If the bradyarrhythmia does not resolve after treatment of these causes, especially in the case of an acute anterior MI, a permanent PM is indicated. Also, if rate-slowing drugs are necessary to control frequent paroxysms of SVT, a PM is indicated. Pacemakers (PMs) are designated by a three- or four-letter code: e.g., AAI, VVI or DDD. A VVI PM has a single sensing and pacing lead in the right ventricle. It paces the ventricle when it sees that the interval between two QRS complexes is longer than the back-up limit, called the lower rate interval; a limit of 1 second, for example, corresponds to a heart rate (HR) of 60 bpm. It does not see or pace the atrium, and does not make the ventricle track the atrium. In patients with sinus rhythm, VVI may lead to AV dissociation and loss of the atrial contribution to the cardiac output. VVI pacing is mainly used in chronic AF, wherein the atrium cannot be paced or tracked. It may also be an acceptable option in older sedentary patients with AV block. VVI pacing may lead to a pacemaker syndrome. This is a syndrome of fatigue, dyspnea, and pre-syncope that results from: (i) loss of the atrial contribution to the cardiac output; (ii) some of the asynchronous atrial contractions occur during ventricular systole while the AV valve is closed, leading to increased atrial pressure. Moreover, ventricular pacing may lead to retrograde P waves; those retrograde P waves are an additional cause of atrial contraction during ventricular systole and may be a major contributor to the pacemaker syndrome. DDD is a dual-chamber PM, with sensing and pacing leads placed in both the right atrium and the right ventricle. DDD pacing is indicated for sinus node disease and AV nodal disease. If the patient develops AF, DDD senses the excessive atrial activity and switches the pacing mode to VVI or DDI (mode-switch function). A biventricular pacemaker (BiV PM, also called cardiac resynchronization therapy, CRT), is a pacemaker that typically has three leads: RA lead, RV lead, and LV epicardial lead placed via the coronary sinus. In a way, it is a DDD PM with an additional LV lead. This PM is indicated for HF (functional class III or IV) with an EF ≤35% and LBBB or RV-paced rhythm that delays the LV excitation and contraction. A wide QRS, particularly LBBB, is seen in 20–30% of patients with systolic HF. LBBB is associated with dyssynchronous LV contraction, i.e., the RV contracts before the LV (interventricular conduction delay) and the septal wall contracts before the posterolateral wall (intraventricular conduction delay). This leads to ineffective systolic function, as one wall contracts while the other stretches in the opposite direction, a reduction in stroke volume, a larger LV volume in systole, and subsequently, a maladaptive LV dilatation. Typically, BiV PM is used in patients who are not bradycardic. On the other hand, when patients with HF and low EF (≤35%) require PM placement for bradycardia, and when the ventricle is expected to be frequently paced (>40%), the implantation of a BiV PM rather than a right ventricular PM seems to be the best option regardless of NYHA symptoms (class IIa recommendation). The purpose of the BiV PM is to allow simultaneous contraction of the LV septal and posterolateral walls, as well as the RV and LV, to improve cardiac function. To be beneficial, it has to track the atrial rate and pace both ventricles ~100% of the time. It cannot be in a standby mode. The AV tracking interval needs to be programmed shorter than the intrinsic PR interval to ensure ventricular pacing. AV delay is also optimized to ensure appropriate diastolic filling (a long AV delay reduces diastolic filling and leads to fusion of E–A waves on echo; a