2004 to 2007, especially in the setting of high-risk interventions and CS with higher-than-normal-risk profiles for surgically implanted LVADs as a bridge to recovery (BTR) (FIGURE 17.1).2,4 Despite increasing use of AMCS devices, an analysis of the National Cardiovascular Data Registry CathPCI-participating hospitals from 2009 to 2013 identified that the probability of non-IABP AMCS device use for CS was <5% for 1/2 of the hospitals and >20% in less than 1/10th of these hospitals.4
![]() FIGURE 17.1 Trends in the use of percutaneously delivered AMCS devices.2 PCPS, percutaneous cardiopulmonary support. Reproduced with permission from Stretch R, Sauer CM, Yuh DD, Bonde P. National trends in the utilization of short-term mechanical circulatory support: incidence, outcomes, and cost analysis. J Am Coll Cardiol. 2014;64:1407-1415. |
![]() FIGURE 17.2 Trends in the use of surgically implanted durable MCS.5 A, The number of LVADs being implanted as part of a BTR strategy or as a rescue therapy has significantly declined over the past decade. This shift in LVAD use away from unstable, high-risk INTERMACS profiles is partly driven by data showing increased mortality after LVAD implantation for INTERMACS profiles 1 and 2 after the age of 65 years and the increasing availability of AMCS devices, which include short-term, percutaneously inserted devices without the need for cardiac surgery. B, The predicted 1 year mortality after LVAD implantation is highest among the sickest patients defined as INTERMACS Levels 1 and 2. Reproduced with permission from Kirklin JK, Naftel DC, Kormos RL, et al. Fifth INTERMACS annual report: risk factor analysis from more than 6000 mechanical circulatory support patients. J Heart Lung Transplant. 2013;32:141-156. |
TABLE 17.1 Suggested Indications for AMCS6 | ||||||||||||
---|---|---|---|---|---|---|---|---|---|---|---|---|
|
TABLE 17.2 Potential Complications Associated with AMCS Devices | ||||||||||||
---|---|---|---|---|---|---|---|---|---|---|---|---|
|
![]() FIGURE 17.3 The acute hemodynamic support equation. BNP, brain natriuretic peptide; EDV, end diastolic volume; MAP, mean arterial pressure. |
diastolic pressure, the ejection phase begins; during this phase the ventricular volume decreases as the LV pressure reaches peak systolic pressure and then begins to relax (C); the residual LV volume is the end systolic volume. The end systolic pressure volume relationship (ESPVR) is a valuable measure of ventricular systolic function for both clinical and experimental evaluations. Changes in ESPVR represent changes in contractility, whereas the slope measures end-systolic chamber stiffness. Systole is then followed by the isovolumetric relaxation (D) before the next cardiac cycle.
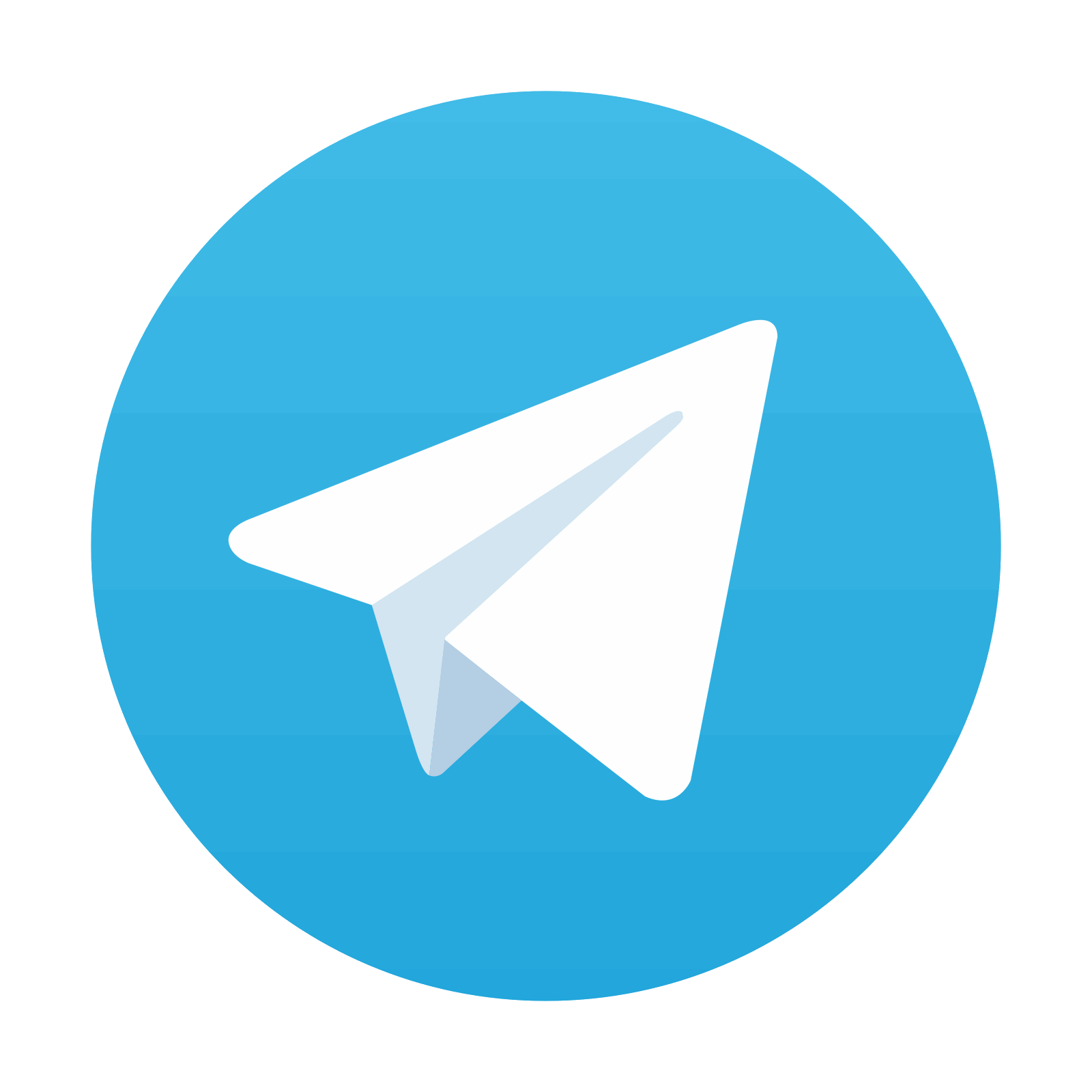
Stay updated, free articles. Join our Telegram channel

Full access? Get Clinical Tree
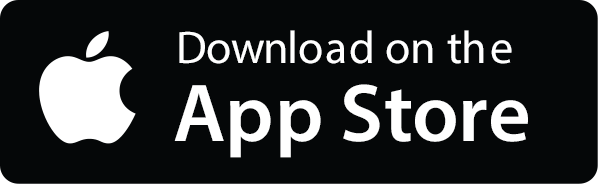
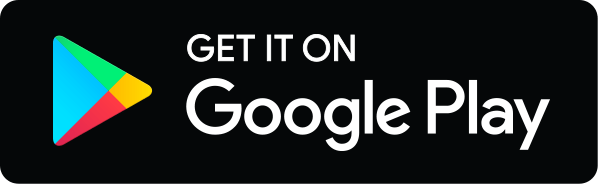