Transvesical method for measuring intra-abdominal pressure. (Adapted from Mullens with permission)
Pathophysiology of Abdominal Congestion Leading to Kidney Dysfunction
Abdominal Venous Congestion
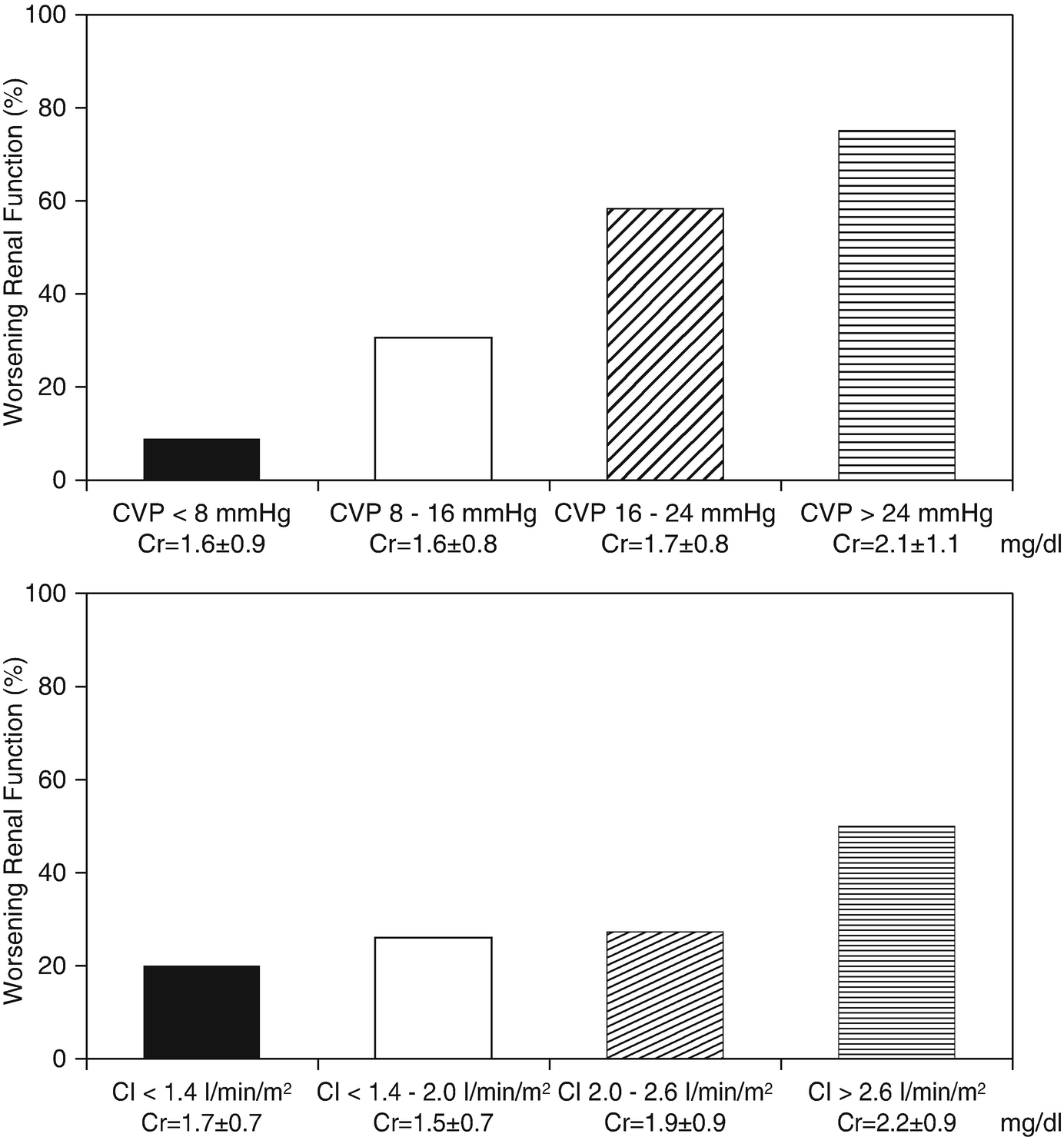
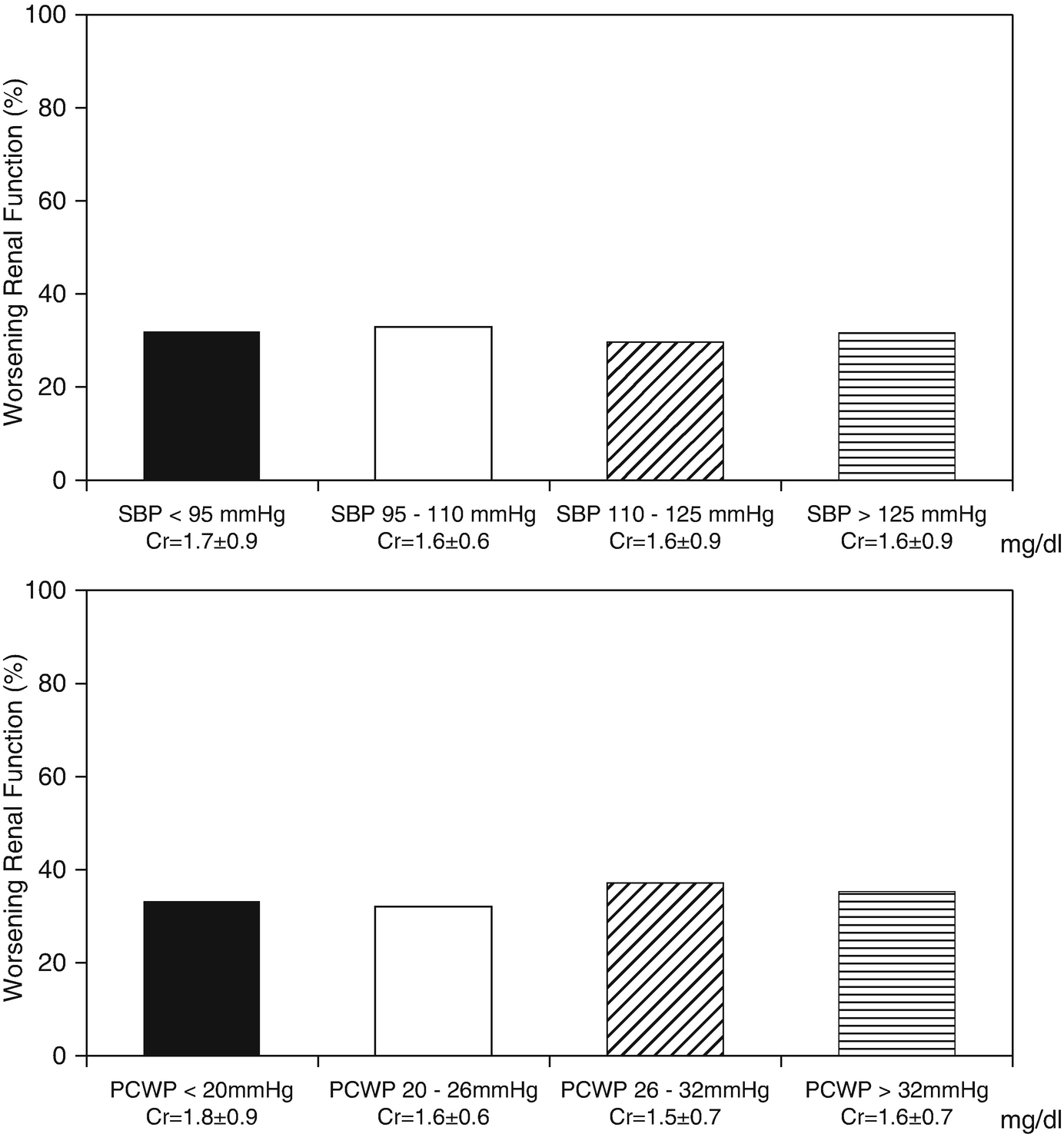
Prevalence of WRF according to categories of admission CVP, CI, SBP and PCWP. CVP denotes central venous pressure, CI denotes cardiac index, SBP denotes systolic blood pressure, and PCWP denotes pulmonary wedge pressure. (Adapted from Mullens with permission)
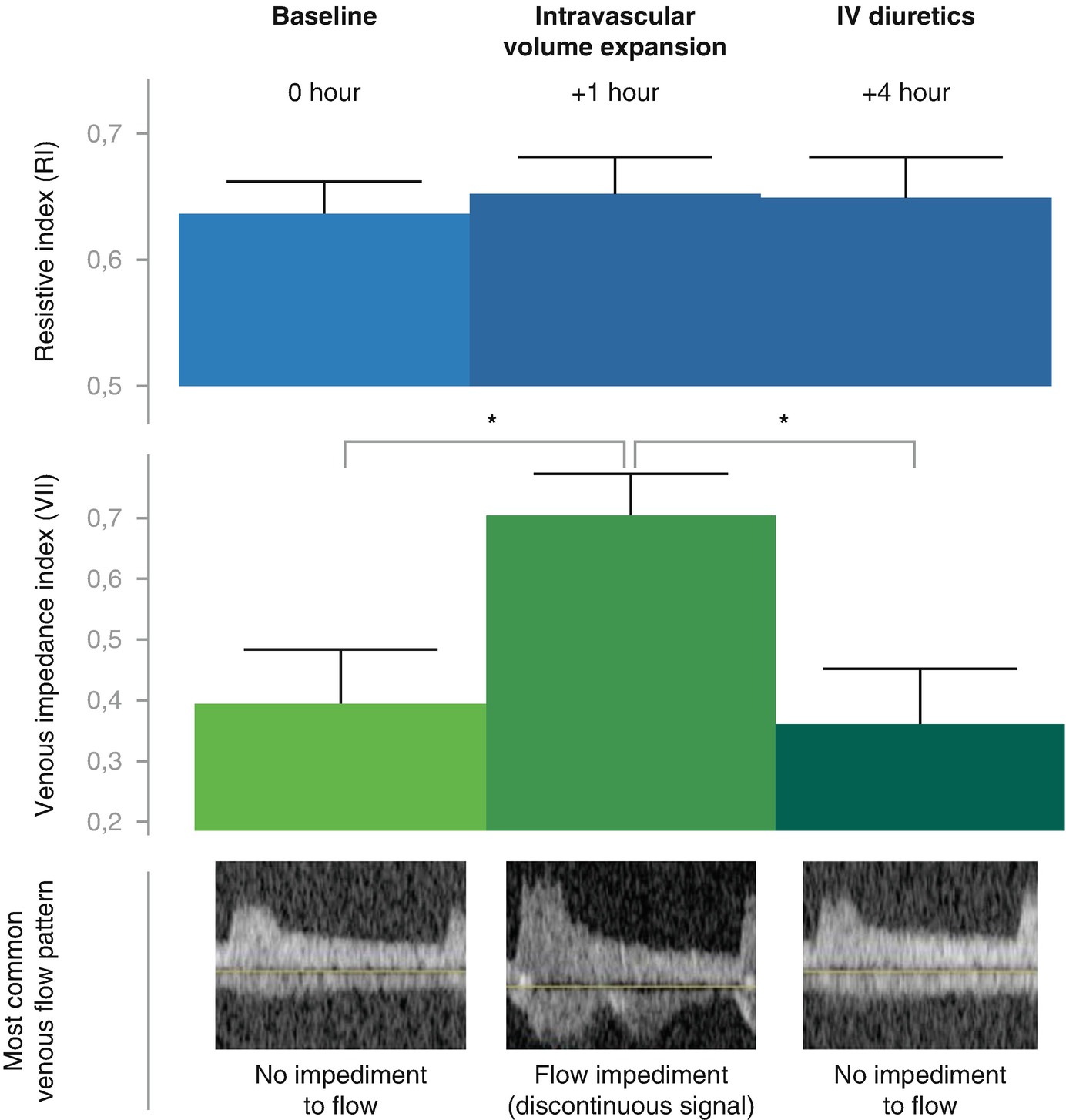
Venous flow patterns in heart failure patients in baseline compensated status, during volume expansion and during therapy with IV diuretics. (Adapted from Nijst with permission)
Extrinsic Kidney and Kidney Outflow Compression
