Background
The aim of this study was to investige serial changes of myocardial deformation using two-dimensional speckle-tracking echocardiographic (2DSTE) imaging in children undergoing balloon valvuloplasty for congenital valvular aortic stenosis (VAS).
Methods
Thirty-seven children with isolated congenital VAS were enrolled in this study prospectively. Patients underwent echocardiographic evaluation at three instances: before balloon valvuloplasty, 6 months after intervention, and 3 years after intervention. Longitudinal, circumferential, and radial peak systolic strain values were determined, as well as systolic strain rate and the time to peak global systolic strain. Linear mixed statistical models were used to assess changes in 2DSTE parameters after balloon intervention. Using one-way analysis of variance, 2DSTE results at 3-year follow-up were compared with 2DSTE measurements in 74 healthy age-matched children and 76 children with uncorrected VAS whose severity of stenosis corresponded to residual stenosis of study subjects at 3-year follow-up.
Results
Global peak strain and strain rate measurements in all three directions were decreased before intervention compared with healthy children. Global peak strain and strain rate measurements increased significantly ( P < .001) several months after balloon valvuloplasty and continued to increase at 3-year follow-up. However, at 3-year follow-up, global peak strain and strain rate in the longitudinal and circumferential directions were significantly lower ( P < .001) compared with both control groups. Measurements of time to peak global systolic strain were significantly shorter at early follow-up compared with measurements before intervention ( P < .05).
Conclusions
Shortly after balloon valvuloplasty for severe congenital VAS, there is an improvement in systolic myocardial deformation. However, 2DSTE parameters do not return to normal at 3-year follow-up. These abnormalities in systolic deformation cannot be fully attributed to residual stenosis or aortic regurgitation.
Patients with congenital valvular aortic stenosis (VAS) are subjected to long-standing pressure overload of the left ventricle, leading to alterations in left ventricular (LV) architecture and myocardial dysfunction. Previous studies in both children and adults have indicated that abnormal LV systolic function starts at an early stage of the disease, with progressive reduction of regional and global deformation. However, those early alterations in myocardial function have proven to be difficult to detect in congenital VAS with conventional (echocardiographic) imaging modalities. Two-dimensional (2D) strain echocardiographic or 2D speckle-tracking echocardiographic (2DSTE) imaging has emerged as a relatively new index of regional and global myocardial function. The spectrum of potential clinical applications is very wide. Because of its ability to differentiate between active and passive deformation of myocardial segments, to quantify intraventricular dyssynchrony, and to evaluate individual components of myocardial function such as longitudinal myocardial shortening, 2DSTE imaging can detect myocardial dysfunction at an early stage. Previous studies have indicated that 2DSTE imaging is more sensitive than conventional echocardiography in detecting early myocardial dysfunction in VAS. Two-dimensional strain parameters have been shown to be abnormal even in children with asymptomatic, mild congenital aortic stenosis. In adults with degenerative VAS, these abnormal alterations in (regional) deformation appear to be only partially reversible after aortic valve replacement (AVR). In children with congenital isolated VAS, the treatment of choice is valvulotomy, either surgical or balloon valvuloplasty. The reversibility of systolic myocardial dysfunction after valvulotomy in children with congenital VAS has not been established by means of 2DSTE imaging.
The aim of the present study was twofold: first, to assess the effects of balloon valvuloplasty on myocardial deformation assessed with 2DSTE imaging in all three directions of deformation (longitudinal, circumferential, and radial) in children with isolated congenital VAS, and second, in case of persistent abnormal 2DSTE findings at 3-year follow-up to determine whether these abnormalities could be attributed to a residual valvar stenosis.
