Fig. 19.1
Top panel. Right ventricular (RV) and pulmonary arterial (PA) pressures before and after compression of the left PA in an experimental animal (swine). Changes in both the amplitude and morphology of the pressure waveforms are evident. Bottom panel. Changes in the RV pressure–volume relationship produced by compression of the left PA are depicted
These differences reflect fundamental properties of each chamber in regard to the pattern of free wall contraction (the RV shows sequential shortening from the inflow to outflow tract), septal motion, the timing of peak pressure (early for the RV, late for the LV), and the contribution of inertia to maintaining flow out of the ventricle late in systole (minimal for the LV) [24, 25]. For a normal RV ejecting into a normal pulmonary circulation, these differences may complicate simple application of principles derived for characterization of LV systolic function such as the linear end-systolic pressure–volume relationship based upon automated detection of a discrete end-systolic point from pressure–volume loop analysis, and the myocardial performance index based in part upon echocardiographic assessment of isovolumic relaxation time. However, in the setting of PAH, RV free wall shortening can become more synchronous, ventricular interdependence changes, peak pressure occurs later in systole, and the inertial component of blood flow into the PA is diminished [24, 25]. As shown in Fig. 19.1, under these conditions the RV pressure–volume relationship resembles that for the LV with a more clearly defined end-systolic point and an isovolumic relaxation period.
Preoperative Evaluation
In 2007, the American College of Cardiology and the American Heart Association published the most current guidelines for perioperative cardiovascular evaluation for noncardiac surgery. This expert consensus document stratifies patients into three categories of risk factors: major, intermediate, and minor clinical predictors. Similarly the guidelines also categorize the type of surgery as high, intermediate, or low risk [26]. This type of risk calculation can be used to consider the specific perioperative risk for PH patients.
Assessing Surgical Risk with PH
For general practitioners and many cardiologists, the specifics of surgical procedures may not be known. The ACC/AHA guidelines define high-risk procedures to include emergent major operations, aortic and major vascular surgery, peripheral vascular surgery, and anticipated prolonged procedures associated with large blood loss or fluid shifts [26]. For PH patients this list must be expanded to include procedures with risk for venous embolism (air, fat, cement), elevations in venous pressures (laparoscopy, Trendelenburg positioning), reduction in pulmonary vascular volume (lung compression or resection), perioperative systemic inflammatory response, and emergency procedures (Table 19.1). Again, effective communication between the PH specialist, surgeon and anesthesiologist can determine the risks and benefits of the proposed surgery or surgical technique. This will be revisited below.
Table 19.1
Risk factors for morbidity and mortality in noncardiac surgery
Patient factors |
• NYHA/WHO FC ≥ II [14] |
• Higher ASA class [12] |
• RAD on ECG [14] |
• Echo parameters: RVH, RVMPI ≥ 0.75 [14] |
Operative factors |
– Procedures inducing severe systemic inflammatory response [79] |
• Intra-operative vasopressor use [14] |
Assessing Patient Risk Factors in PH
A known history of PH will prompt an evaluation of functional status, cardiac function (especially RV function), pulmonary function and current severity of disease. Echocardiography and right heart catheterization are essential to make these assessments. Meyer et al. reviewed major risk factors as being, an elevated right atrial (RA) pressure, a 6 min walking distance <399 m at the last evaluation and need for emergency surgery [16]. The discerning physician must also identify patients with risk factors for PH who as yet may have gone undiagnosed such as patients with scleroderma spectrum of disease, obstructive sleep apnea, cardiac valvular lesions, depressed left ventricular function, heart failure with preserved ejection fraction (HFpEF) or interstitial lung disease (ILD). At a minimum, symptoms of pulmonary hypertension or RV failure should be elicited (shortness of breath, abnormal cardiac silhouette on chest radiograph, elevated jugular venous distension, etc.). Intermediate- or high-risk surgeries might prompt a screening echocardiogram.
Comorbidities such as coronary artery disease (CAD), chronic renal insufficiency, history of pulmonary embolism, NYHA functional class III/IV have been correlated with increased morbidity and mortality in cardiac surgery. Right ventricular impairment is also a predictor of worse outcome. Right axis deviation (RAD) (p = 0.02), RV hypertrophy (RVH) (p = 0.04), RV index of myocardial performance (RVMPI) ≥0.075 (p = 0.03), RV systolic pressure to systemic systolic blood pressure ratio ≥2/3 (p = 0.01) all predict increased postoperative mortality [14].