very short AV delay leads to atrial contraction near systole and A truncation). In AF, the BiV PM is programmed in VVI mode (no A tracking). The AV conduction has to be aggressively slowed so that the intrinsic QRS rate is slow, and the BiV pacing would pace the ventricles at a rate faster than the spontaneous ventricular rate. AV nodal ablation may need to be performed to ensure consistent BiV pacing in patients with AF and an indication for BiV pacing. The QRS of a BiV-initiated complex is typically narrower than the RV pacemaker-initiated complex and the baseline non-paced QRS, even though the QRS often remains wide. The morphology is not an LBBB morphology, and the QRS has at least one of the following two features: (i) prominent negativity in lead I, or (ii) prominent positivity in lead V1. If not negative, the QRS in lead I needs to have at least a q or Q wave. If the LV lead is positioned close to the LV apex, QRS may be negative in all precordial leads V1–V6, simulating RV apical pacing in those leads (Figure 14.1). Examples of pacemaker ECGs are provided in Figures 14.2–14.6. An ICD has one (V) or two (A + V) leads. In addition to antitachycardia capacity provided by the special RV lead, it has single- or dual-chamber sensing and pacing capacities (VVI or DDD). Figure 14.1 The paced QRS morphology varies according to the vector of depolarization. Analyze the QRS morphology in lead I, the inferior leads, and the precordial leads V1–V6. In RV apical pacing, the QRS is characterized by a left axis and by being negative in the inferior leads II, III, and aVF. It has an LBBB morphology, and similarly to LBBB, QRS may be negative in V1–V3 and upright in V4–V6, I, and aVL. However, the vector of depolarization may also be looking away from all precordial leads, leading to a deep negative QS in V1–V6, and may be looking away to the right, leading to a negative QRS in lead I. If the vector is looking to the right, the QRS may paradoxically be upright in leads V1 and V2, simulating LV or BiV pacing (especially if V1 is high in relation to the heart). In RVOT pacing, the depolarization looks towards the inferior leads (vertical or right axis). QRS may be positive or negative in lead I. In the precordial leads, QRS simulates an LBBB morphology and, as opposed to RV apical pacing, cannot be negative all the way from V1 through V6. In BiV pacing, the QRS: (1) is predominantly negative in lead I with a Q wave (QR or qR pattern), and (2) has a positive QRS or at least a prominent R wave in the precordial lead V1. This is different from RV pacing, which occasionally has either one of these two features. If the LV lead is close to the apex, QRS may be negative in all precordial leads V1–V6, similar to RV pacing. Figure 14.2 Ventricular pacing regularly tracking the sinus P activity (DDD PM, VAT action). The paced ventricular complexes have an LBBB morphology with QS or rS pattern that extends further to the left (V1–V6) than in a typical LBBB (in which QRS often transitions to positive in lead V4). The large Q wave in lead I is not typical of RV apical pacing but may be seen if the vector of depolarization is turned a bit rightward. BiV pacing is unlikely, because of the negative QRS in V1–V2. This is not RVOT pacing, because in RVOT pacing the depolarization vector is directed up to down, and the QRS is consequently [+] in the inferior leads. Figure 14.3 VVI pacing in a patient with no P wave (sinus arrest or subtle AF). RV lead is in the RVOT (QRS is positive in the inferior leads). Off/on failure to capture is noted (arrow, failure to capture). This is actually a temporary pacemaker. RVOT is an unstable position for a temporary pacemaker. Figure 14.4 Ventricular pacing that consistently