Methods
Study Population
All asymptomatic children with severe isolated congenital VAS visiting the outpatient clinic at the Children’s Heart Centre Nijmegen between May 2005 and May 2009, who were scheduled for balloon valvuloplasty, were prospectively analyzed for their eligibility for inclusion in the present study. Inclusion criteria consisted of isolated congenital VAS (e.g., thickening of the valve or functional bicuspid aortic valve), accompanied by a repeated Doppler-derived peak systolic instantaneous aortic flow velocity ≥ 4.0 m/sec (which corresponds to a transvalvular pressure gradient of >64 mm Hg) at subsequent visits. Peak LV–to–peak aortic pressure gradient assessed during catheterization under general anesthesia had to be confirmative of aortic stenosis (according to the American College of Cardiology and American Heart Association 2006 guidelines for the management of patients with valvular heart disease ). Previous studies have indicated that general anesthesia results in a decreased stroke volume and lower pressure gradient estimations. Exclusion criteria were (1) moderate or severe aortic regurgitation (i.e., grade > 1), (2) (a history of repeated) abnormal cardiac rhythm such as (supra)ventricular tachycardia, (3) previous balloon and/or surgical valvulotomy, (4) acute or chronic illness at the time of echocardiographic evaluation, and (5) metabolic and/or genetic syndrome. Demographic and anthropometric characteristics, including age and gender, were collected at the same time the echocardiographic study was performed. A complete history, a physical examination, electrocardiography, and echocardiographic or 2DSTE examinations were performed three times: (1) <1 month before balloon valvuloplasty, (2) at intermediate follow-up (6 months) after balloon intervention, and (3) at 3-year follow-up.
Control Groups
Subjects who were routinely referred for echocardiographic evaluation of asymptomatic, innocent heart murmurs or for screening purposes between May 2005 and November 2009 were retrospectively analyzed for their eligibility for inclusion in the study to serve as a control group. Patients with structural (congenital) heart disease, abnormal cardiac rhythm, and/or (histories of) chronic or acute illness were excluded. A group of 74 healthy, age-matched children was included and examined to provide normal reference values for conventional echocardiographic and 2DSTE parameters.
A total of 76 children, consisting of a cohort of 45 children with mild, isolated congenital VAS (peak systolic flow velocity, 2.5–3.0 m/sec) and a cohort of 31 children with moderate, isolated congenital VAS (peak systolic flow velocity, 3.0–4.0 m/sec) without prior surgical or balloon intervention were previously analyzed and described by our research group. In the present study, their 2DSTE results were compared with 2DSTE findings in children with residual aortic stenosis at 3-year follow-up after balloon intervention.
This study was approved by the local ethics committee and conformed with the Declaration of Helsinki. Consent was given by each participant and/or his or her parents.
Conventional Echocardiographic Parameters
All subjects underwent detailed transthoracic echocardiographic examinations in the left lateral position according to the recommendations of the American Society of Echocardiography and a local research protocol previously described by Mavinkurve-Groothuis et al. Every examination was performed at rest, without using sedation. Images were obtained with a 3.0-MHz (S3) or a 5.0-MHz (S5) phased-array transducer using a commercially available system (Vivid 7; GE Vingmed Ultrasound AS, Horten, Norway). The choice of an S3 or an S5 transducer depended on the age and posture of the child. Quantification of cardiac chamber size, ventricular mass, and systolic LV function was performed in accordance with the recommendations for chamber quantification by the American Society of Echocardiography’s Guidelines and Standards Committee and the Chamber Quantification Writing Group. LV systolic function was characterized using fractional shortening, ejection fraction, pulsed-wave Doppler–derived LV myocardial performance index (or Tei index), and rate-corrected velocity of circumferential fiber shortening. Ejection fraction was calculated using the modified Simpson’s rule. The obtained values of LV mass (corrected for body surface area) were compared with those previously described in normal, healthy children.
