Fig. 20.1
Increased cardiac output is observed during pregnancy. Schematic highlighting the changes in cardiac output and systemic vascular resistance throughout the course of pregnancy
The expansion in red blood cell mass, stimulated by an increase in erythropoietin, begins in the third month of gestation. A marked increase in blood volume occurs during the second trimester and a slight increase through the third trimester [4]. The extent of change in red blood cell mass is dependent on whether the mother is taking iron supplementation. With iron supplementation, the red blood cell mass increases 20–30 % (250–450 ml); with no iron supplementation, the red blood cell mass increases only 15–20 % [5]. This physiological anemia of pregnancy is due to the disproportionate increase in plasma volume compared to red blood cell mass. The decrease in blood viscosity is a protective response that reduces resistance to blood flow through the placenta and thereby reduces the workload of the maternal heart. The expansion of blood volume to 50 % above the nonpregnant state helps protect the mother in the event of hemorrhage at the time of delivery. Vaginal delivery is associated with a 500 ml blood loss for a single fetus and upwards of 1000 ml for twins [4]. Immediately after delivery, a reverse transfusion of 500 mL takes place from the uteroplacental organs or unit into the maternal circulation. Maternal red blood cell mass and plasma volume gradually return to normal levels during the first 2 months after delivery. The hematocrit begins to increase within 3 days following delivery.
Numerous hemodynamic changes associated with pregnancy occur, including an increase in cardiac output, a decrease in vascular resistance, and a reduction in systolic and diastolic blood pressures (◘ Fig. 20.1) [6]. Pregnancy is associated with an increase in cardiac output of 30–50 %, much of which occurs during the first 2 months of pregnancy [7]. The increase in plasma volume results in a rightward shift on the Frank-Starling curve, with an increase in preload and greater stroke volume. The decline in systemic vascular resistance leads to a decrease in afterload. The increase in preload, the decrease in afterload, and the increase in basal heart rate of 15–20 bpm all result in an increase in cardiac output [8].
Augmentation of stroke volume is a greater contributor to cardiac output early in pregnancy, whereas an increase in heart rate accounts for the augmentation in cardiac output during the later stages of pregnancy. Importantly, left ventricular ejection fraction (LVEF) does not change significantly during pregnancy, and, thus, a reduction in ejection volume is pathologic. The reduction in systemic vascular resistance is due to the low resistance of the uteroplacental unit and from general vasodilation [9]. Potential mechanisms resulting in vasodilation include a decreased response to pressors (angiotensin II and norepinephrine) as well as increased endothelial prostacyclin [10], increased nitric oxide [11], and increased arterial compliance (◘ Fig. 20.2) [12]. The decrease in systemic vascular resistance is associated with a reduction in both systolic and diastolic pressures [9]. In normotensive women, the systolic and diastolic pressures decline until the 21st week of pregnancy (about 5–10 mmHg below baseline) and gradually return to the baseline level during the third trimester [13].


Fig. 20.2
Cardiovascular physiological responses to pregnancy. Table outlining changes in blood volume, hemodynamics, and coagulation during pregnancy
Central venous pressure does not change during pregnancy because the increased plasma volume can be accommodated by the low-resistance vascular circuit [14]. Similarly, the pulmonary arterial and pulmonary capillary wedge pressures are not significantly impacted, owing to the fall in pulmonary vascular resistance. Maternal cardiac output depends on posture. The cardiac output is greater in the left lateral decubitus position and decreases as much as 25–30 % in the supine position. The changes in cardiac output are related to changes in preload, which can be impacted whether the uteroplacental unit is compressing the inferior vena cava. The supine hypotensive syndrome is associated with a decrease in pulse pressure and can produce a physiologic reflex tachycardia. Cardiac output is not equally distributed among the organs. Uterine blood flow increases from 50 to 60 ml during the first trimester and to 450–750 ml at term. Blood flow is also preferentially directed to the kidneys for removing maternal and fetal waste and to the skin for facilitating maternal temperature control.
Pregnancy is associated with vascular changes and changes in coagulation factors. Vascular changes include fragmentation of reticular fibers, a decrease in acid mucopolysaccharides, loss of normal corrugation of elastic fibers, and hypertrophy and hyperplasia of smooth muscle cells [15]. Reports of both aortic dissection and coronary dissection are more prevalent during gestation than during the nonpregnant state. While pregnancy is considered a hypercoagulable state, it represents the net effect of competing processes. Favoring thrombolysis is a resistance to activated protein C and a reduction in protein S [16]. Favoring an increase in coagulation is the increase in multiple factors—I, II, VII, VIII, X, and XII—and an increase in inhibitors of fibrinolysis (PAI-1 and PAI-2) (◘ Fig. 20.2) [17, 18]. While the hypercoagulable state is a physiological response and is protective in the setting of maternal hemorrhage, it poses an increased risk of venous thrombosis (deep venous thrombosis of lower extremities and pulmonary embolism).
