Non-biological
Biological
Drug development process
Pharmacokinetics
Mainly done in young male animals of well selected genetic background
Body composition
Weight
Reductionist approach
Absorption
Clinical phase 1 and 2 trials mainly include young men
Drug distribution
Metabolic enzymes
Routes of excretion
Pharmacodynamics
Gender differences in:
Blood flow to different organs
Ion channel composition in different organs
When reviewing the existing literature, it is evident that women are under-represented in clinical trials as stated previously, the reasons for this are multifactorial and beyond the scope of this discussion [7]. Recently, the National institutes of Health has committed to a strategic plan to ensure more women are enrolled in clinical studies. The goals for women’s research for 2020 include “increasing sex difference research in basic science studies” as well as “incorporating findings of sex/gender differences in the design and application of new technologies, medical devices, and therapeutic drugs (Table 20.2)” [8]. With the completion of these goals, we hope to gain more knowledge regarding adequate dosing and efficacy of major therapeutic interventions.
Office of Research on Women’s Health 2020 goals |
National Institutes of Health |
U.S. Department of Health & Human Services: |
Increase sex differences research in basic science studies |
Incorporate findings of sex/gender differences in the design and application of new technologies, medical devices, and therapeutic drugs |
Actualize personalized prevention, diagnostics, and therapeutics for girls and women |
Create strategic alliances and partnerships to maximize the domestic and global impact of Women’s Health Research |
Develop and implement new communication and social networking technologies to increase understanding and appreciation of Women’s Health and Wellness Research |
Employ innovative strategies to build a well-trained, diverse, and vigorous Women’s Health Research Workforce |
A number of physiologic differences exist between men and women that in part explain differences in drug effect. Gender differences have been found in regard to blood flow to different organ systems in human and animal models. Males have been shown to have more blood flow to the kidneys and muscle tissue whereas women have more blood flow distributed to adipose tissue and liver. In regards to the heart women have been shown to have a 5 % of blood flow distribution versus 4 % for men but this difference accentuates with pregnancy [9]. Women also have been noted to have higher heart rates at rest, shorter sinus recovery time and longer corrected QT intervals compared with men [5]. Differences in gender hormones may explain some of these findings, but the precise mechanism is not well understood.
These physiological differences between men and women can affect how a medication is absorbed, metabolized and ultimately excreted from the body which describes the processes of pharmacokinetics and pharmacodynamics that will be review below.
Pharmacokinetics in Women
Pharmacokinetics describes what happens to a drug after it has been administered. This includes drug absorption, distribution and elimination or excretion [10, 11]. The goal of any therapeutic agent is to enhance efficacy and minimize toxicity [10, 11]. Therefore pharmacokinetics allows us to safely manage medications by predicting the concentrations in the body and maintaining appropriate therapeutic efficacy.
In order for a medication to reach its site of action, the medication needs to be absorbed. Medication absorption is affected by many different factors including route. Differences in drug route could play a role in the amount of drug that is allowed into the body and the length of time in which a drug takes to reach its desired target organ or receptor. The oral route is much slower compared to other routes such as intravenous or sublingual. For example, consider the use of nitroglycerin for a patient that is experiencing angina. At the first sign of chest pain sublingual nitroglycerin may be given to ensure continued and appropriate blood flow to the heart. By applying the medication under the tongue rather than digesting the tablet orally we allow for quicker response of nitric oxide release therefore faster onset of action and greater efficacy. Oral nitrate tablets have shown much slower time to effect.