tracks a preceding sinus P wave. This is AV sequential pacing (probable DDD mode). Note that QRS is negative in lead I and starts with a Q wave, and QRS is positive in V1: this is typical of BiV pacing. Also, PR is very short to ensure ~100% pacing, which is characteristic of BiV programming. Q or qR in lead I is rare in RV pacing, but is often present with BiV pacing (90%). If initially present with BiV pacing, the loss of Q wave in lead I is 100% predictive of loss of LV capture. Figure 14.5 Atrial flutter with variable conduction. Note that when the R–R interval increases to >1200 ms (which corresponds to 6 large boxes, or a rate of 50 bpm), V pacing kicks in at an interval of 1000 ms (60 bpm). This is called hysteresis, wherein V pacing is initiated only when the heart rate drops well below the programmed pacing rate, allowing a reduction of V pacing. The V lead has one (RV) or two coils (RV + SVC) that allow the delivery of the shock energy. The vector of shock goes between the battery can and the coil; in patients with two coils, the vector may be modified to provide superior efficacy (e.g., can-to-RV, or can + SVC-to-RV). The ICD is best placed on the left side to allow the vector of shock to be orthogonal to the heart, fully traversing the myocardium. ICD patients often do not require pacing. The pacing function is a standby function that should only be initiated at very low ventricular rates (e.g., 34–40 bpm). Harmful ventricular pacing should be prevented. The sensing function is what detects VF/VT. Typically, a certain ventricular rate is considered VT, regardless of its true origin. Many ICDs are programmed into two detection zones: VT and VF. Those two zones are differentiated by their rate (e.g., VT zone encompasses any ventricular rate of 170–220; VF zone encompasses ventricular rates >220). The ICD may have, on the other hand, SVT–VT discrimination algorithms, particularly the dual-chamber ICD, wherein the atrial lead can see the atrial activity: These discrimination algorithms are applied only for the VT zone. Any tachycardia in the VF zone is considered VF. Moreover, these discrimination algorithms may have a time out. For example, ICD may be programmed in such a way that if the tachycardia persists more than 5 minutes it is treated as VT regardless of the initial diagnosis. Figure 14.6 Prominent R wave in V1–V2 may suggest BiV pacing. However, there is no Q wave in lead I, which suggests that RV pacing is more likely (RV apical pacing by the fact that QRS is negative in the inferior leads and in V4–V6). V1 may be placed one intercostal space lower and the ECG repeated; QRS of V1 should become negative in RV apical pacing. Typically, VT is initially treated with multiple cycles of antitachycardia pacing (ATP: overdrive ventricular pacing at a cycle length ~85% the tachycardia cycle length). If these fail, the ICD delivers a shock. If the tachycardia is fast enough to fall in the programmed VF zone, ICD immediately delivers a maximal shock. ATP enters the VT reentry cycle and breaks it in many patients; however, if the excitable gap of the reentry is wide, ATP may entrain the reentry at a faster rate, and may trigger further reentries that lead to VF. In fact, the most important ICD primary prevention trial (SCD-HeFT) recommended against ATP therapy and used a single-chamber rather than dual-chamber ICD, with shock therapy for tachycardia >187 bpm.5 A VVI pacemaker is characterized by two basic intervals: (i) lower rate interval (the basic pacing rate), and (ii) ventricular refractory period, during which the pacemaker cannot sense any signal or reset its timing based on signals. Even if the lower rate interval is 1 second, the pacemaker may not initiate pacing