Labor and delivery produce additional physiological adaptive hemodynamic changes [19, 20]. Uterine contractions result in the transfer of blood from the sinusoids into the maternal systemic circulation. An increase in the maternal cardiac output results from an increase in both stroke volume and pulse [21]. The cardiac output increases 15 % during the early phase of labor and 25 % during the active phase of labor. Cardiac output increases 50 % in the second stage of labor when the woman is actively pushing. Following delivery, the uterus involutes, resulting in additional autotransfusion and up to an 80 % increase in cardiac output above the prelabor value. The augmentation in cardiac output and stroke volume remain markedly elevated during the hours after delivery. Cardiac output and systemic vascular resistance return to baseline over the next 3 months.
Uterine contractions result in a 15–25 % increase in systolic and a 10–15 % increase in diastolic blood pressure. The blood pressure and heart rate changes occur during the second stage of labor, when the woman is pushing, similar to the changes that occur during the Valsalva maneuver. At the onset of pushing, a brief increase in left ventricular output takes place. The straining phase that follows is associated with a decrease in venous return, right and left ventricular volumes, stroke volume, mean arterial pressure, and pulse pressure. A reflex tachycardia results. Following the release of the strain, the left ventricular volume further declines. Completion of the strain brings an increase in stroke volume, an increase in arterial pressure, and a decrease in the heart rate.
The cardiovascular symptoms and physical findings associated with pregnancy are noteworthy. Innocent hyperpnea (breathlessness), reduced exercise tolerance, bruisability, basal rales that clear with coughing, and edema are common and not pathological. The arterial pulse is characterized by a rapid rise and collapse starting in the first trimester and resembles a water hammer pulse. While the jugular venous pressure remains normal, the x and y descents become more prominent during the second trimester. As the uterus enlarges, the heart is shifted anteriorly and laterally, and the apical impulse shifts to the fourth intercostal space in the midclavicular line. The heart rate and blood pressure increase. The heart sounds augment, and S1 splits widely. Similarly, S2 splits widely during the latter stage of pregnancy (◘ Fig. 20.3). While an S3 is frequent, an S4 is pathologic. A systolic ejection murmur, moderate in intensity, can be auscultated over both the tricuspid and pulmonic and tricuspid areas. The mammary soufflé is unique to pregnancy and is auscultated over the breasts in the third trimester—and can either be systolic or continuous.


Fig. 20.3
Causes of peripartum cardiomyopathy. Schematic highlighting the most common causes of peripartum cardiomyopathy, including autoimmune issues, viral infections, inflammation, oxidative stress, and angiogenic perturbations
Definition of Peripartum Cardiomyopathy
Peripartum cardiomyopathy has been recognized as a clinical syndrome since the 1930s. Various definitions have been proposed by national organizations, both in the United States and Europe [22–25]. In 2000, a National Heart, Lung, and Blood Institute (NHLBI) workshop set forth the following diagnostic criteria for peripartum cardiomyopathy:
The development of heart failure (HF) in the last month of pregnancy or within 5 months postpartum
The absence of another identifiable cause of HF
The absence of recognizable heart disease prior to the last month of pregnancy
Left ventricular systolic dysfunction demonstrated by classical echocardiography criteria (LVEF < 45 %, fractional shortening <30 %, or both, with or without an LV end-diastolic dimension >2.7 cm/m2 body surface area) [22].
In 2010, the European Society of Cardiology (ESC) Working Group on Peripartum Cardiology expanded the definition of peripartum cardiomyopathy by describing it as an idiopathic cardiomyopathy with the following features:
The development of heart failure toward the end of pregnancy or in the months following delivery
Absence of another identifiable cause for heart failure
LVEF < 45 %, with either a dilated or normal-dimension left ventricle [25]
Importantly, the definition of peripartum cardiomyopathy excludes heart failure that occurs during the early stages of pregnancy. Pregnancy-associated cardiomyopathy (PACM) has been used to describe a dilated cardiomyopathy (DCM) occurring during the earlier stages of pregnancy [26].
Incidence and Risk Factors of Peripartum Cardiomyopathy
The incidence of peripartum cardiomyopathy varies widely around the world. Epidemiologic studies published since 2010 estimate the incidence of peripartum cardiomyopathy in the United States to range from 1:1141 to 1:4350 live births [27–32]. Peripartum cardiomyopathy is more frequent, with an incidence ranging from 1:300 in Haiti to 1:1000 in South Africa [33, 34]. The epidemiologic variance has been attributed to a wide variety of factors including hypertension and dietary intake. The Nigerian tradition of ingesting kanwa (dried lake salt) while lying on heated mud beds twice a day for 40 days during pregnancy is thought to be responsible for the strikingly high incidence of peripartum cardiomyopathy in that country.
