscopy is recommended 2–3 days after each light administration for the purpose of debridement and to prevent airway obstruction from the necrotic tumor slough (Fig. 37.7). If any viable and potentially obstructive residual tumor is present, as evident by bleeding during debridement, a second light application may be performed without the need for another injection of the drug. Residual drug is seen in the tumor for up to 120 h after the initial injection.
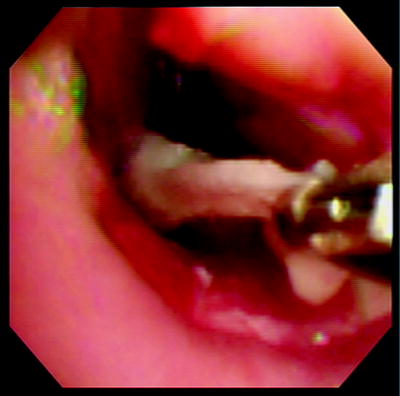
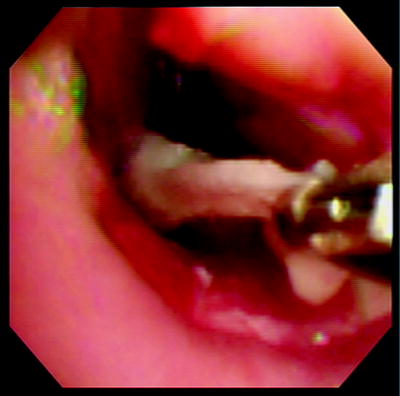
Fig. 37.7
Debridement of necrotic tumor following activation of PDT (Courtesy: Armin Ernst)
If necessary, PDT can be repeated and the current FDA recommendations allow up to three courses of PDT with a minimum of 30-day interval between the treatments. If PDT is being performed after radiation therapy, at least 4 weeks should be allowed between therapies to ensure that the acute inflammation induced by the radiation therapy has subsided prior to PDT.
Indications for PDT in the Lung
Microinvasive Endobronchial Non-Small Cell Lung Cancer (NSCLC)
Patients with early stage NSCLC with the lesions confined to the airway wall typically are treated with surgical resection and have good long-term survival. However, patients may not be suitable for a surgical procedure because of comorbid illnesses, poor pulmonary function, a prior pneumonectomy, or the presence of multifocal endobronchial lesions. Photodynamic therapy is approved for use in such patients, with a curative intent.
The use of PDT in the management of early stage lung cancer was first demonstrated by Hayata et al. in 1982. Since then, numerous trials were conducted showing its efficacy and it acquired FDA approval for use in inoperable early stage NSCLC in 1998. The term “early stage” is used broadly, and in clinical trials, this included stage I lung cancer, microinvasive lung cancer, and carcinoma in situ. Synopsis of the major clinical trials in bronchoscopic PDT for early NSCLC is listed in Table 37.1.
Table 37.1
PDT in early NSCLC
Reference no. | Author and year | Patients/lesions | Drug | Results |
---|---|---|---|---|
2 | Edell and Cortese (1987) | 38/40 | Hematoporphyrin derivative | CR 30 %; PR 70 % |
3 | Edell and Cortese (1992) | 13/14 | Hematoporphyrin derivative | CR 77 %; PR 23 % |
4 | Ono et al. (1992) | 36/39
![]() Stay updated, free articles. Join our Telegram channel![]() Full access? Get Clinical Tree![]() ![]() ![]() |