Absorption times can be affected by other factors other than route. When comparing absorption rates, women have longer gastrointestinal transit times compared with men, approximately 91.7 h in women and 44.8 h in men and therefore bioavailability of a medication may be greater in women [12–14]. Women also appear to have less gastric acidity therefore absorption of certain medications may be altered. Due to the difference in acidity, there will be decrease absorption for weak acids and increase absorption for weak bases in women [15]. Additionally, women have a smaller body surface and less cardiac output compared to men which may decrease absorption of some medications as well [15]. Once a medication is absorbed, it needs to travel to its site of action. The concept of a medication getting to its site of action is volume of distribution. Factors that affect volume of distribution are protein binding, molecular size of medications, and whether a medication is lipophilic or hydrophilic. In general, no differences have been found between men and women in regards to protein binding [15]. However it has been shown that volume of distribution is decreased in women compare to men. This decrease is thought to be secondary to women having lower total body water, lower intra- and extracellular water, and lower total blood volume. Therefore there will be higher concentrations of water soluble medications in females and potentially an increase in a woman’s clinical response to a water soluble medication [15, 16]. An example of this is atenolol which has been shown to have better blood pressure lowering effects compared to men secondary to the lower volume of distribution [10]. Furthermore, women have a higher percentage of body fat in relationship to total body weight compared to men [17]. In general, body weight increases from young adulthood to the age of 60. After 60, the overall body size decreases and fat in women increases [18]. Therefore, lipid soluble medications, such as benzodiazepines, could distribute to more areas of the body and thereby increasing the effect of the medication in women [12, 16].
After a medication is distributed it is metabolized to allow it to exert its effect. The main site of metabolism is the liver via the cytochrome 450 systems; however other sites of metabolism include the lungs, kidneys, skin, and gastrointestinal tract [13]. There is conflicting information about gender differences in drug metabolism. Even though there is large variation in metabolism of medications, most gender related differences appear to be eliminated by controlling for height, weight, surface area, and body composition [13]. One study by Bebia et al. indicates that the CYP1A system showed decrease clearance of up to 10–20 % in women compared to men. However, another study showed no difference in clearance [5]. Given the conflicting findings, it is postulated that other environmental factors may explain the difference seen in this system. Factors such as diet, smoking, and alcohol use indeed seem to play a role. Furthermore, no consistent gender-related difference in clearance in regards to CYP2C pathway has been shown. The CYP2D6 system has been shown to manifest slower clearance in women compared to men and therefore it has been suggested that the dose of medications that are metabolized through this pathway may need to be reduced by 10–20 %. The CYP3A system was shown by Wolbold et al. to have 15–35 % faster clearance in women suggesting that medications metabolized by this enzyme such as verapamil, nifedipine, and amlodipine may need higher dosing [5, 13, 14, 18]. Table 20.3 summarizes these findings.
CYP1A2 | CYP2C9 | CYP2C19 | CYP2D6 | CYP3A4 |
---|---|---|---|---|
M > F/M = F | M = F | M = F | M > F | M < F |
Clomipramine | Ibuprofen | Diazepam | Codeine | Alprazolam |
Acetaminophen | Warfarin | Omeprazole | Flecainide | Atorvastatin |
Clozapine | Fluvastatin | Citalopram | Fluoxetine | Diltiazem |
Olanzapine | Glipizide | Irbesartan | Metoprolol | Lovastatin |
Theophylline | Losartan | Celecoxib | Mexiletine | Quinidine |
Clopidogrel | Phenytoin | Propranolol | Verapamil | |
Propranolol | Torsemide | Haloperidol | Simvastatin | |
Amiodarone | ||||
Amlodipine |
Once the medication has been used by the body, it needs to be removed. Excretion is final route by which drug metabolites are removed from the body and is primarily handled by the kidneys [13]. The primary technique by which excretion is measured is called clearance. Clearance is defined as a hypothetical volume of distribution of the unmetabolized drug which is cleared per unit of time (ml/min or ml/h) by any pathway of drug removal (renal, hepatic (or) other pathway of elimination) [19]. It has well been established that renal clearance decreases with age. Similarly, several drug trials have shown that women have lower clearance at all ages compared to men [13]. Werner and colleagues also demonstrated that women have less elimination of certain loop diuretics, torsemide specifically, thus leading to higher adverse reactions (ADRs) in women compared to men [20]. For example in a German Pharmacovigilance Project, 66 % of hospitalizations due to torasemide ADRs occurred in women [20]. It is thus critically important to consider women’s decreased renal clearance when prescribing medications that are primarily eliminated by the kidney, especially if a toxic metabolite is formed when the medication is metabolized.
Pharmacokinetic Changes During Pregnancy
Pharmacokinetic studies have shown metabolism of medications via the cytochrome P450 system are altered during pregnancy as summarized in Table 20.4 [21]. As pregnancy progresses, metabolism progressively decreases through the 1A2 pathway. In the third trimester 1A2 and 2C19 activity decreases, 60 and 50 % respectively. However 2A6 increases 54 %, 2A9 increases 20 %, 2D6 increase 50 %, and 3A4 increases 50–100 %. In addition renal metabolism increases 20–65 % in each trimester [21].