About 45 % of cases worldwide are identified within 1 week of delivery and 75 % of cases within 1 month. A retrospective survey (2004–2011) of nationwide inpatient hospitalizations found the overall incidence of peripartum cardiomyopathy to be 10.3 per 10,000 (or 1 in 968) live births [35]. Over that 8-year period, the incidence increased from 8.5 to 11.8 per 10,000 live births (P trend < 0.001). The major adverse event rate was 13.5 %. Researchers observed an increase in cardiogenic shock from 1.0 % in 2004 to 4.0 % in 2011 (P trend < 0.001) and in mechanical circulatory support (P trend < 0.05). In-hospital mortality averaged 1.3 % per year with a statistically insignificant increase from 0.7 % in 2004 to 1.8 % in 2011.
Various risk factors have been identified for peripartum cardiomyopathy:
Maternal age > 30 years: Peripartum cardiomyopathy has been reported in the United States in women with a mean age ranging from 27 to 33 [26, 28, 29, 31, 32, 36].
African race: In epidemiologic studies, Africans have represented anywhere from 19 to 93 % of peripartum cardiomyopathy patients. In most of the studies, it was difficult to distinguish the racial makeup from the geographic source of patients. In a case–control study, Gentry et al. found African-American women had a 15.7-fold higher relative risk of peripartum cardiomyopathy than non-African-Americans [26, 28, 29, 31, 32, 36, 37].
Hypertension: The vast majority of studies have cited hypertension as a risk factor for peripartum cardiomyopathy, with an incidence varying from 15 to 68 % (mean 23 %) [26, 29, 31, 32, 38, 39]. This is in contrast to the 8 % incidence of hypertension in pregnancy in the absence of peripartum cardiomyopathy [40]. The diagnosis of hypertension can often cloud the diagnosis of peripartum cardiomyopathy because the presenting symptoms of heart failure can be attributed to either hypertension, preeclampsia, or eclampsia. However, in young women, hypertension is unlikely to produce systolic dysfunction.
Multiparity: Early data suggested multiparity was a risk factor for peripartum cardiomyopathy [36]. Recent studies have found peripartum cardiomyopathy to be associated with the first or second pregnancy in the majority of cases [9, 16, 18, 41]. Pregnancy with multiple fetuses: The incidence of multiple births in the United States is estimated to be 3 % [42]. Epidemiologic studies of peripartum cardiomyopathy have observed multiple births in 7–14.5 % of pregnancies [9, 15, 18, 19, 41].
Maternal cocaine use: In a small case report series, cocaine use during pregnancy was associated with peripartum cardiomyopathy [43].
Oral tocolytic therapy : Mendelson reported an association between chronic terbutaline therapy and peripartum cardiomyopathy. Among 15 gravidas who had peripartum heart failure, four had received terbutaline tocolysis for at least 4 weeks. All four of these patients subsequently recovered LV function [43].
Etiology of Peripartum Cardiomyopathy
The etiology of peripartum cardiomyopathy remains poorly defined. Cardiovascular risk factors (hypertension, diabetes, and smoking) as well as pregnancy-related factors (maternal age, number of pregnancies, number of fetuses, medications, and malnutrition) have been implicated. The pathogenesis, like the risk factors, is not well understood. Various mechanisms have been postulated including increased oxidative stress, cleavage of prolactin to an angiostatic N-terminal 16-kDA prolactin fragment, and impaired vascular endothelial growth factor (VEGF) signaling, resulting from upregulation of soluble forms like tyrosine kinase 1 (sFLT1) which may all be contributing factors for peripartum cardiomyopathy (◘ Fig. 20.3).
Oxidative stress and prolactin: Oxidative stress reflected by increased serum levels of oxidized low-density lipoprotein (LDL ) has been implicated in the pathophysiology of peripartum cardiomyopathy. Oxidative stress activates cathepsin D in cardiomyocytes, and cathepsin D cleaves prolactin into an angiostatic 16-kDa proapoptotic subfragment. Mice with a knockout in the cardiac tissue-specific signal transducer and activator of transcription 3 (STAT3) have increased cleavage of prolactin and develop peripartum cardiomyopathy [44]. The 16-kDa prolactin fragment inhibits endothelial cell proliferation and migration, induces endothelial cell apoptosis, disrupts capillary structures, promotes vasoconstriction, and impairs cardiomyocyte function (◘ Fig. 20.4) [45]. The 16-kDa prolactin isoform also promotes microRNA-146a expression in endothelial cells and results in anti-angiogenic effects (◘ Fig. 20.4). Increased levels of microRNA-146a have been found in women with peripartum cardiomyopathy [45]. Increased levels of soluble death receptor sFas/Apo-1, a proapoptotic serum marker, have also been identified in women with peripartum cardiomyopathy [46]. When bromocriptine, a dopamine D2 receptor agonist that inhibits lactation, was administered to knockout STAT3 mice, peripartum cardiomyopathy could be prevented [44]. This observation has formed the basis for early studies of bromocriptine and may offer a treatment strategy for women who develop peripartum cardiomyopathy (◘ Fig. 20.4) [47–51].