2DSTE Data Acquisition
Two-dimensional multiframe B-mode (grayscale) images were obtained from the apical four-chamber, the parasternal midcavity short-axis view (at the level of the papillary muscle), and parasternal basal short-axis view (at the level of the mitral valve). A sector scan angle of approximately 60° was chosen, and frame rates of 60 to 90 Hz were used because these rates are considered to be optimal for 2D speckle tracking. Data were stored at the same frame rate as the acquisition frame rate. Preferably, images from five cardiac cycles triggered by the R wave of the QRS complex were digitally saved in cine loop format. Offline strain analysis was performed using speckle-tracking software for echocardiographic quantification (EchoPAC version 6.1.0; GE Vingmed Ultrasound AS). The timing of aortic valve closure and mitral valve opening with respect to peak strain and peak systolic strain were manually obtained, using single gated pulsed-wave Doppler or continuous wave Doppler blood flow velocity images of the LV outflow tract. For these measurements, special care was taken to keep the heart rate in the same range as during the 2D grayscale imaging used for 2D strain calculations. Endomyocardial borders of the left ventricle were manually tracked within the end-systolic frame. The second, epicardial tracing was generated by EchoPAC and, when necessary, manually adjusted to cover the whole myocardial wall. The tracking algorithm then followed the myocardial speckles through the cardiac cycle. Tracking was accepted only if both visual inspection and EchoPAC indicated adequate tracking. The software automatically divided the cross-sectional image into six segments, which were named and identified according to the statement of the Cardiac Imaging Committee of the Council on Clinical Cardiology of the American Heart Association. The LV segments to be analyzed were the apical, mid, and basal segments of the septal and the lateral wall in the four-chamber view, as well as the anteroseptal, anterior, lateral, posterior, inferior, and septal segments in the basal (mitral valve) and midcavity (papillary muscle) short-axis views. Strain curves of the three consecutive cardiac cycles and values of the manual timing were imported into a custom-made software package to average the strain curves of three consecutive cycles. The Q-Q interval was determined from the electrocardiographic signal to obtain cardiac cycle length. Cardiac cycles with lengths >10% different from the mean length of the three cardiac cycles were excluded from averaging and thus from further analysis. Myocardial longitudinal, radial, and circumferential strain values were obtained, as well as peak systolic strain rate and the time to reach peak systolic strain. To determine global strain and strain rate, the strain and strain rate values of the six segments were averaged for the four-chamber as well as for the short-axis views. All 2DSTE analyses were performed by the same investigator (K.A.M.) to avoid interobserver variability. Intraobserver variability scores have been previously described by our research group. Strain values are dimensionless and are expressed as percentages. Strain rate is the time derivative of strain and is expressed per second. Time to peak systolic strain is expressed in milliseconds. Negative strain values reflect shortening or thinning, while positive strain values reflect lengthening or thickening.
Statistical Analysis
All normally distributed demographic, anthropometric, conventional echocardiographic, and 2D strain values are expressed as mean ± SD and all data not normally distributed as medians and ranges. Conventional echocardiographic as well as 2DSTE measurements derived from the children with severe VAS before intervention were compared with those acquired at intermediate and late follow-up. The changes in each standard echocardiographic finding and each 2DSTE parameter were reported using linear mixed models and Bland-Altman plots. Because it is possible that changes in 2DSTE results after intervention (especially at 3-year follow-up) could be (partly) biased by maturational changes, all 2DSTE results were compared with pediatric reference values established by our research group. For all individual 2DSTE results of each study subject (before intervention and at follow-up), the deviation from normal was calculated (2DSTE result of the individual patient minus the mean reference value of 2DSTE parameter at the same age). The change in deviation from normal for each 2DSTE parameter at follow-up was determined by using linear mixed models. Two-dimensional speckle-tracking echocardiographic measurements at 3-year follow-up were compared with respective findings in both control groups: (1) a cohort of 74 healthy, age-matched children and (2) 76 children with mild ( n = 45) or moderate ( n = 31) isolated congenital VAS without prior surgical or balloon intervention. For the latter comparison, patients with VAS at 3-year follow-up were divided according to residual VAS before further analysis. None to mild VAS was defined as a peak systolic flow velocity < 3.0 m/sec and moderate VAS between 3.0 and 4.0 m/sec, and patients with systolic peak jet velocities > 4.0 m/sec were considered to have severe VAS. Subsequently, global peak systolic strain, global peak systolic strain rate, and time to peak global systolic strain measurements were compared with respective findings in children with corresponding peak systolic flow velocity measurements but no prior balloon intervention as well as healthy, age-matched children. To determine their relation, one-way analysis of variance was used with Bonferroni’s correction for multiple comparisons. To determine the relation between individual 2DSTE parameters and aortic regurgitation at 3-year follow-up, one-way analysis of variance was performed. Relations between preintervention conventional echocardiographic and 2DSTE parameters and respective postintervention findings were expressed in terms of linear regression analyses. All 2DSTE parameters were normally distributed. P values < .05 were considered to indicate significance. All statistical analyses were performed using SPSS for Windows version 16.0 (SPSS, Inc., Chicago, IL).
Results
Study Population
A total of 51 children who underwent a balloon valvuloplasty to relieve pressure overload due to severe congenital VAS was identified from our outpatient clinic for possible inclusion in the present study.