until a 1.5 second pause occurs. In other words, the pacemaker may not start pacing until the ventricular rate falls to 40 bpm, after which it will start pacing at 60 bpm. This is the hysteresis function of a pacemaker, meant to reduce the burden of ventricular pacing. A DDD pacemaker is characterized by four basic intervals:4,6 Figure 14.7 DDD pacemaker timing intervals. AP, atrial paced event; AS, atrial sensed event; VP, ventricular paced event; VS, ventricular sensed event. AEI, atrial escape interval after any ventricular sensed or paced activity (the occurrence of an atrial sensed event aborts AEI); LRI, lower rate interval; pAVI, paced atrioventricular interval; PVARP, post-ventricular atrial refractory period; PVAB, post-ventricular atrial blanking period; sAVI, sensed atrioventricular interval; VB, ventricular blanking (post-atrial); VSP, ventricular safety pacing. Figure 14.8 Sinus/atrial tachycardia with atrial rate > upper rate. Case where TARP (total atrial refractory period) = URI (upper rate interval), which leads to 2:1 ventricular pacing pattern. Other abbreviations as in Figure 14.7. In the case of sinus tachycardia, the pacemaker senses the intrinsic sinus P waves and tracks them, leading to fast ventricular pacing. However, the pacemaker can only track the P waves up to a certain upper rate, called the upper rate limit (e.g., 500 ms = 120 bpm). If the atrial rate is faster than this upper rate, the PM will only track some of the P waves. If this upper rate limit is equal to the TARP and if the atrial rate exceeds 120 bpm, the P wave that follows the paced QRS will fall into PVARP, which means that only every other P wave gets conducted; the patient’s rate suddenly falls from 120 to 60 bpm (Figure 14.9). However, if PVARP is reduced in such a way that TARP is shorter than the upper rate interval (e.g., TARP = 400 ms, TARP rate 150 bpm), the ventricular rate will remain steady at 120 bpm even if the atrial rate exceeds 120 bpm. P waves will be conducted with progressively longer PR intervals. One P will drop every few cycles in a pseudo-Wenckebach fashion. When the atrial rate reaches 150 bpm (TARP), every other P wave gets conducted. Shortening the TARP, however, allowed a delay in onset of the 2:1 atrial conduction. PM can also be programmed to have an adaptive PVARP and AV delay that decrease with exercise, allowing a reduction in TARP and in the onset of sudden rate drop. Figure 14.9 Sinus tachycardia with pseudo-Wenckebach ventricular pacing pattern. In contrast to Figure 14.8, PVARP is short with TARP <URI. This shortened PVARP allows AS to fall outside PVARP and get tracked. AVI extends after every cycle, until one beat drops. It is a pseudo-Wenckebach rather than a true Wenckebach pattern, as the R–R interval remains steady rather than shortens. Unlike patients with a dual-chamber PM, patients with BiV often need a higher upper rate limit (e.g., 140 bpm) to ensure consistent biventricular pacing at upper rates and prevent Wenckebach from occurring, particularly since higher sinus rates are seen in decompensated HF at rest and with activity. The prolongation of the paced AV delay (Wenckebach) that occurs as the atrial rate reaches the upper rate may allow an undesirable intrinsic AV conduction. A short PVARP is necessary to allow an increase in the upper rate, and thus, a rate-adaptive PVARP that automatically decrements at higher rates may be programmed. The pacer spike has a high voltage (measured in volts), much higher than the voltage of the intrinsic cardiac activity (millivolts). If the ventricular lead senses the atrial spike, ventricular pacing will be inhibited after atrial pacing. Therefore, atrial pacing automatically initiates a short