Fig. 20.4
Bromocriptine as a therapy for peripartum cardiomyopathy. Schematic highlighting the release and functional impact of prolactin. Blockade of prolactin with the dopamine-2D agonist bromocriptine has been shown to prevent the onset of cardiomyopathy in mice and a small clinical cohort of women at high risk for cardiomyopathy. The women had a documented peripartum cardiomyopathy in a previous pregnancy. Note that increased serum levels of 16-kDa prolactin and increased cathepsin D activity have been shown to be associated with peripartum cardiomyopathy. Bromocriptine eliminates the 16-kDa prolactin form, which has a number of deleterious effects on the endothelium and the myocardium
Inflammation: Evidence suggests that inflammation plays a role in the development of peripartum cardiomyopathy. Women afflicted with peripartum cardiomyopathy have higher serum levels of the soluble death receptor sFas/Apo-1, C-reactive protein, interferon gamma (IFN-g), tumor necrosis factor, and interleukin 6 (IL-6) [44, 46, 52, 53]. Serum levels of Fas/Apo-1 and C-reactive protein have been shown to correlate with the severity of peripartum cardiomyopathy [46]. Pentoxifylline , an anti-inflammatory drug, was associated with a clinical benefit in a single nonrandomized study of 58 women with peripartum cardiomyopathy [54].
Angiogenic imbalance : Cardiac angiogenic imbalance has been implicated in the development of peripartum cardiomyopathy. Mice that lack cardiac peroxisome proliferator-activated receptor-gamma coactivator (PGC)-1alpha , a powerful regulator of angiogenesis, develop profound PPCM [55]. Importantly, the PPCM is entirely rescued by pro-angiogenic therapies (i.e., VEGF). In humans, the placenta in late gestation secretes soluble FLT1, an inhibitor of VEGF, and this is associated with subclinical cardiac dysfunction. Preeclampsia and multiple previous pregnancies are associated with excess anti-angiogenic signaling and abnormally high levels of sFLT1, which can be identified in the serum of women with peripartum cardiomyopathy.
Autoimmune response : The autoimmune system may contribute to the pathophysiology of peripartum syndrome. Fetal cells can be found in maternal peripheral blood, and the influx of fetal cells into the maternal circulation increases during parturition [56, 57]. Potentially, these cells could migrate to the maternal heart and trigger an autoimmune response leading to cardiac dysfunction. Women with postpartum cardiomyopathy have increased serum levels of autoantibodies to cardiac tissue proteins [58]. Studies have identified autoantibodies against myosin heavy chain, adenine nucleotide translocator, and branched-chain alpha-ketoacid dehydrogenase [59, 60]. A study of 39 Nigerian women with peripartum cardiomyopathy compared to controls failed to show a difference in serum immunoglobulins, cardiac muscle antibodies, and circulating immune complexes [61]. Further research is warranted to determine whether fetal antigens are promoting an autoimmune response in the maternal heart.
Viruses and myocarditis: The extent to which viruses and myocarditis may contribute to peripartum cardiomyopathy remains controversial. The first case report (n = 3) of myocarditis among patients with peripartum cardiomyopathy was reported by Melvin et al. in 1982 [62]. Endomyocardial biopsies from five of 11 Nairobi women with peripartum cardiomyopathy found “healing myocarditis”—mild inflammatory cell infiltration within the myocardium with foci of necrosis and variable amounts of hypertrophy and fibrosis [63]. In a study of 26 patients with peripartum cardiomyopathy, endomyocardial biopsy revealed viral genomes (human parvovirus B19, Epstein-Barr virus , and human cytomegalovirus) in eight (30.7 %) individuals associated with interstitial inflammation [64]. Midei investigated 26 consecutive patients with peripartum cardiomyopathy and found myocarditis in 14 (78 %) of the patients. Ten of these patients were treated with immunosuppressive therapy with a 90 % recovery rate [65].