Fourteen of those patients were subsequently excluded: in two cases, VAS was accompanied by another congenital heart defect; three patients had moderate to severe aortic regurgitation; eight patients had histories of valvuloplasty before the present valvuloplasty; and one patient had been diagnosed with Turner syndrome. Ultimately, 37 pediatric patients aged 0 to 15 years were eligible for inclusion in the study. Their Doppler-derived peak instantaneous systolic flow velocity across the aortic valve before intervention was on average 4.8 m/sec, corresponding to a pressure gradient across the stenotic valve of 91 mm Hg. The peak-to-peak gradient at catheterization was 64 mm Hg (range, 51–75 mm Hg). All catheterizations were performed under general anesthesia. Of those 37 patients, one patient underwent balloon valvuloplasty <1 year before the end of the inclusion period, and therefore no information regarding 3-year follow-up findings was available for this particular patient.
History, Physical Examination, and Electrocardiography
History and physical examination before the intervention were unremarkable, with the exception of a systolic murmur. All patients were asymptomatic and in New York Heart Association functional class I. Electrocardiographic findings did not reveal abnormal cardiac rhythms, conduction disorders, or signs of ischemia. There were no T-wave abnormalities indicative of cardiac strain at rest. In five patients, electrocardiographic findings were suggestive of LV hypertrophy, with increased LV forces and leftward deviation of the QRS axis. Echocardiography confirmed the presence of LV hypertrophy in three of these patients. At intermediate (5.8 ± 1.5 months after balloon intervention) and late (3.4 ± 0.7 years after balloon intervention) follow-up, patients were free from cardiac symptoms. As expected, several anthropometric characteristics of the study subjects were statistically significant different ( P < .05) at 3-year follow-up compared with preintervention measurements by linear mixed models. These small but statistically significant differences can be fully explained by maturational changes. Patient demographic characteristics and anthropometric parameters before the intervention and at follow-up are listed in Table 1 . Demographic and anthropometric characteristics of control subjects and study subjects at 3-year follow-up are described in Table 2 . There were no statistically significant differences in anthropometric parameters among the various groups of children under investigation (children with severe VAS at 3-year follow-up after balloon valvuloplasty, healthy controls, and VAS patients without prior intervention).
Characteristic | Before intervention ( n = 37) | 6-mo follow-up ( n = 37) | P | 3-y follow-up ( n = 36) | P |
---|---|---|---|---|---|
Male | 25 (68%) | 25 (68%) | — | 25 (68%) | — |
Age (y) | 6.7 (0.0–15.5) | 7.3 (0.3–16.3) | .52 | 10.3 (2.5–19.4) | .04 ∗ |
Height (cm) | 114 ± 42 | 121 ± 39 | .35 | 139 ± 30 | .08 |
Weight (kg) | 26.5 (2–65) | 30.4 (5–66) | .38 | 38.8 (12–73) | .14 |
BSA (m 2 ) | 0.90 ± 0.51 | 0.99 ± 0.51 | .37 | 1.20 ± 0.46 | .11 |
BMI (kg/m 2 ) | 17.1 ± 2.9 | 17.5 ± 3.9 | .58 | 18.3 ± 4.5 | .45 |
HR (beats/min) | 99 ± 32 | 95 ± 32 | .45 | 86 ± 21 | .38 |
MAP (mm Hg) | 64 ± 10 | 67 ± 9 | .17 | 74 ± 9 | .002 ∗ |
SBP (mm Hg) | 91 ± 13 | 94 ± 11 | .30 | 103 ± 13 | .003 ∗ |
DBP (mm Hg) | 51 ± 10 | 54 ± 9 | .13 | 60 ± 8 | .005 ∗ |
∗ P < .05 compared with the same parameter at the prior examination by linear mixed models.