Table 20.4
Metabolism during pregnancy
Metabolism | First trimester | Second trimester | Third trimester |
---|---|---|---|
CYP 1A2 | Dec 33 % | Dec 50 % | Dec 60 % |
CYP 2A6 | – | Inc 54 % | Inc 54 % |
CYP 2A9 | No change | No change | Inc 20 % |
CYP 2C19 | – | Dec 50 % | Dec 50 % |
CYP 2D6 | – | – | Inc 50 % |
CYP 3A4 | – | – | Inc 50 – 100 % |
Renal | Inc 20–65 % | Inc 20–65 % | Inc 20–65 % |
A review by Anderson and Carr in the late 1980s and early 1990s estimated that approximately 12 % of women had hypertension during pregnancy [21]. It is expected that this number has increased significantly and therefore the amount of medications used to treat hypertension has also increased. The primary medication used to treat hypertension is methyldopa due to its decreased risk on the fetus (pregnancy category B) [22]. Other treatments which are used less frequency are clonidine, hydralazine, beta blockers and calcium channel blockers [17]. Since atenolol, furosemide and methyldopa are greater than 90, 60–90 and 50 % renally eliminated respectively, we would anticipate that there would be less effect on blood pressure with higher renal clearance as the pregnancy progresses. Nevertheless given decreased fetal risk, methyldopa is favored for BP control during pregnancy. Furthermore, diltiazem and nifedipine are mainly metabolized by CYP 3A4 and metoprolol is mainly metabolized by 2D6, therefore these medications may have less effect of blood pressure during the third trimester since the 3A4 and 2D6 pathways have increased activity during the third trimester [21].
Role of Genetic Polymorphism
Genetic Polymorphism may modify drug response. In the cardiovascular field, this has been associated with the metabolism of beta blockers and calcium channel blockers and response to ACE inhibitors. Some cardiovascular phenotypes are associated with autosomal gene polymorphisms that have been shown to manifest gender differences in drug response [23]. Examples of these are shown in Table 20.5. Genes located in the X chromosome are also good candidates to account for some gender differences. Carrel and Willard among others have extensively studied how mutations in these genes or functionally related polymorphisms might be better compensated in women [24]. For example genes for Angiotensin Converting Enzyme −2 (ACE-2) and angiotensin II receptor (ATR2) are located in the X chromosome [25]. The AT2R has been shown to modulate left ventricular hypertrophy in women with HCM independently of the circulating RAS but this has not been shown in men. Studies have suggested that LV mass in women is in part regulated by number of specific alleles of the AT2R gene [26]. In Transgenic mice models a more severe cardiovascular phenotype was seen in male rather than female animals and this correlated to earlier development of and faster progression to heart failure [27]. Interestingly, a variety of factors and complex interactions ranging from diet, sex hormonal influences, removal of ovaries and administration of exogenous estrogen, or age, seem to change gene expression adding to the complexity of the pathways that lead to gender differences in drug responses [28].
Table 20.5
Gender differences in drug effects of some common medications (not inclusive)
Adverse effects | Trend towards benefit | Require dose adaptation |
---|---|---|
Digoxin (^ mortality) in women with heart failure | Calcium channel blockers – amlodipine | BB – metoprolol |
QT prolonging drugs (^ Torsades) | Ramipril | CCB – verapamil |
ACE I (^ cough) | Eplerenone |
Role of Sex Hormones
Estrogens and progestins interacts with a large number of cardiovascular drugs possibly by inhibiting CYP enzymes or increasing drug glucuronidation [5]. Menstrual cycle, pregnancy, and menopause can modify this interaction due to variation of levels of estrogen and other hormones, alterations in total body water due to renal plasma flow variations and changes in glomerular filtration during these specific periods for women. For example, it has been reported that menstruation, pregnancy and, ovariectomy can modulate CYP2D6 activity [29]. The clinical relevance of these changes is not clear. Interactions with exogenous hormone therapy such as hormone replacement therapy (HRT) and oral contraceptives must also be taken into account. Estrogens seem to interfere with the synthesis of Angiotensinogen in the liver and also with the expression of Angiotensin I receptor (ATR-1) in the myocardium [30]. On the other hand estrogens appear to increase the expression of AT2R in the myocardium. In the cardiovascular system, progesterones appear to have a partially synergistic and/ or antagonistic relationship with estrogens [31]. In some animal models (ovariectomized rabbits), progesterone was seen to exert a direct inhibitory effect on the atheroprotective action of estrogen [31], while in other models (ischemic rats) progesterone has been shown to have cardioprotective effects but only in female ischemic rats. These findings suggest complex interactions with endogenous estrogen.