Evaluation
The preoperative evaluation of a patient with pulmonary hypertension should seek out indications of right ventricular dysfunction to determine areas for potential optimization or disqualification for surgery due to unacceptable risk. This section will review common preoperative testing modalities to highlight their utility in diagnosing right ventricular insufficiency.
History and Physical
The evaluation begins with the history and physical examination. The most common complaints among PH patients, albeit nonspecific, are dyspnea and generalized fatigue [27]. Angina, pre-syncope, and syncope are indications of advanced PAH and may portend a poor prognosis [27, 28]. Physical signs of elevated RV pressure include jugular venous distension, right ventricular S3 gallop, hepatomegaly, ascites, and peripheral edema. By contrast, pulmonary crackles indicate left-sided heart failure or primary lung disease and not PAH.
Echocardiography
Transthoracic echocardiography (TTE) is easy to obtain and an excellent screening tool to estimate pulmonary arterial pressures as well as evaluate cardiac function. A complete assessment of ventricular and valvular pathology provides invaluable data to determine advancement of disease as well as etiology. By measuring the tricuspid regurgitant jet velocity, the PA systolic pressure (PASP) can be estimated. There is limited accuracy of this measurement in PH patients compared to catheterization, but it is useful as a screening tool [29]. Right ventricular dilation and contractility vary depending on severity and chronicity of disease. Objective measurements of RV function include tricuspid annular plane systolic excursion (TAPSE), RV fractional area change, and RV myocardial performance index, and possible use of mid and late systolic RV outflow Doppler notching to assess RV-PA coupling [30, 31]. Patent foramen ovale can be discovered by injection of agitated saline contrast, as well as intracardiac or intrapulmonary shunts, which may have clinical significance in the operative setting.
The elevated RV pressure during systole results in paradoxical ventricular septal flattening, pathognomonic for PH. Similar septal flattening during diastole marks RV volume overload, typically from RV failure with or without severe tricuspid regurgitation. Analysis of the blood flow through the mitral valve and pulmonary veins, as well as tissue Doppler velocities can determine LV filling patterns and identify elevations in left atrial pressure. Of note this technique is limited to patients in normal sinus rhythm. Left atrial size and function are useful to assess for the WHO 2 PH patient.
TTE may be used to suggest the possibility of HFpEF; however, cardiac catheterization is generally used to confirm the diagnosis. Reduced LV systolic function, severe left sided valvular lesions and left atrial enlargement in the presence of PH suggest a post-capillary etiology. Low grade diastolic dysfunction observed on echocardiography may still be attributable to pre-capillary PH and is not uncommon in PAH, parenchymal lung disease or CTEPH, when LV filling is impaired by altered RV function. It is not surprising that markers of significant RV dysfunction (right atrial enlargement, reduced TAPSE, increased interventricular septal flattening) are associated with poor overall prognosis in PAH patients and can probably be extrapolated to expect poor perioperative outcomes as well [31–33].
Heart Catheterization and Preoperative Optimization
Right heart catheterization (RHC) should be considered for PH patients undergoing intermediate- to high-risk operations or patients with moderate or severe PH by history, noninvasive screening, or with related comorbidities (e.g., obesity, sleep apnea, scleroderma, or risk factors for HFpEF such as atrial fibrillation and LAE). Left heart catheterization should also be performed in patients with coexisting left heart disease because of discrepancies between PCWP and left ventricular end-diastolic pressure (LVEDP) that could lead to misclassification of PH and have significant implications in treatment paradigms [34].