In addition to a poor trans-renal pressure gradient mediated by backward failure (high venous outflow pressures) and forward cardiac failure (low kidney perfusion pressure), extrinsic kidney compression can result in further WRF in heart failure patients [6]. Importantly, the kidney is an encapsulated organ so intra-renal interstitial fluid built-up will further increase the parenchymateous pressures. Indeed, as earlier alluded to, an increased IAP is strongly associated with WRF, and reductions in IAP are associated with improvement in renal function (measured as a decline in plasma creatinine). A high IAP can mechanically obstruct the glomerular filtration force [2, 3]. The perfusion pressure of the abdomen is an important determinant of the perfusion of the visceral organs. Abdominal perfusion pressure (APP) is calculated as mean arterial pressure (MAP) minus the obstruction to venous outflow by the IAP. Elevated IAP induced kidney function is proposedly mediate by a low renal perfusion pressure and low renal filtration gradient (FG). The filtration gradient is the mechanical force across the glomerulus and equals the difference between the glomerular filtration pressure (GFP) and the proximal tubular pressure (PTP) [2, 3]. In the presence of an elevated IAP, PTP may be assumed to be equal to IAP and GFP is equated as MAP minus IAP. Therefore, the FG = GFP-PTP or FG = MAP – 2x IAP. During decongestive therapy in patients with ADHF, both a reduction in IAP and an increase in FG results in an improvement of serum creatinine [8].