Genetic Association of Peripartum Cardiomyopathy
Efforts to establish a genetic susceptibility to peripartum cardiomyopathy have been hampered by the low frequency of this condition. In one of the first reports, Pierce et al. identified 3 of 17 probands with peripartum cardiomyopathy who had a family history of this condition [66]. Several small case reports subsequently identified peripartum cardiomyopathy in 2 or 3 first-degree relatives [50, 67, 68]. The presence of a dilated cardiomyopathy in other male relatives diminished the argument for genetic susceptibility. Recent family studies indicated that selected cases of peripartum cardiomyopathy may represent a form of familial dilated cardiomyopathy [69, 70]. van Spaendonck-Zwarts et al. reviewed their database of 90 families with dilated cardiomyopathy and found peripartum cardiomyopathy in 6 % of the sample [70]. They next performed cardiovascular testing of first-degree relatives of three peripartum cardiomyopathy patients who failed to recover and found dilated cardiomyopathy in all three families. Genetic analyses revealed a mutation (c.149A_G, p.Gln50Arg) in the gene encoding cardiac troponin C (TNNC1) segregating with disease in a DCM family with a member having PPCM. In another family-based genetic study, Morales et al. investigated 4110 women from 520 pedigrees in the Familial Dilated Cardiomyopathy Research Project database and found 45 cases of peripartum cardiomyopathy or pregnancy-associated cardiomyopathy. Evidence of familial clustering with dilated cardiomyopathy was observed in 23 unrelated cases. Nineteen of these cases were resequenced for known dilated cardiomyopathy genes and six carried mutations. These studies suggest a genetic association between peripartum cardiomyopathy and dilated cardiomyopathy.
Clinical Presentation of Peripartum Cardiomyopathy
The diagnosis of peripartum cardiomyopathy is based on the presentation of heart failure toward the end of pregnancy or in the first 5 months following delivery in the absence of identifiable etiology and in the setting of reduced left ventricular systolic function (LVEF < 45 %). The clinical presentation of peripartum cardiomyopathy can be insidious in onset or acute, and the New York Heart Association (NYHA) classification can vary based on the reduction in LVEF. Typical symptoms include dyspnea, cough, and fatigue. Other common symptoms include lower extremity edema, orthopnea, paroxysmal nocturnal dyspnea, palpitations, and dizziness. Less common symptoms include nocturia, right-upper-quadrant pain, chest pain, postural hypotension, and syncope. Frequently, the symptoms of peripartum cardiomyopathy are confused with other conditions or diseases and lead to a delay in diagnosis [71].
Physical exam findings are similar to those observed in the setting of congestive heart failure. The patient appears tachypneic and tachycardic; blood pressure may vary based on volume status and the severity of cardiac dysfunction. The jugular venous pressure is elevated, a right ventricular heave can be palpated, the apical pulse is displaced inferolaterally, and an S3 or summation gallop may be appreciated. Murmurs of tricuspid and mitral regurgitation can be auscultated. Pulmonary rales are present, hepatojugular venous reflex can be noted, extremities are cool to palpation, and lower extremity edema is evident.
The electrocardiographic findings are nonspecific. Frequently, sinus tachycardia, supraventricular tachycardia (SVT, atrial fibrillation or atrial flutter), and nonspecific ST and T wave changes can be observed [33, 72]. Other possible pathological findings include LV hypertrophy, left atrial enlargement, and left bundle branch block. Chest X-ray findings include cardiomegaly, pulmonary venous congestion, frank pulmonary edema, and pleural effusions [32]. B-type natriuretic peptide (BNP) and N-terminal pro-BNP (NT-proBNP) are elevated as a result of increased left ventricular diastolic dysfunction [52]. Forster et al. observed markedly elevated mean NT-BNP levels in 36 peripartum cardiomyopathy patients compared to 21 controls (1727 vs. 339, P < 0.0001) [52].
Cardiovascular imaging is essential to establish the diagnosis of peripartum cardiomyopathy. Echocardiography has been used at baseline to define the cardiomyopathy and serially to determine patient prognosis [31, 73–75]. The echocardiographic criteria for idiopathic dilated cardiomyopathy set forth by an NHLBI-sponsored workshop in 1992 included a left ventricular ejection fraction less than 45 % or M-mode fractional shortening less than 30 %, or both, with a left ventricular end-diastolic dimension greater than 2.7 cm/m2 [75]. The American Society of Echocardiography endorses the biplane method of disks to calculate both left ventricular volumes and ejection fraction [76]. Endocardial borders are traced using the apical four-chamber and two-chamber views (oriented at 90 = degree angles with similar long axis dimensions) at end-diastole and end-systole. These echocardiographic features were incorporated into the subsequent definitions of peripartum cardiomyopathy [22, 74]. The degree of left ventricular systolic function and dilation may vary from mild to severe and may be accompanied by right ventricular systolic dysfunction and dilation as well. Additional echocardiographic findings include moderate to severe mitral regurgitation and tricuspid regurgitation, increased pulmonary artery pressures, pulmonary regurgitation, and left ventricular thrombus. Serial echocardiographic evaluations following the baseline study—prior to discharge, 6 weeks, 6 months, and annually—for both prognostic assessment and to assess response to pharmacologic therapy [25]. In one study of 32 peripartum cardiomyopathy patients, a fractional shortening value less than 20 % and a left ventricular end-diastolic dimension 6 cm or greater at the time of diagnosis was associated with a more than threefold higher risk for persistent left ventricular dysfunction [31].