Ideally, RHC should be performed well in advance of surgery to allow for an adequate period of optimization for elective and semi-elective surgery. In all other cases, attempts should be made to lower PVR and enhance RV function prior to surgery. Routine vasoreactivity testing is performed during diagnostic RHC to determine candidates for vasodilator therapy [35, 36]. Although inhaled nitric oxide (iNO) is the drug of choice for testing due to lack of systemic effects and ease of administration, intravenous adenosine, epoprostenol, sildenafil, and inhaled iloprost can also be tried. Pre-capillary PH patients may benefit from advancing targeted pulmonary vasodilator therapy. When surgery cannot be delayed, phosphodiesterase 5 inhibitors (PDE5-I) such as sildenafil 20–40 mg three times daily, can provide acute vasodilatory effects and augmentation of right ventricular inotropy [37]. In those with high-risk hemodynamics (high right atrial pressure (RAP), low cardiac index (CI)), initiation of parenteral prostanoids should be considered prior to surgery. Furthermore, this same vasoactive testing is likewise useful in the perioperative period to guide intraoperative and postoperative management, such as the use of iNO, inhaled prostacyclins, and less commonly continuous epoprostenol. Patients with parenchymal lung disease and a component of hypoxic pulmonary vasoconstriction may also experience an improvement in PA pressures via an iNO mediated improvement in V/Q matching. Post-capillary PH patients may not benefit at all from pulmonary vasodilator therapy and moreover treatment may worsen pulmonary edema formation. In these patients, perioperative treatment should focus on diuresis and control of systemic hypertension. Some of the sickest WHO group 2 PH patients may need preoperative inodilators for low output states with aggressive up titration of the systemic vasodilators. WHO group 2 PH patients who have an increase in their PCWP in response to pulmonary vasodilators are at risk for developing worsening pulmonary vascular congestion and an increase in the driving force of left atrial hypertension with use of pulmonary vasodilators. Abnormal pulmonary function should be optimized with treatments such as oxygen, continuous positive airway pressure (CPAP), bronchodilators, antibiotics, and steroids where appropriate. Physical therapy and weight loss in obese patients may be beneficial although usually require a long-term plan [38, 39].
Optimal hemodynamic stability would include: mean arterial pressure (MAP) ≥ 60 mmHg, systolic BP ≥ 85 mmHg, oxygen saturation > 92 %, RAP < 12, mPAP < 35 (if feasible), PVR/SVR < 0.5 (if feasible), PCW 8–12 (some WHO 2 PH < 18), and CI ≥ 2.2 L/min/m2.
Dyspnea at rest, syncope, hemodynamic findings of severe RV failure (low CI, high RAP >15 mmHg), metabolic acidosis, and marked hypoxemia are all signs of advanced, unstable disease and serious consideration should be given to cancelling or postponing the surgery until/unless improvement and stabilization or pulmonary hypertension can be achieved [37].
Planning for Surgery
Preoperative coordination of care among the multidisciplinary team is crucial for best outcomes. Ideally, all patients except those with the lowest risk PH and lowest risk procedures should be operated on in a tertiary care center, where a multidisciplinary team of specialists experienced in managing patients with PH is available. The multidisciplinary team for preoperative management of PH patients includes anesthesiologist, cardiologists, intensivists and pulmonologists, surgeons, and experienced allied health-care members including respiratory therapists, pharmacy (availability of medications and a pharmacist with experience in administration of PH therapies), as well as nurse managers (systemic prostacyclin administration requires staff training and often is approved only in certain units). Meticulous advanced planning and discussion amongst team members must take place to transition from oral to IV/inhaled therapies where prolonged surgery/intubation/extended NPO periods are expected. Generally, chronic PAH therapies, including PDE5-I (sildenafil, tadalafil), endothelin receptor anatagonists (ERAs) (bosentan, ambrisentan, macitentan), and prostanoids (inhaled, intravenous, subcutaneous, oral) should be continued throughout the perioperative period, with appropriate substitutions as above when necessary (intravenous for oral PDE5-I, intravenous for subcutaneous prostacyclin infusion, etc., depending on the surgery and interference of subcutaneous site,). If inhaled prostacyclin analogues cannot be continued due to intubation appropriate substitution should be planned with iNO, intermittent nebulized prostacyclin, continuous inhaled epoprostenol, vs. occasional conversion to IV prostanoids (there are no significant bleeding complications with IV prostanoids, despite platelet inhibition) [37]. Oral therapies should be resumed as soon as possible after procedure, keeping in mind that compromised absorption can result in low drug levels and rebound PH. Coumadin can usually be discontinued with judicious decision regarding heparin bridging depending on risk/benefit of bleeding vs. clotting (such as hypercoagulable states, pulmonary embolism (PE), or mechanical valves). In high-risk situations (such as major orthopedic surgeries), retrievable preoperative IVC filter placement may be considered [40]. Careful perioperative deep venous thrombosis (DVT) prophylaxis should be instituted and early ambulation is essential for both DVT/PE prevention and avoiding deconditioning.