Characteristic | 3-y follow-up | No prior intervention | Healthy control subjects ( n = 74) | ||
---|---|---|---|---|---|
None or mild residual stenosis ( n = 17) | Moderate residual stenosis ( n = 19) | Mild VAS ( n = 45) | Moderate VAS ( n = 31) | ||
Male | 11 (65%) | 12 (64%) | 31 (69%) | 23 (74%) | 50 (68%) |
Age (y) | 10.2 (3–18) | 10.3 (3–19) | 10.2 (2–18) | 9.0 (0–17) | 10.3 (3–19) |
Height (cm) | 140 ± 30 | 139 ± 31 | 137 ± 30 | 133 ± 30 | 141 ± 30 |
Weight (kg) | 37.4 (12–68) | 40.0 (14–73) | 36.2 (9–75) | 31.0 (7–86) | 38.7 (13–70) |
BSA (m 2 ) | 1.19 ± 0.44 | 1.22 ± 0.48 | 1.20 ± 0.44 | 1.13 ± 0.45 | 1.21 ± 0.47 |
BMI (kg/m 2 ) | 17.6 ± 3.1 | 18.9 ± 5.4 | 17.9 ± 3.2 | 18.1 ± 3.6 | 17.8 ± 2.9 |
HR (beats/min) | 79 ± 19 | 83 ± 23 | 81 ± 20 | 83 ± 22 | 79 ± 16 |
MAP (mm Hg) | 76 ± 7 | 73 ± 10 | 75 ± 9 | 76 ± 10 | 74 ± 9 |
SBP (mm Hg) | 106 ± 11 | 101 ± 14 | 103 ± 13 | 100 ± 11 | 103 ± 12 |
DBP (mm Hg) | 61 ± 7 | 59 ± 9 | 60 ± 8 | 61 ± 11 | 60 ± 6 |
Conventional Echocardiography
Conventional echocardiographic findings are shown in Table 3 . Before intervention, echocardiographic findings indicated significant LV hypertrophy in three patients. At that time, mild aortic regurgitation was present in 11 of 37 patients. Conventional systolic echocardiographic indices were normal in all echocardiographic examinations performed before the intervention. At intermediate and 3-year follow-up, none of the patients had significant LV hypertrophy. Mild aortic regurgitation was present in 19 of 37 patients, whereas moderate aortic regurgitation was revealed in two patients. After the intervention, conventional functional echocardiographic findings did not indicate systolic LV dysfunction and were all within normal limits. Each conventional echocardiographic finding of the preintervention examination was similar to respective echocardiographic findings at follow-up, as determined by linear mixed models. Peak instantaneous systolic flow velocity across the aortic valve and the Bernoulli equation–derived peak pressure gradient across the stenotic valve were the only conventional parameters that changed after balloon valvuloplasty. Both declined significantly ( P < .001) within 6 months after balloon valvuloplasty and remained stable at 3-year follow-up.
Parameter | Before intervention ( n = 37) | 6-mo follow-up ( n = 37) | P | 3-y follow-up ( n = 36) | P |
---|---|---|---|---|---|
Peak velocity (m/sec) | 4.8 (4.1 to 6.2) | 3.2 (2.1 to 4.1) | <.001 ∗ | 3.1 (1.9 to 3.9) | .46 |
Peak pressure (mm Hg) | 91 (67 to 154) | 42 (18 to 68) | <.001 ∗ | 39 (14 to 61) | .49 |
Mitral A velocity | 0.59 ± 0.12 | 0.58 ± 0.12 | .72 | 0.57 ± 0.14 | .88 |
Mitral E velocity | 1.00 ± 0.17 | 0.98 ± 0.17 | .50 | 1.03 ± 0.17 | .19 |
Mitral E/A ratio | 1.75 ± 0.41 | 1.74 ± 0.39 | .91 | 1.85 ± 0.33 | .27 |
LVETc (sec) | 0.30 ± 0.02 | 0.30 ± 0.02 | .83 | 0.30 ± 0.02 | .76 |
VCFc (circ/sec) | 1.30 ± 0.14 | 1.29 ± 0.10 | .49 | 1.27 ± 0.10 | .21 |
Tei index | 0.39 ± 0.02 | 0.38 ± 0.02 | .64 | 0.39 ± 0.02 | .58 |
FS | 0.40 ± 0.04 | 0.39 ± 0.02 | .16 | 0.38 ± 0.03 | .22 |
Biplane LVEF | 0.74 ± 0.04 | 0.73 ± 0.05 | .36 | 0.72 ± 0.05 | .92 |
LVM (g) | 68.1 ± 37 | 71.2 ± 39 | .88 | 84.9 ± 42 | .26 |
Z -score | +0.85 (−0.30 to +2.45) | +0.54 (−0.76 to +1.70) | −0.03 (−1.14 to +1.33) | ||
LVM/BSA (g/m 2 ) | 75.7 ± 18 | 71.9 ± 14 | .52 | 70.8 ± 16 | .83 |
∗ P < .05 compared to the same echocardiographic parameter at the prior examination by linear mixed models.