Cardiac magnetic resonance imaging (MRI ) has become a valuable tool for assessing cardiomyopathy. Cardiac MRI is more accurate than echocardiography in assessing chamber volumes and ventricular function, as well as LV thrombus. While the administration of intravenous gadolinium can help distinguish myocarditis from idiopathic dilated cardiomyopathy, gadolinium crosses the placenta, and 0.04 % of the maternal dose can be found in breast milk. The 2013 American College of Radiology and the European Society of Radiology statements on safe MRI practices discourage the use of IV gadolinium during pregnancy unless it is absolutely essential [77, 78]. Because less than 1 % of the contrast medium ingested by the infant is absorbed from its gastrointestinal tract, the expected systemic dose absorbed by the infant from the breast milk is less than 0.0004 % of the intravascular dose given to the mother [79]. While the risk is considered remote, the concern can be alleviated by instructing the mother to discontinue breastfeeding for 24 h after administration of gadolinium.
Differential Diagnosis of Peripartum Cardiomyopathy
By definition, peripartum cardiomyopathy is a diagnosis of exclusion and, as such, requires the clinician to consider alternative diagnoses. Patients with idiopathic dilated cardiomyopathy have identical symptoms but present in either the first or second trimester. They also have nonspecific findings on ECG, evidence of congestion on chest X-ray, elevated BNP, and reduced left ventricular ejection fraction. Patients with familial dilated cardiomyopathy likewise present with heart failure by the second trimester. They report heart failure among family members and often have markedly dilated left ventricles with reduced ejection fraction. Genetic testing remains a research tool and is not routinely employed in clinical practice. Human immunodeficiency virus (HIV)-associated cardiomyopathy is a well-described entity, typically with reduced left ventricular ejection fraction but not significant left ventricular dilatation. Women with preexisting hypertension may present with heart failure. Classically, the ECG shows left ventricular hypertrophy. Echocardiography may similarly show left ventricular hypertrophy with either preserved left ventricular systolic function (heart failure with preserved ejection fraction or diastolic dysfunction) or dilated cardiomyopathy. Acquired valvular heart disease such as rheumatic mitral stenosis may be unmasked in the first or second trimester of pregnancy and can be confirmed by echocardiography. Acute myocardial infarction secondary to spontaneous coronary dissection and acute pulmonary embolism can also present with heart failure and cardiogenic shock.
Congenital heart disease , as categorized by the World Health Organization , ranges from Class I (low risk) to Class IV (pregnancy contraindicated) [80]. Pregnant women with underlying cyanotic heart disease, moderate to severe left-sided valvular stenosis, aortic root dilation, and reduced left ventricular ejection fraction are at greatest risk. WHO Class I conditions include mild, uncomplicated valvular lesions (pulmonary stenosis, patent ductus arteriosus, and mitral valve prolapse) as well as successfully repaired simple lesions (atrial or ventricular septal defect, patent ductus arteriosus, and anomalous pulmonary venous drainage). WHO Class II lesions include unrepaired atrial or ventricular septal defects and repaired tetralogy of Fallot. WHO Class II–III lesions include mild left ventricular impairment, hypertrophic cardiomyopathy, native or tissue valvular heart disease not considered WHO I or IV, Marfan syndrome without aortic dilatation, aorta <45 mm in aortic disease associated with bicuspid aortic valve, and repaired coarctation of the aorta. WHO Class III conditions include mechanical valves, systemic right ventricle, Fontan circulation, unrepaired cyanotic heart disease, other complex congenital heart disease, aortic dilatation (40–45 mm) in Marfan syndrome, and aortic dilatation (45–50 mm) in aortic disease associated with bicuspid aortic valve. WHO Class IV conditions (pregnancy contraindicated) include pulmonary arterial hypertension of any cause, severe systemic ventricular dysfunction (LVEF < 30 %, NYHA III–IV), previous peripartum cardiomyopathy with any residual impairment of left ventricular function, severe mitral stenosis, severe symptomatic aortic stenosis, Marfan syndrome with dilatation of the aorta >45 mm, and aortic dilatation >50 mm in aortic disease associated with a bicuspid aortic valve, and native severe coarctation of the aorta.