Meetings with the patient and family must take place preoperatively and include some discussion of modes of anesthesia, need for invasive monitoring, and their role during the recovery period.
Intraoperative Management
The primary physiological concept is to maintain optimal right ventricular-pulmonary arterial coupling and promote adequate left sided filling and systemic perfusion. Thus, all interventions that affect RV preload, RV inotropy, RV afterload including pulmonary vascular resistance, large pulmonary artery capacitance or impedance, thoracic pressures, and oxygen supply and demand relationship need to be taken into consideration.
Right Ventricular Afterload
Pulmonary vascular disease leads to an increase in RV afterload that impedes RV ejection and thereby leads to increased RV wall stress, RV diastolic overload, RV dilation, and in the more chronic state, RVH. In contrast to the LV, the thinner walled RV is subjected to greater wall tension for the same degree of increase in end diastolic volume; this leads to an increase in RV myocardial oxygen demand and consumption.
Although often described simply as PVR (the steady-state, mean pressure/mean flow relationship largely dictated by small vessels), the true interaction between the RV and the pulmonary circulation is pulsatile and dynamic. Accordingly, the concept of input impedance has been applied as a means to summarize the resistive, elastic, and reflective components of afterload, and provide for some discrimination between the relative contributions of small vessels (steady state resistance) and large elastic ones (“characteristic” impedance) [25]. However, assessment of input impedance requires simultaneous measurement of pressure and flow, and generally involves analysis in the “frequency domain,” i.e., mathematical resolution of pressure and flow waves into their individual frequency components and then defining their ratio at set frequencies along a spectrum. Not surprisingly, the complexity of both measuring and interpreting input impedance spectra has limited clinical utility. Nonetheless, there has been general acceptance of “lumped parameter” models such as the Windkessel to help conceptualize the static and dynamic contributions to afterload. Essentially adaptations of electrical circuits, these models incorporate a resistor (PVR), a capacitor (vascular compliance), and an inductor (characteristic impedance) to represent the basic physiological components dictating input impedance. While alternative methods for calculating characteristic impedance as a measure of large vessel load from more conventional “time-domain” measures (PA pressure, PA diameter, and stroke volume) have been described [41], from a clinical perspective, prognostic significance has focused more upon compliance (calculated as stroke volume/pulse pressure) and its reciprocal relationship with PVR [42–45]. Data suggest that early in the course of PAH, a relatively small rise in PVR will be accompanied by a larger relative decline in compliance, while later in the disease course, the fall in compliance elicited by increased PVR will diminish since the vascular wall approaches maximum stiffness [41]. Functionally, an acute change in compliance will lessen the ability of large elastic vessels to “absorb” pressure waves reflected from more distal potions of the circulation. This effect can be directly observed in the RV and proximal PA pressure waveform as the timing of peak pressure achievement moves from early to late in systole. This “late phase load” produced by summation of reflective pressure components parallels the systolic augmentation described for systemic vessels and contributes to a widened PA pulse pressure. This is particularly relevant to acute insults that may occur during surgery and affect RV pulsatile load, and importantly, may be underestimated by PA catheter tracings where pressure is measured more distal in the circulation. For example, compliance and PVR may be altered by events such as the addition of positive end-expiratory pressure (PEEP) to mechanical ventilation (Fig. 19.2), a change to prone or Trendelenburg positions, pneumoperitoneum during a laparoscopic procedure (Fig. 19.3), venous emboli (including air emboli or particulate matter, i.e., from orthopedic procedures), and any direct compression or displacement of the large PA branches.