2DSTE Results
Tracking was feasible in 98% of all segments in the four-chamber view, in 97% of all segments in the short-axis view at the level of the papillary muscle, and in 93% of all segments in the short-axis view at the level of the mitral valve. The 2DSTE findings before the intervention and at follow-up are presented in Table 4 and Figures 1 A to 1 C. One important observation was a strong, statistically significant change in 2DSTE parameters after balloon valvuloplasty. At intermediate follow-up, global peak systolic strain in all three directions (longitudinal, circumferential, and radial) improved significantly compared with preintervention measurements by means of linear mixed models. Peak systolic strain in all three directions continued to change thereafter. At 3-year follow-up, peak systolic strain in all three direction was significantly improved compared with findings at intermediate follow-up. Time to global peak systolic strain values at intermediate follow-up were significantly shorter in the radial direction compared with preintervention measurements. On the contrary, time to peak global systolic strain values at 3-year follow-up were significantly longer (in all three directions of contraction) compared with findings at intermediate follow-up and time to peak global systolic strain results acquired before the intervention. The latter finding could be the effect of maturational changes in heart rate (prolongation of the R-R interval), as regression analysis showed a strong, statistically significant relation between time to peak global systolic strain indices and R-R interval ( R 2 = 0.65, P < .0001).
2DSTE parameter | Before intervention ( n = 37) | 6-mo follow-up ( n = 37) | 3-y follow-up ( n = 36) |
---|---|---|---|
Global SL (%) | −14.7 ± 1.0 | −16.2 ± 1.6 ∗ | −17.2 ± 1.2 † |
Global SC-P (%) | −18.2 ± 2.2 | −19.4 ± 2.0 † | −20.7 ± 1.4 † |
Global SC-M (%) | −16.0 ± 1.3 | −17.7 ± 1.8 ∗ | −18.5 ± 1.6 |
Global SR-P (%) | 51.7 ± 2.5 | 53.3 ± 2.6 † | 54.8 ± 2.0 † |
Global SR-M (%) | 49.2 ± 2.5 | 51.0 ± 2.6 † | 52.5 ± 2.2 † |
T2P global SL (msec) | 374 ± 58 | 363 ± 48 | 393 ± 31 † |
T2P global SC-P (msec) | 347 ± 60 | 335 ± 52 | 366 ± 30 † |
T2P global SC-M (msec) | 363 ± 55 | 353 ± 45 | 375 ± 22 † |
T2P global SR-P (msec) | 354 ± 55 | 324 ± 37 † | 344 ± 23 † |
T2P global SR-M (msec) | 401 ± 51 | 352 ± 52 † | 353 ± 41 |
Global SrL (s −1 ) | −0.9 ± 0.1 | −1.1 ± 0.2 ∗ | −1.1 ± 0.1 |
Global SrC-P (s −1 ) | −1.4 ± 0.2 | −1.6 ± 0.2 † | −1.6 ± 0.2 |
Global SrC-M (s −1 ) | −1.0 ± 0.3 | −1.2 ± 0.3 † | −1.2 ± 0.3 |
Global SrR-P (s −1 ) | 3.1 ± 0.5 | 3.4 ± 0.4 | 3.4 ± 0.5 |
Global SrR-M (s −1 ) | 2.9 ± 0.5 | 3.1 ± 0.4 | 3.0 ± 0.6 |
∗ P < .0001 and † P < .05 compared with the same 2DSTE parameter at the prior examination by linear mixed models.

Global peak systolic strain rate in the longitudinal and circumferential directions improved significantly at intermediate follow-up compared with preintervention measurements. At 3-year follow-up, global peak systolic strain rate results were similar to those acquired at intermediate follow-up in all three directions of deformation. Figures 2A to 4B show examples of strain curves in a representative VAS patient before and after balloon valvuloplasty.