interval called the ventricular blanking period, during which the ventricular sensing is totally blind to any signal. This only occurs after a paced atrial event (not an intrinsic atrial event). A ventricular activity that is detected early after the atrial activity (<110 ms) but beyond the ventricular blanking period may be a far-field artifact or a PVC. The PM tends to immediately pace over it. As opposed to the blanking period, this activity cannot be ignored; in fact, if it turns out to be a PVC that is ignored, the ventricular pacing that follows the end of the AV delay would fall on the PVC’s T wave and trigger VF. Conversely, it cannot be automatically considered PVC and allowed to reset ventricular pacing, as there is a high rate of atrial output artifacts within this zone (called afterpotentials). That is why, during this interval, any sensed activity by the V channel triggers immediate V pacing (ventricular safety pacing). On ECG, a spike in the middle of a PVC with a short PR interval implies safety pacing rather than undersensing. The PVARP is meant to prevent the PM from tracking retrograde or premature P waves. The PM sees the atrial activity during most of the PVARP and counts it for mode-switch purposes, but does not track it with V pacing. However, the PVARP, whether initiated by a sensed or a paced QRS, contains a small interval called PVAB (post-ventricular atrial blanking), where the atrial channel does not even see any electrical activity. In fact, in order to detect the small electrograms of atrial tachyarrhythmias, atrial sensitivity is programmed high, and may erroneously detect ventricular activity. PVAB prevents it from sensing ventricular activity and counting it as a P wave. PVAB and PVARP are initiated after both paced and intrinsic ventricular events (the atrial channel may sense the ventricular pacing spike but also the QRS ventricular complex, which is much louder than the atrial complex). Overcounting P waves, including those falling in a refractory period, leads to a harmful mode switch (to VVI). In a DDD pacemaker, the upper rate limit (or maximal tracking rate) designates the maximal ventricular pacing in response to an intrinsic/sensed atrial rate (e.g., tracking intrinsic sinus tachycardia in a patient with complete AV block). Figure 14.10 Difference between maximal tracking rate, mode-switch rate, and maximal sensor rate. The mode-switch rate is the maximal intrinsic/sensed atrial rate at which the pacemaker continues to track some of the atrial rate and pace the ventricle. Beyond the mode-switch rate, the pacemaker calls the atrial rhythm atrial tachyarrhythmia and reverts to a non-tracking mode (VVI-R). The mode-switch rate is higher, sometimes much higher, than the upper rate limit (e.g., the PM remains in DDD mode up to 170 bpm and continues to track some of the atrial rate, pacing the ventricle at the upper rate of 120 bpm). In a DDD-R pacemaker, the upper sensor rate is the maximal paced atrial rate of a sensor-driven pacemaker. This is different from the maximal tracking rate, which refers to the tracking of intrinsic atrial activity.* Magnet mode is the pacemaker behavior when a magnet is applied over it. A magnet closes a reed switch in the generator and makes it convert to an asynchronous ventricular and/or dual pacing mode (VOO or DOO). This mode is useful as a means of breaking PMT (pacemaker-mediated tachycardia). Furthermore, the magnet rate is programmed in a way that indicates the status of the battery (e.g., a lower magnet rate indicates that the battery is close to end of life). A magnet is part of the modem used in pacemaker transtelephonic follow-up. A magnet may be present in programmer heads, and this temporarily closes