Gender differences in drug metabolism can in part be explained by the dismorphic expression of CYP450 and other liver-expressed genes (females predominantly express CYP3A4). This expression is in part regulated by GH (Growth Hormone) release by the pituitary gland which shows significant gender difference in its pattern of release [32]
Pharmacodynamics in Women
Pharmacodynamics describes the principle of drug action and its resulting effect at its target site, i.e. receptor or membrane [10]. This concept is often used to examine overall maximum effect of a drug and/or the sensitivity of the target area to the drug concentration [11]. Current literature regarding differences of pharmacodynamics between men and women is limited. Most pharmacodynamic data deals primarily with the elderly. Despite the lack of pharmacodynamic studies in women we can hypothesize that there may be differences in drug response due to gender by evaluating adverse drug reports.
Currently, the Food and Drug Administration (FDA) holds a database in which patients and providers can voluntarily document adverse events. The reporting system is known as FAERS (FDA Adverse Event Reporting System) which allows the FDA to monitor medication and report post-marketing data to the public [33]. A study by Moore et al. examined adverse event data from FAERS between 1998 and 2005. In their analysis they found that more women had adverse events (55.5 %) compared to men (45.5 %) and the adverse effects were more serious in women [15]. When age was closely examined patients between 45 and 64 years old had 33.7 % of the events which accounted for 22.2 % of the total population. Individuals that were greater than or equal to 65 years old were found to have 33.6 % of the adverse events but only accounted for 12.6 % of the total population. Of the cardiovascular drugs that totaled 500, more adverse events in any year were reported in women. HMG-CoA reductase inhibitors (statins) were associated with the most issues. Cerivastatin, which was later withdrawn from the market, was found to have 1,573 events [34]. Furthermore, out of 10 medications that were withdrawn from market during 1997 and 2000, 8 were withdrawn secondary to greater adverse effects in women [15]. In general women have a 1.5- to 1.7-fold greater risk of developing an adverse drug reaction. The reasons for this increased risk are not entirely clear but include gender-related differences in pharmacokinetics already discussed, immunological and hormonal factors as well as differences in the use of medications by women compared with men, polypharmacy and increasing age.
Gender Differences Within Specific Medication Classes and Medications
Beta Blockers
Gender-specific differences in the pharmacokinetics of beta-blockers lead to greater drug exposure in women [35]. The pharmacokinetics differences can be due to lower volume of distribution and slower clearance. Furthermore, pharmacodynamic differences may be secondary to estrogen deficiency in older women which can modulate the up regulation of beta receptors therefore more receptors to exert its effect [5]. Hormone supplementation with estrogens and progestins can prevent such upregulation [36]. Since sex hormones can modulate the regulation of beta adrenergic receptors in the heart and vessels, gender specific differences in the pharmacodynamics of beta receptor blockers are to be expected [5]. Gender differences have been described for response to cardio selective and non-selective beta blockers. Men have greater clearance of both selective and non-selective beta blockers leading to faster clearance of these drugs compared to women [35]. Higher beta-blocker plasma levels in women translates into a more pronounced decrease in heart rate and systolic blood pressure than men [35].
Women have been a minority in heart failure clinical trials testing beta-blockers, representing 20–30 % in the first major trials [37]. Two major trials, the MERIT Heart Failure study and the COPERNICUS trial, failed to find a beneficial effect on mortality in women [38, 39]. In a detailed gender-specific post-hoc analysis for the CIBIS II study, women profited significantly from treatment with bisoprolol which had a greater unadjusted effect on all-cause mortality in women than in men [40]. Pooling of mortality results from MERIT Heart Failure, CIBIS II and COPERNICUS showed survival benefits in both women and men [41] (Fig. 20.1). The lack of evidence in some large beta-blocker studies is therefore probably due to the under representation of women in these trials.