Treatment of Peripartum Cardiomyopathy
Acute management: The initial management of peripartum cardiomyopathy, directed at oxygenation and hemodynamic support, is comparable to the management of heart failure in pregnancy [81]. Supplemental oxygenation should be provided to ensure that oxygen saturation remains above 95 %. If nasal cannula and non-rebreather facemask prove inadequate, then noninvasive ventilation with a positive end-expiratory pressure of 5–7.5 cm H2O should be instituted. Intravenous furosemide (risk category C), with an initial dosage of 20–40 mg, should be administered to patients with hypervolemia on exam. IV nitroglycerin (starting at 10–20 up to 200 mg/min) can be initiated in patients with a systolic blood pressure (SBP) > 110 mmHg and can be used with caution in patients with an SBP between 90 and 110 mmHg. IV nitroglycerin (risk category B) is preferred over IV nipride (risk category C) which may be associated with thiocyanate toxicity.
Cardiogenic shock , as manifested by low cardiac output and hypoperfusion [hypotension, cool/clammy skin, low urine output (<0.5 ml/kg/h), mental status changes, hepatic dysfunction], may require the combination of vasodilator and inotropic therapy. Dopamine (risk category C) is an inotrope with a dose-dependent effect on the peripheral vasculature. Dobutamine (risk category B) combines the inotropic and vasodilator properties. Vasopressors such as phenylephrine and norepinephrine should be avoided because their vasoconstrictor properties impair cardiac output, and they can impair uterine blood flow.
There is limited experience with mechanical support devices—intra-aortic balloon pump (IABP) counterpulsation [82, 83], left ventricular assist devices (LVADs ) [84–88], and extracorporeal membranous oxygenation [89]—in the setting of pregnancy. The intra-aortic balloon pump is less invasive and does not require anticoagulation, but provides only mild augmentation in cardiac output. Percutaneous and surgically implanted left ventricular devices significantly augment cardiac output (2.5 = 5.0 L/min), are more invasive, are associated with greater risk, and require anticoagulation. Both the IABP and percutaneous LVAD devices such as the Impella have the added risk of radiation exposure associated with fluoroscopy. The LVAD can serve as a bridge to transplantation among women who do not recover from cardiomyopathy or may be removed if left ventricular function recovers.
Cardiac transplantation has been successfully performed in peripartum cardiomyopathy patients [90–92]. The incidence of cardiac transplantation for peripartum cardiomyopathy ranges from 0 to 11 % [31, 32, 93]. Rasmusson et al. reported on cardiac transplantations performed on 69 women from 29 US hospitals [94]. The risk of rejection was higher for women without prior pregnancies but similar among women who had previously given birth. The cumulative incidence of mortality and allograft vasculopathy was lower among women who had been transplanted for peripartum cardiomyopathy.
Chronic management: The pharmacological treatment of peripartum cardiomyopathy patients following delivery is identical to the treatment of idiopathic dilated cardiomyopathy [81]. Special consideration should be given to pharmacological therapy as several of the commonly used medications for idiopathic dilated cardiomyopathy are either untested or contraindicated in pregnancy [80]. Both angiotensin II receptor blockers and angiotensin-converting enzyme (ACE) inhibitors are contraindicated (risk category D) and pose the risks of renal or tubular dysplasia, oligohydramnion, growth retardation, ossification disorders of the skull, lung hypoplasia, contractures, large joints, anemia, and intrauterine fetal death. Spironolactone (risk category D) can have antiandrogenic effects and cause oral clefts during the first trimester and is therefore contraindicated. Eplerenone remains unclassified because of limited experience. As an alternative, the combinations of hydralazine (risk category C) and isosorbide dinitrate (risk category B) may be used to treat cardiomyopathy. Beta blockers (risk category B) have not been shown to have teratogenic effects but may produce hypoglycemia and bradycardia in the fetus and may result in intrauterine growth delay. β-1 selective drugs may be preferred because β-2 agents could have an anti-tocolytic effect. Hydrochlorothiazide (risk category B) and furosemide (risk category C) have both been used in pregnancy. Excessive diuretic use may lead to oligohydramnion. Unfractionated heparin (risk category B) can be utilized during pregnancy, whereas coumadin (risk category D) poses the risk of embryopathy during the first trimester and fetal hemorrhage throughout the pregnancy. The decision for anticoagulation in the setting of peripartum cardiomyopathy must take the maternal and fetal risks into consideration.