the reed switch, ending a PMT. However, after the programmer is turned on, the magnet effect is functional for only a short period of time. In contrast to a PM, the application of a magnet on an ICD does not affect the pacing mode or the sensing function, i.e., the DDD pacing function of an ICD keeps its DDD pacing function. It does not affect arrhythmia detection either. It only inhibits antitachycardia therapy and shock. Leads detect electrical activity between two electrodes (anode and cathode) and deliver the output current between these two electrodes. A unipolar lead has one electrode at the distal tip, the metal of the can constituting the other electrode. The pacing current flows through a large circuit and may cause stimulation of the chest muscle and a large pacing spike on the ECG. Also, the sensing current is large and is prone to sensing myopotentials. A bipolar lead has two electrodes on its distal end (ring and tip electrodes) creating a smaller sensing and pacing circuit. However, it has to contain two wires to conduct signals to and from both electrodes and needs to be slightly thicker. If the ring wire breaks, the lead converts to a unipolar mode. A bipolar defibrillator lead contains two pacing electrodes and one or two defibrillator coils: one at the distal end (RV coil) ± one more proximal (SVC coil). After VT is sensed, the defibrillation circuit occurs between the can, which functions as an electrode, and the RV coil. Various configurations are possible (e.g., energy can be delivered between the can and the SVC coil as one pole, and the RV coil as second pole). Generator placement on the left side allows delivery of energy that is orthogonal to the heart and traverses it more effectively. The current that flows through the lead and is delivered to the myocardium depends on both the battery voltage delivered and the lead resistance/impedance. In fact: current (mA) = voltage/resistance. The battery voltage is typically 2.8 volts. The lead itself needs to have a high impedance to reduce the current that is being drained. The lead tip needs to have a low resistance to allow electrical flow to the myocardium. Upon new PM implantation, the pacing threshold rises over the first few weeks then goes down to a chronic baseline 8 weeks later. This is due to the initial inflammation at the lead tip and mandates programming of high pacing thresholds initially, followed by reprogramming 8 weeks later. A steroid-eluting lead prevents this phenomenon and allows chronically lower pacing thresholds. Pacing leads are insulated, meaning they are covered with material that does not conduct electricity and prevents loss of current from the lead, allowing current to travel all the way to the myocardium. Figure 14.11 Various lead configurations for pacemakers (first two images) and ICD (third image). Figure 14.12 Example of a “quick look” or “summary” screen of pacemaker/ICD interrogation. On the right, the three most important icons are indicated (data, parameters, and tests). On top of the screen, three types of live recordings are shown: (1) surface ECG (uppermost recording), (2) intracardiac electrograms (EGM), which capture the actual signals occurring in each cardiac chamber (in this case, ventricular EGM is shown), and (3) marker representation, which indicates how the pacemaker sees and interprets these signals. AS and VS are sensed intrinsic atrial and ventricular activities, while AP and VP are paced atrial and ventricular activities. The pacemaker may interpret a signal as VS on the marker channel, while in fact it is a T wave oversensing, false signal, or far-field atrial sensing. Looking at the EGMs allows one to understand what the issue is; looking at the markers