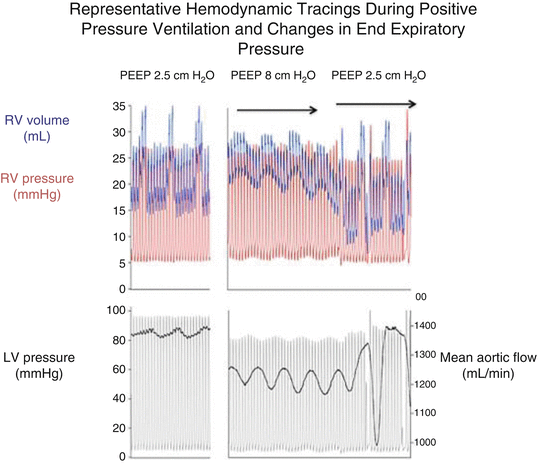
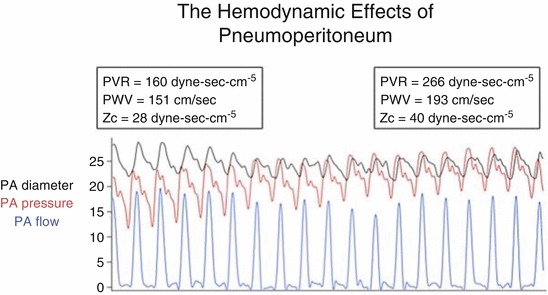
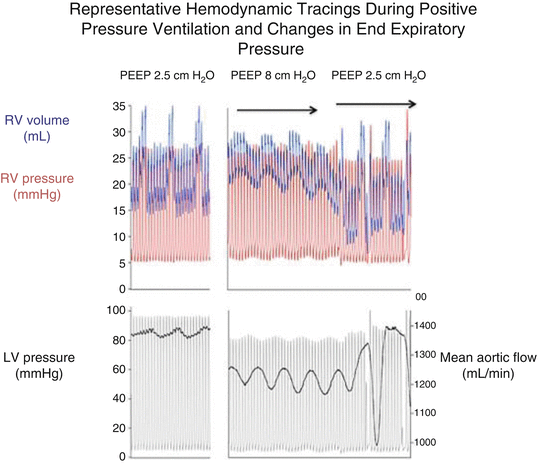
Fig. 19.2
Representative tracings of right (RV) and left (LV) ventricular pressures along with RV volume and aortic blood flow during positive pressure ventilation and variations in positive end-expiratory pressure (PEEP) in an experimental animal (dog). Marked respiratory variation in RV volume and aortic flow is evident, particularly with increased levels of PEEP
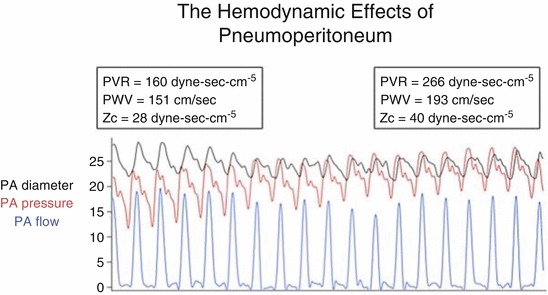
Fig. 19.3
The representative effect of inducing pneumoperitoneum and increasing positive end-expiratory pressure (PEEP) on pulmonary arterial (PA) pressure, diameter, flow, pulse wave velocity (PWV), and characteristic impedance (Zc). The data indicate an acute increase in right ventricular afterload in terms of effects on both pulmonary vascular resistance (PVR, primary determined by small vessels) and characteristic impedance (Zc, primarily determined by large vessels). In addition, the increase in pulse wave velocity (PWV) suggests that pressure waves will be reflected from the distal pulmonary circulation more rapidly, potentially contributing to increased afterload
Changes in Myocardial Supply and Demand
Under normal circumstances the RV intramyocardial pressure is lower than the aortic root pressure and the RV coronary perfusion occurs throughout the cardiac cycle. In PH, due to the elevated RV intramyocardial pressure, coronary flow occurs predominantly during diastole, which further worsens the mismatch between oxygen demand and supply promoting RV ischemia and diminished RV contractility [46, 47]. Low RV oxygen supply is associated with a shift away from aerobic glucose and fatty acid oxidation to the less efficient RV glycolytic pathways [25].
Systemic vasodilatation and hypotension with anesthesia (see Sect. “Choice of Anesthetics”) leads to a relative increase in the PVR/SVR ratios, and hypotension induced RV ischemia in the setting of increased oxygen demands [48].