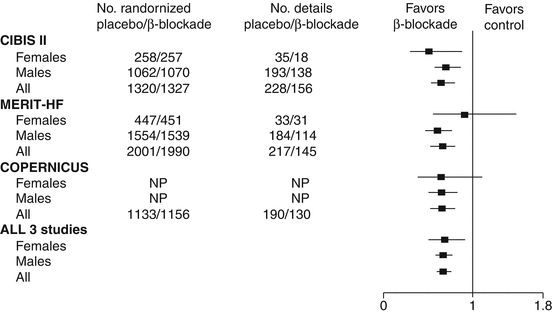
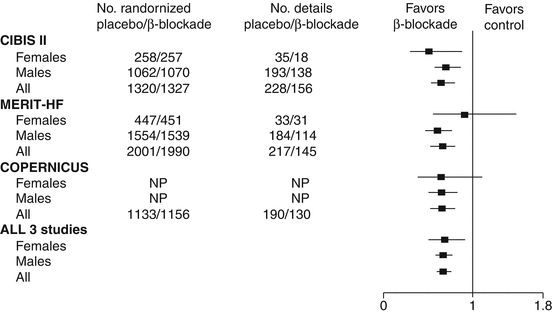
Fig. 20.1
Relative risk ratios and 95 % confidence intervals for total mortality in women and men, in studies evaluating the impact of B-blockade in heart failure (Adapted with permission from Jochmann et al. [5])
Findings of beta-blocker therapy in secondary prevention after myocardial infarction have revealed conflicting findings with respect to gender specific differences. However, most of these studies have not included sufficient number of women to enable definitive conclusions [42]. A meta-analysis of more than 5,000 patients (1,121 women) investigating effect of metoprolol post myocardial infarction showed that reduction of cardiovascular death was comparable in women and men [43]. Similarly, the ISIS-I and ISIS-II trials demonstrated that improved survival in women receiving beta blockers and aspirin was comparable to that in men [44, 45].
In summary, beta blockers are effective for women in ischemic heart disease and heart failure, though data is not as extensive and as solid as it is for men. Caution should be exercised in dosing beta-blockers in women as they tend to be poor metabolizers leading to higher plasma concentration of these drugs compared to men.
ACE Inhibitors and ARBs
The Renin-Angiotensin System (RAS) is a key regulator of blood pressure Endogenous RAS activity differs between men and women [46]. Gender differences may also be seen with medications that work against the RAS. Estrogens increase angiotensin II (Ang II) plasma levels, leading to a decrease of angiotensin converting enzyme (ACE) and renin activity via negative feedback. Therefore estrogens net effect is to decrease the activity of the RAS, leading to less effect when using angiotensin converting enzyme inhibitors (ACE-I) or angiotensin receptor blockers (ARB) [46]. Subsequently, premenopausal women show lower ACE activity than post-menopausal women but this difference is abolished in woman taking exogenous estrogen [47].
ACE-I (and more recently ARBs) have been part of evidence-based therapy for heart failure and hypertension for decades. Several multicenter studies, e.g. CONSENSUS I, SAVE, and SOLVD, have shown a much smaller mortality reduction in women compared to men. However it is now recognized that these findings were likely due to the small percentage of women included in those trials [48]. Later trials, including a meta-analysis of more than 7,000 patients in 1995, have shown comparable benefits in women and men [49]. However, on the basis of the small proportion of women included in ACE-I studies, data for women are less advantageous than for men and the question of less benefit from this therapy based on gender remains unanswered. Although animal models have shown gender differences in response to Ang II even during endogenous blockade of the ACE system, this has not been consistently seen in human studies [47].