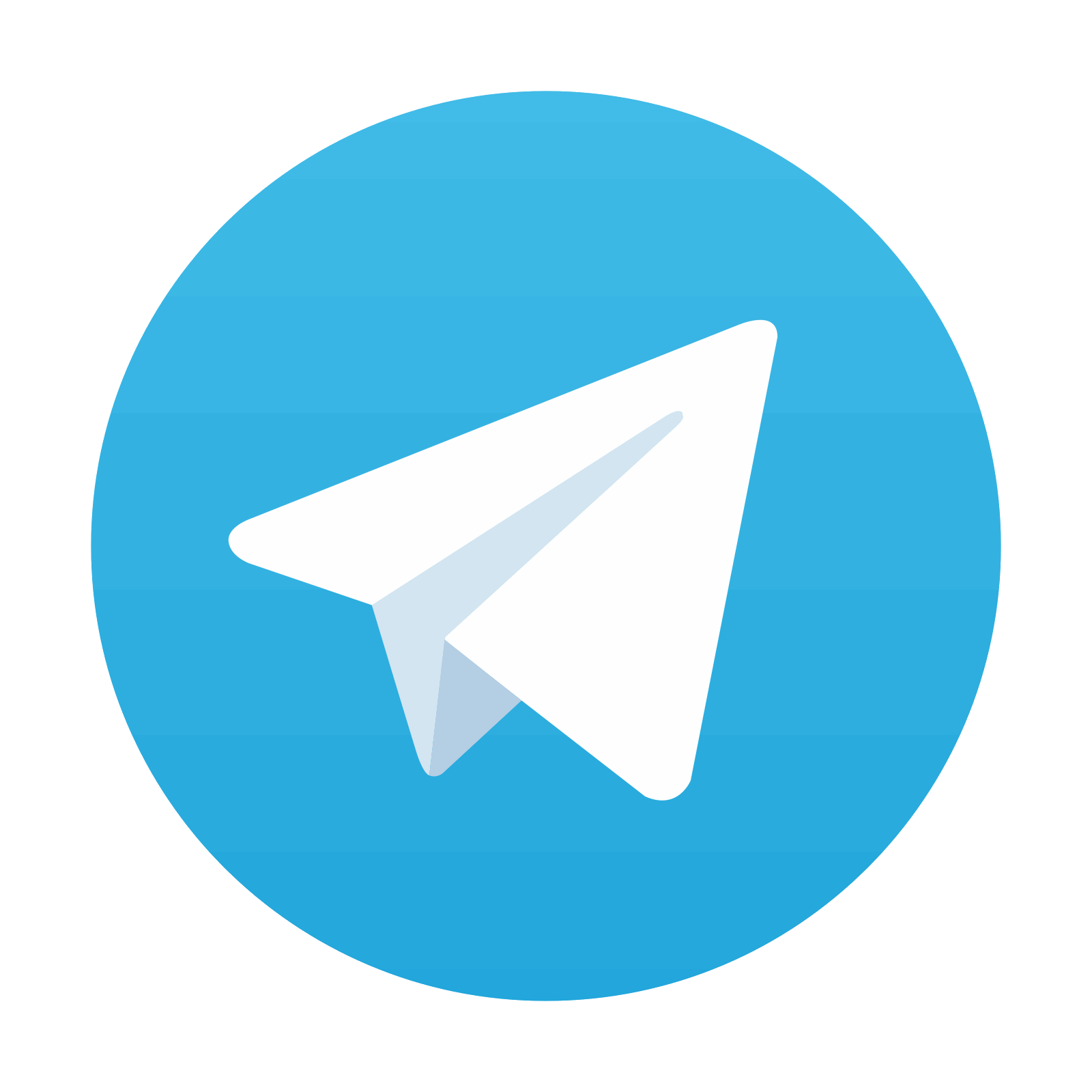
Stay updated, free articles. Join our Telegram channel

Full access? Get Clinical Tree
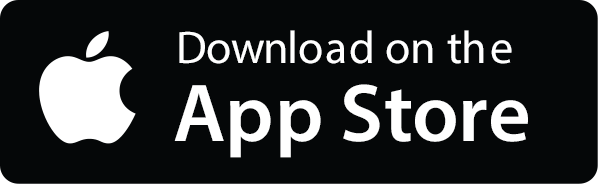
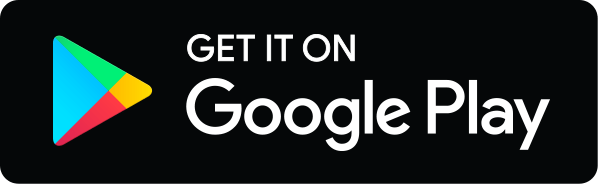