Intraoperative manipulation of the heart and/or great vessels may further contribute to the hypotension.
Interventricular Dependence
The combination of elevated PVR/reduced RV compliance and systemic hypotension may promote RV ischemia resulting in the “lethal combination” of RV dilatation, interventricular septal bulging into the left ventricle, and insufficient left ventricular filling, with resulting progressive decline in CO and further systemic hypotension [49]. Management should be focused on RV unloading with pulmonary vasodilators, optimizing intravascular fluid balance, and maintaining adequate systemic pressure. Combination of RV inotropes and systemic pressors may be needed [50]. Dobutamine may be a preferred RV inotrope in this situation due to less systemic vasodilation as compared to milrinone which may cause further LV unloading and septal displacement; vasopressin may be a good option to maintain systemic pressure although there is some institutional bias as well with the choice of systemic pressors and catecholamine sparing. Ongoing monitoring of arterial pressure, central venous pressure, cardiac output, central venous oxygen saturation, arterial blood gases, and lactate levels are necessary, ideally supplemented by transesophageal echocardiography (TEE) guided assessment of RV/LV filling.
General Anesthetic Management
Preparation
Decision making regarding choice of anesthesia in a PH patient depends on the type of planned surgery, PH severity, comorbidities, and patient’s preference. While conscious sedation may result in less anesthetic related problems in a non-PH patient, even mild hypoxia and hypercarbia (frequently associated with conscious sedation) can cause pulmonary vasoconstriction and lead to sudden decompensation in a PH patient. Also, any baseline comorbidity predisposing to hypoxia (sleep apnea, obesity, lung disease) may be an additional indication for a protected airway. Thus, elective intubation and general anesthesia are frequently preferable. Also, any procedures associated with high risk of pulmonary emboli (such as orthopedic procedures) may require intubation, general anesthesia, and invasive hemodynamic monitoring in a PH patient, as to avoid emergent intubation should hemodynamic instability or suboptimal oxygenation be precipitated.
Particular care should be taken to de-air all lines and syringes, as even a small amount of air can cause hemodynamic decompensation in a PH patient [51]; there is also a high risk of passage of air to systemic circulation via PFO. Hypothermia should be avoided as it inhibits physiologic hypoxic pulmonary vasoconstriction (HPV) and may result in worsened VQ mismatch, which may have considerable effect in procedures requiring reduction of lung volume [52].
Airway and Optimal Ventilator Strategies
With any type of non-general anesthesia, airway patency needs to be insured, and an airway access should be planned should ventilation become compromised. With any means of anesthetic administration (face mask, laryngeal airway, or ET) supplemental oxygen must be administered for its direct pulmonary vasodilating effects [53].
With general anesthesia, carefully planning the induction is critical, as uncontrolled ventilation with possibility of hypoxia, and sympathetic stimuli from laryngoscopy can result in acute rise in PVR. Use of 100 % oxygen by mask prior to induction and optimizing depth of anesthesia prior to laryngoscopy and intubation can minimize this effect. In patient with difficult airway, OSA or intrinsic lung disease and poor functional reserve capacity, “awake intubation” with fiberoptic bronchoscopy may be preferable to avoid a period of poor ventilation with regular induction and intubation. Use of systemic pressors to protect against any acute vagal mediated vasodilatory response is often suggested.
After securing airway, ventilator management in PH is focused on use of higher FiO2, with mild hyperventilation (goal PCO2 of 35 % or less) and maintenance of lung volumes at normal functional residual capacity (Table 19.2) [54–56]. There is a U-shape relationship between lung volumes and PVR during mechanical ventilation, with PVR being the lowest at functional residual capacity. At low lung volumes, resulting hypercapnia and hypoxia will cause hypoxic vasoconstriction and an increase in PVR, while hyperinflation and high PEEP (preferably <10 mmHg) will result in an undesirable compression of the intra-alveolar vessels which can also lead to increase in PVR [56, 57].