< div class='tao-gold-member'>
Only gold members can continue reading. Log In or Register a > to continue
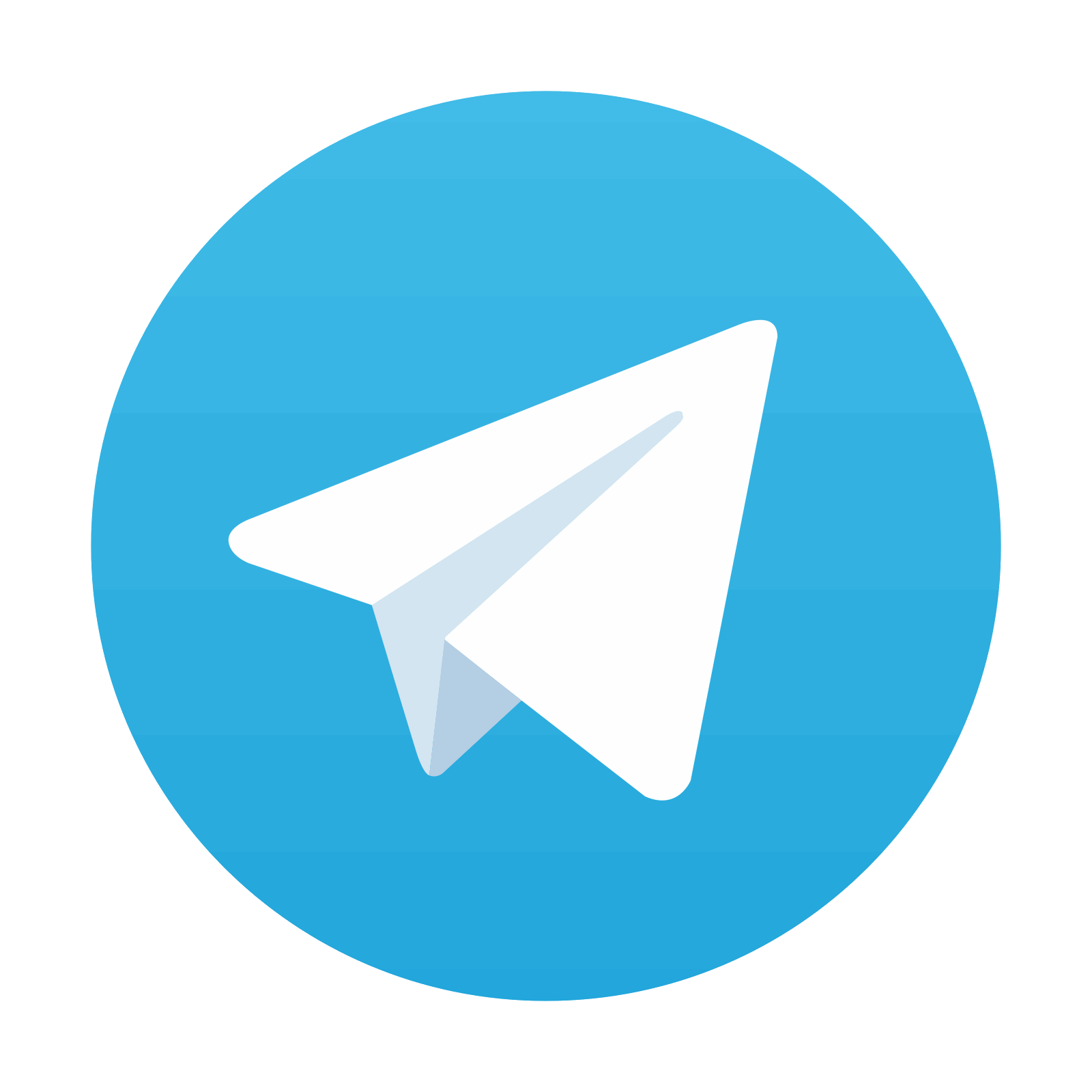
Stay updated, free articles. Join our Telegram channel

Full access? Get Clinical Tree
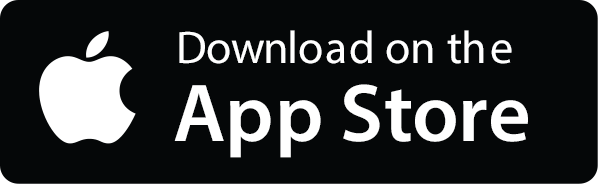
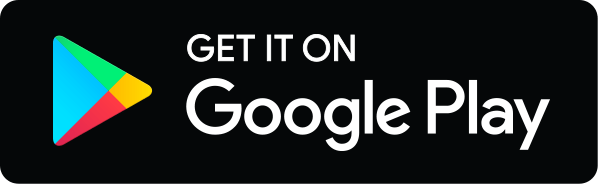