Deviation from Normal 2DSTE Reference Values
All 2DSTE results were compared with pediatric reference values to correct for possible bias caused by maturational changes. For all individual 2DSTE results of the study subjects (preintervention and at follow-up), the deviation from normal was calculated (2DSTE result of the individual patient minus mean the reference value of the 2DSTE parameter for age). The deviation from normal for all individual strain parameters was significantly ( P < .0001) reduced at intermediate follow-up. At 3-year follow-up, there was no significant further reduction in deviation from normal, and similar values as at intermediate follow-up were found ( Table 5 ). The decline in longitudinal systolic strain as well as longitudinal systolic strain rate before intervention was more pronounced in segments of the interventricular septum, especially its basal parts. Global peak systolic longitudinal strain was on average 6.8 ± 1.0% lower in the interventricular septum (7.2 ± 0.9% in the basal part) and 5.0 ± 1.2% lower in the lateral free wall compared with healthy, age-matched children ( P < .001). In contrast to longitudinal deformation, circumferential and radial strain and strain rate values were equally affected throughout the interventricular septum and LV lateral wall segments. In the individual patient with aortic stenosis under investigation, preintervention global peak systolic strain was abnormal (below the fifth percentile of normal according to previously mentioned reference values) in 97% of patients for longitudinal strain, 65% of patients for circumferential strain at the level of the papillary muscle, and 68% of patients for circumferential strain at the level of the mitral valve. Global peak systolic radial strain was abnormal at the level of the papillary muscle in 24% of the patients compared to 16% of patients at the level of the mitral valve.
2DSTE parameter | Before intervention ( n = 37) | 6-mo follow-up ( n = 37) | 3-y follow-up ( n = 36) |
---|---|---|---|
Δ global SL (%) | 5.8 ± 0.9 | 4.5 ± 1.3 ∗ | 4.4 ± 1.0 |
Δ global SC-P (%) | 3.5 ± 0.8 | 2.6 ± 1.2 † | 2.4 ± 1.3 |
Δ global SC-M (%) | 4.0 ± 1.0 | 2.5 ± 1.3 ∗ | 2.3 ± 1.2 |
Δ global SR-P (%) | −3.1 ± 1.0 | −1.8 ± 0.9 ∗ | −1.4 ± 0.5 |
Δ global SR-M (%) | −3.0 ± 1.3 | −1.3 ± 0.6 ∗ | −1.0 ± 0.4 |
Δ T2P global SL (msec) | 40 ± 8 | 22 ± 10 ∗ | 19 ± 9 |
Δ T2P global SC-P (msec) | 26 ± 9 | 9 ± 4 ∗ | 11 ± 5 |
Δ T2P global SC-M (msec) | 25 ± 7 | 11 ± 5 ∗ | 7 ± 3 |
Δ T2P global SR-P (msec) | 18 ± 4 | 8 ± 3 ∗ | 8 ± 2 |
Δ T2P global SR-M (msec) | 36 ± 13 | 15 ± 6 ∗ | −5 ± 2 † |
Δ global SrL (s −1 ) | 0.5 ± 0.1 | 0.3 ± 0.2 ∗ | 0.3 ± 0.2 |
Δ global SrC-P (s −1 ) | 0.5 ± 0.2 | 0.3 ± 0.2 ∗ | 0.3 ± 0.2 |
Δ global SrC-M (s −1 ) | 0.5 ± 0.3 | 0.3 ± 0.3 † | 0.3 ± 0.3 |
Δ global SrR-P (s −1 ) | −0.4 ± 0.5 | −0.1 ± 0.4 † | −0.1 ± 0.5 |
Δ global SrR-M (s −1 ) | −0.3 ± 0.5 | −0.1 ± 0.4 | −0.2 ± 0.6 |
∗ P < .0001 and † P < .05 compared with the same 2DSTE parameter at the prior examination by linear mixed models.