Table 19.2
Perioperative ventilatory conditions to avoid and promote
Avoid pulmonary vasoconstrictors |
• Hypoxemia |
• Inspiratory pressure >30 mmHg |
• High PEEP (>15 mmHg) |
• Hypercapnia |
• Acidosis |
Promote pulmonary vasodilation |
• Improve oxygenation (e.g., FiO2 1.0) |
• Permissive hypocapnia (pCO2 ≤30–35 mmHg) |
• Alkalosis (pH N 7.4) |
• Optimal ventilatory volume |
Choice of Anesthetics
All anesthetic techniques have been safely employed in PH patients with appropriate judgment and monitoring. However, two anesthetic effects are of special significance when choosing an agent for a PH patient: avoiding direct myocardial depression and unfavorable effects on autonomic tone.
Many anesthetics are known to have myocardial depressant effects [58–62], by the means of directly affecting calcium cycling by myocytes, or the sensitivity of the contractile proteins to calcium, as well as their indirect effect on the autonomic nervous system. Direct myocardial depression is dose dependent. Propofol causes direct myocardial depression but only at relatively high concentrations and can still be used with caution [55, 57]. Studies showed that with frequently used inhaled anesthetics such as isoflurane, sevoflurane, and desflurane, depression of LV systolic function is offset by a decrease in systemic afterload. However, due to a smaller effect on decreasing RV afterload, there is a resulting disparity of LV and RV workload [60–62]. Ketamine has a modest myocardial depressant effect and with optimal ventilation and acid-base balance it may have pulmonary vasodilating properties [63, 64]. However, ketamine may also cause pulmonary vasoconstriction by the means of sympathetic stimulation [51]. With neuraxial (spinal, epidural) anesthesia, blockade of sympathetic nerves can precipitate hypotension. In addition high spinal or epidural anesthesia could result in cardiac sympathetic blockade, with unopposed parasympathetic stimulation from the cranial region [65]. Such an acute shift in autonomic balance in PH patient may result in profound hypotension and severe hemodynamic compromise [65]. In general, gradual epidural dosing or low spinal techniques are safe.
Intraoperative Pharmacological and Inhalation Therapy
Volume Status
Baseline hemodynamics, including “average” resting CVP, PA sat, and CO/CI are very useful at guiding intraoperative and postoperative fluid management [66]. For a normotensive patient, the goal should be to maintain the lowest baseline CVP. Whereas volume resuscitation is often guided by pulse-pressure variation (PPV), this is not feasible in PH patients. With the failing RV, PPV does not predict volume responsiveness and PPV due to increased RV afterload may erroneously suggest volume responsiveness [67, 68]. Although recent studies question the adequacy of both static (CVP) and dynamic (PPV) indices of preload assessment in PH, clinical experience suggests that targeting a CVP of 8–12 mmHg may have utility in managing systemic hypotension.
Pressors and Inotropes
Pressors of choice for the PAH patient include norepinephrine and vasopressin. While many institutions use phenylephrine and it is effective in increasing the coronary artery driving pressure it is less favorable for RV hemodynamics and relative PVR/SVR ratios [69, 70]. With more significant RV dysfunction, vasopressors with inotropic properties, such as norepinephrine and epinephrine, may be preferable. In experimental models, vasopressin was demonstrated to stimulate nitric oxide release and was vasodilating in pulmonary circulation, while causing peripheral vasoconstriction via V1 receptor stimulation [71], providing in vitro rationale for it as a preferable choice. Although there is no definitive clinical trial showing its superiority to catecholamines, there is some clinical experience [72], and applicability of the lessons of vasodilatory shock [73, 74]. For RV inotropy, especially with underfilled LV, dobutamine may be preferable to milrinone due to less arterial vasodilation [75]. For the WHO group 2 patient, milrinone or dobutamine are appropriate, with pressor support with norepinephrine or vasopressin as indicated.