allows one to see how the pacemaker interprets or misinterprets signals. At the bottom left, capture thresholds, impedance, and the sensed amplitudes of A and V are shown. Under summary/quick look/diagnostics (Figure 14.12), look at the following: Check impedances and impedance trends of A and V leads. Any significant change (>200 ohms) in lead impedance can indicate lead failure. Testing is performed in DDD mode. In patients with intrinsic V activation, the loss of atrial capture is seen as a loss of ventricular sensed events; make sure the AV delay is long enough to allow intrinsic V activation for this test (if possible). Sensitivity is the programmed wall below which the PM cannot detect electrical activity. The higher the wall is in millivolts, the less sensitive the PM is, and the more likely it is to dismiss noise but also, on occasions, native complexes. To test sensing of the V channel, set the PM in VVI mode and reduce the pacing rate below the patient’s intrinsic rate (if the patient has a tolerable intrinsic rate). Start at a low millivolt sensitivity then slowly increase until the PM stops sensing intrinsic activity and starts pacing at the low set rate. Sensitivity should be set below that value. Note that testing for sensing involves maneuvers opposite to those used in testing for capture (low rate and starting with the lowest setting). In addition, current PMs measure the sensed amplitude of the intrinsic A and V activities. The sensed RV amplitude is typically >5 mV, while the sensed A amplitude is typically >2 mV. The sensitivity should be a half or a third this value. A very low sensitivity may detect myopotential noise or oversense T wave. Ventricular undersensing is a situation in which the pacemaker does not see intrinsic QRS and thus does not get inhibited by it. This leads to overpacing, with pacing spikes occurring regularly, including early after intrinsic QRS complexes and unrelated to them. Causes of undersensing: Undersensing may also be seen in atrial flutter, where every other P wave falls in the blanking portion of PVARP and is not sensed. This precludes mode switching and allows tracking of the sensed P waves (e.g., atrial rate of 280 bpm, every other P is sensed and tracked at a fast rate of 140 bpm). It is treated by shortening the blanking period. Ventricular oversensing is a situation in which the pacemaker senses electrical activity that is not QRS and considers it V, therefore inhibiting the ventricular output. It does not pace the ventricle when it should. This leads to underpacing and a paucity of pacer spikes. Causes of oversensing:
14
Permanent Pacemaker and Implantable Cardioverter Defibrillator
I. Indications for permanent pacemaker implantation1
II. Types of cardiac rhythm devices
A. VVI = ventricular demand pacing
B. DDD = AAI + VAT + VVI
Notes
C. Biventricular pacemaker
D. Implantable cardioverter defibrillator (ICD)
III. Pacemaker intervals
A. VVI
B. DDD pacemaker’s four basic intervals (Figure 14.7)
C. Two other intervals are derived from the four basic intervals
D. Upper rate behavior (see Figures 14.8, 14.9)
E. Prevention of crosstalk4,6
Atrial-initiated ventricular blanking period
Ventricular-initiated atrial refractory period
F. Upper rate intervals (see Figure 14.10)
G. Magnet mode
IV. Leads (see Figure 14.11)
V. Systematic PM/ICD interrogation using the programmer
A. Summary screen
B. Lead impedance
C. Capture testing
D. Sensing assessment
VI. Pacemaker troubleshooting
A. Undersensing or failure to sense
B. Oversensing
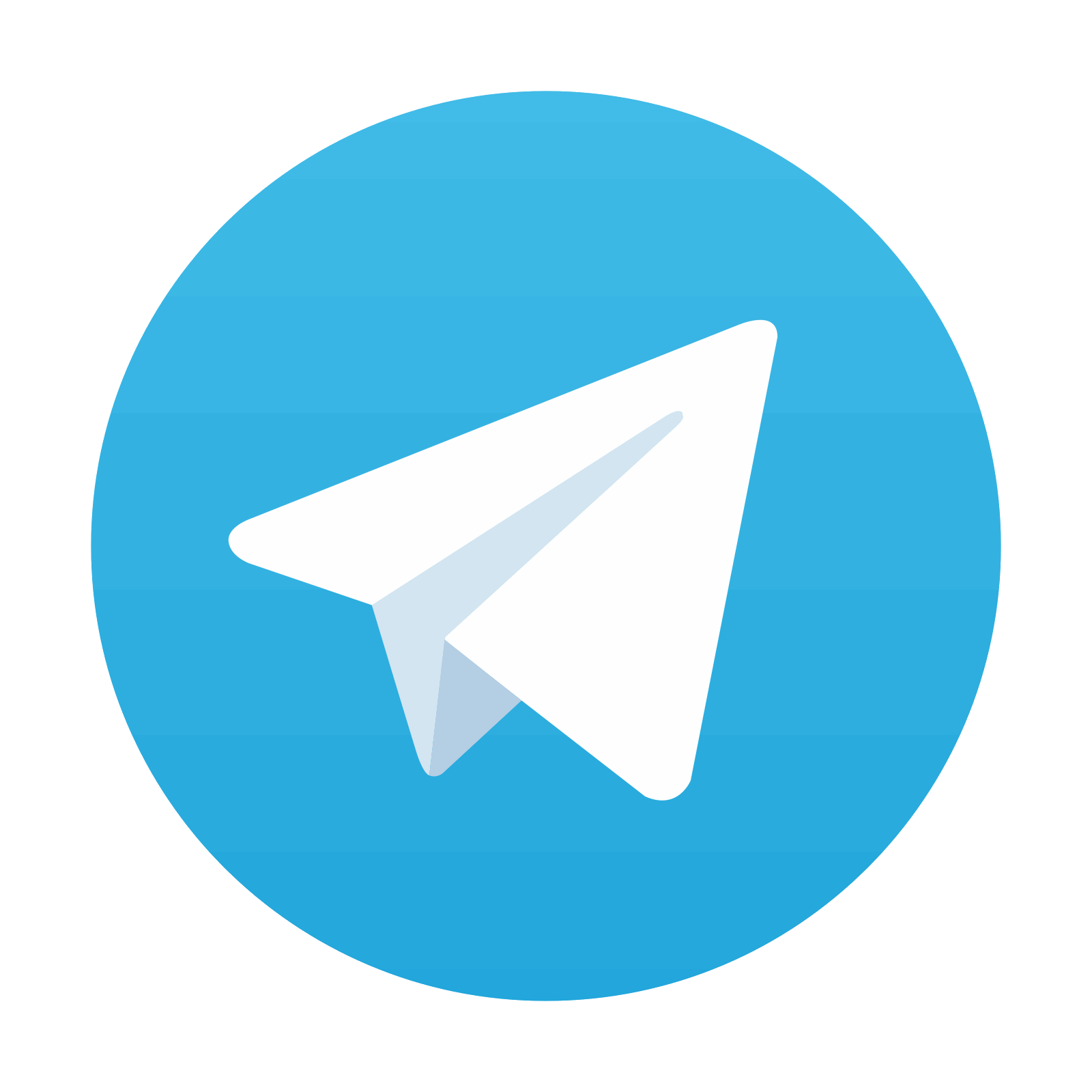
Stay updated, free articles. Join our Telegram channel

Full access? Get Clinical Tree
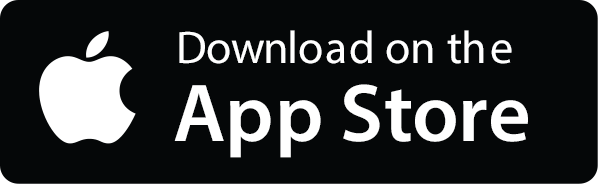
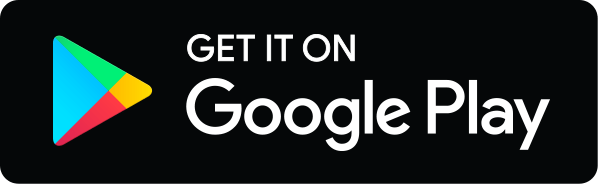