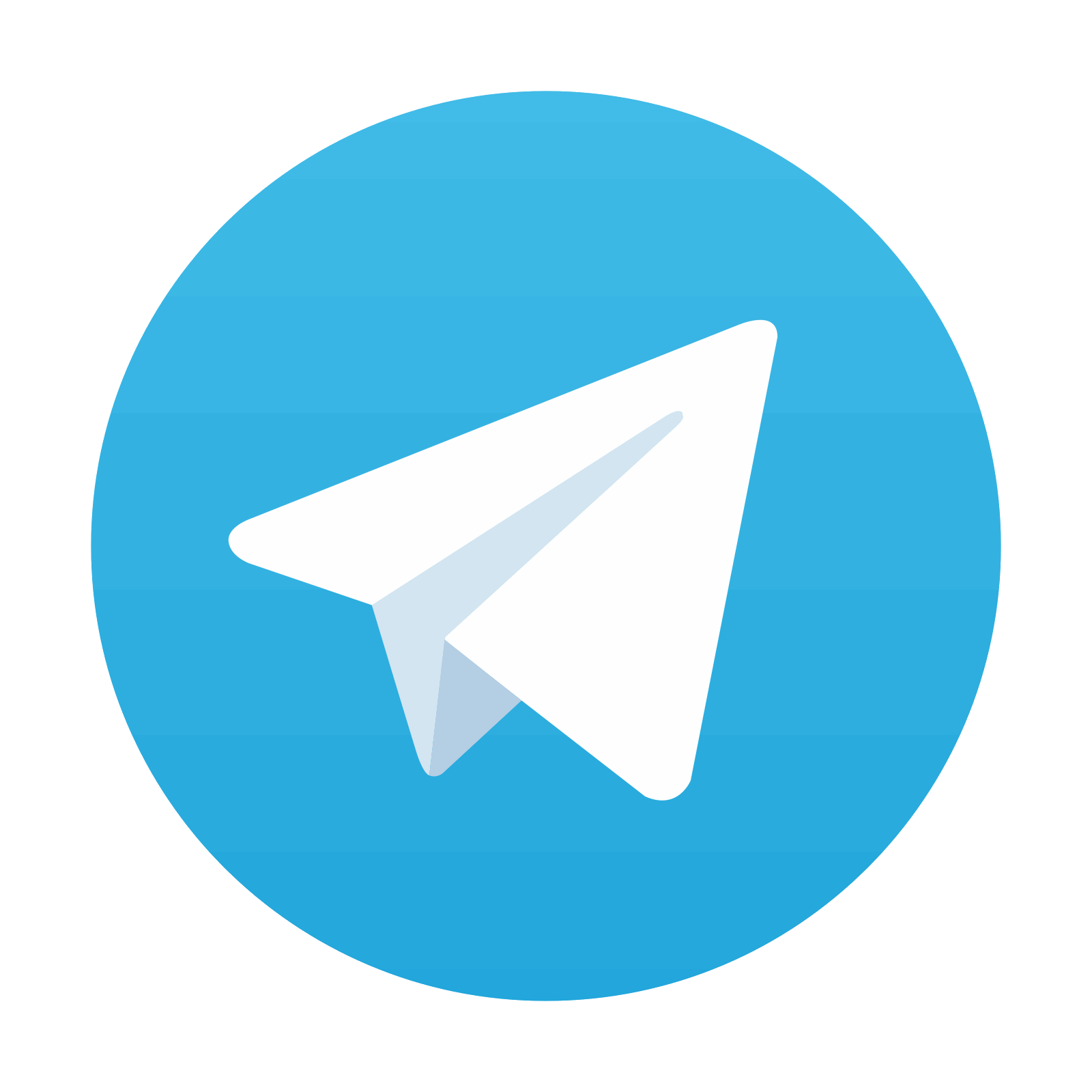
Stay updated, free articles. Join our Telegram channel

Full access? Get Clinical Tree
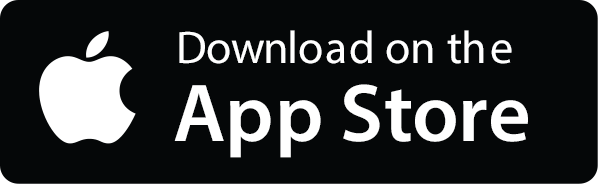
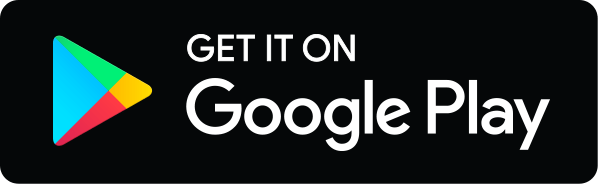