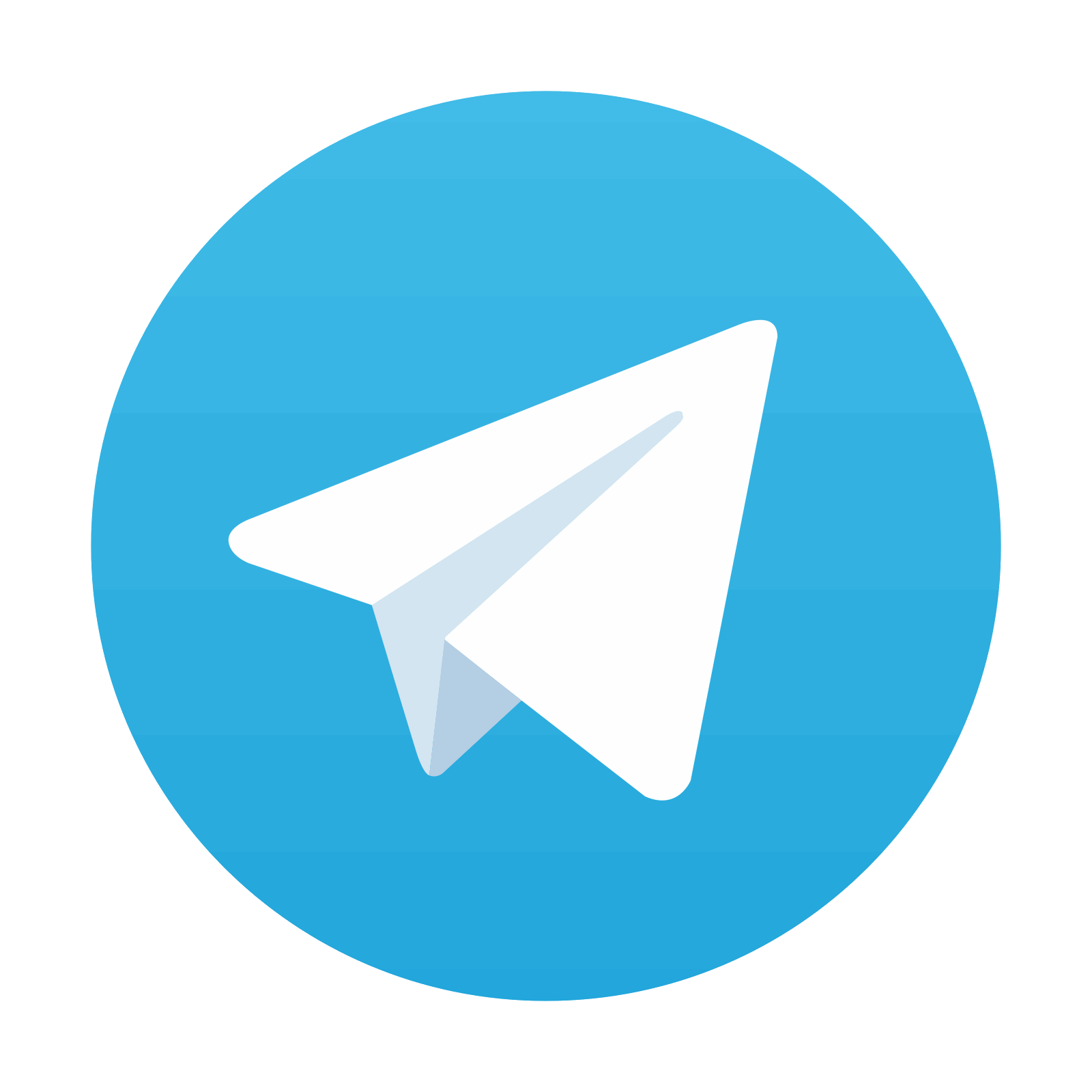
Stay updated, free articles. Join our Telegram channel

Full access? Get Clinical Tree
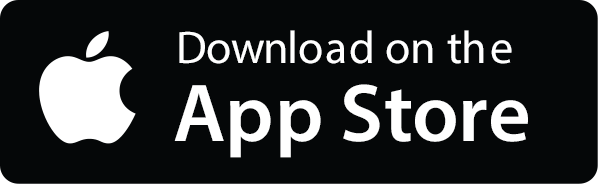
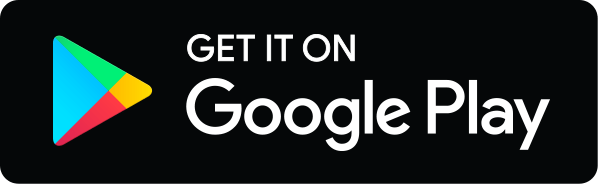