Bowel Wall Congestion – Altered Pharmacology and Inflammation
During ADHF increased hydrostatic venous pressures in the abdomen result in net more filtration at the level of the microcirculation [1]. This can result in the formation of bowel wall edema, if lymphatic reabsorption forces are overwhelmed. Several observational studies in heart failure patients with reduced ejection fraction have shown that patients with high right atrial pressure often manifest with increased bowel wall thickness on abdominal ultrasound. It is well recognized that such formation of abdominal congestion is associated with a reduced appetite and a sensation of abdominal satiety [18, 19]. Furthermore, it is well documented that in the presence of abdominal edema the uptake of oral loop diuretics become less predictable. This results in a reduced bio-availability of loop diuretics in the circulation and potentially leading to incessant congestion. Furthermore, the villi in the bowel wall are very sensitive to changes in blood flow due to the countercurrent system in their arteriovenous supply. Therefore, a state of low cardiac output and venous congestion often results in villi tip ischemia, which is associated with increased bowel wall permeability. This lead to translocation of gram-negative bacteria that normally only reside in the bowel lumen. These gram negative bacteria carry lipopolysaccharides (LPS) on their cell walls, which activate the immune system [18, 19]. Hereby contributing to the overall state of inflammation often seen in heart failure. Interestingly, in patients with cirrhosis, the LPS-induced endotoxicity is strongly associated with the development of hepatorenal syndrome [20].
Treatment Strategies for Abdominal Congestion in Acute Heart Failure
Relieving Congestion During ADHF
As increased filling pressures (congestion) drive the progression of the disease in ADHF and strongly determine symptoms, the goal of therapy should be to completely relief congestion [21]. Lingering congestion following discharge is one of the strongest predictors of adverse outcome following a heart failure hospitalization. Furthermore, hemoconcentration (a marker of relieving excessive plasma volume) is associated with improved outcome in ADHF [22]. As earlier alluded to, increased venous filling pressures can occur both if the increased plasma volume overshoots the splanchnic venous buffering capacity or due to a reduced compliance of the splanchnic venous system. With the former mechanism often being labeled volume overload and the latter mechanism being labeled volume misdistribution. Clearly in clinical practice both these mechanisms (plasma volume expansion and reduced venous capacitance) overlap and contribute to the presence of congestion. If the ADHF-patient clearly manifest with signs of volume overload (e.g. weight gain, pleural effusion, peripheral edema, ascites, ..), than the goal of therapy should be to completely get rid of excessive volume. Loop diuretics remain the cornerstone of diuretic therapy in AHF, with almost 90% of patients receiving intravenous loop diuretics in the ADHERE database [23]. Furthermore, in 63% of patients, loop diuretics are the sole drug therapy being used to combat AHF [23]. In the DOSE trial, no difference was seen between continuous versus bolus infusion. However, patients receiving a high dose of furosemide (median dose of 773 mg vs 358 mg over 72 hours), demonstrated a trend towards faster dyspnea relief and a significantly higher net fluid and weight loss [24]. Adjusting the employed dose of loop diuretics is often necessary when a low glomerular filtration rate is present, with higher doses needed in this setting. Furthermore, recently it has been illustrated that early initiation of loop diuretics might be associated with better outcome [25]. In the case of severe abdominal congestion loop diuretics should be administered intravenous as bowel congestions make oral absorption of loop diuretics less predictable. However, a fair proportion of patients do not attain decongestion despite therapy with a loop diuretic. In these patients it is less clear if further loop diuretic dose uptitration or combinational diuretic therapy should be employed. Several additional agents with a diuretic property such as thiazides, high dose mineralocorticoid receptor antagonists, acetazolamide or sodium glucose linked transporters can be used. A detailed description of their use spans beyond the scope of this chapter.
When volume redistribution is driving congestion, the goal of therapy should be to enhance venous capacitance function and lower cardiac filling pressures [26]. To achieve this goal a combination of vasodilators and lower doses of intravenous diuretics are often employed. Again a detailed discussion spans beyond the scope of the chapter but have been published previously.
Reducing IAP Specifically
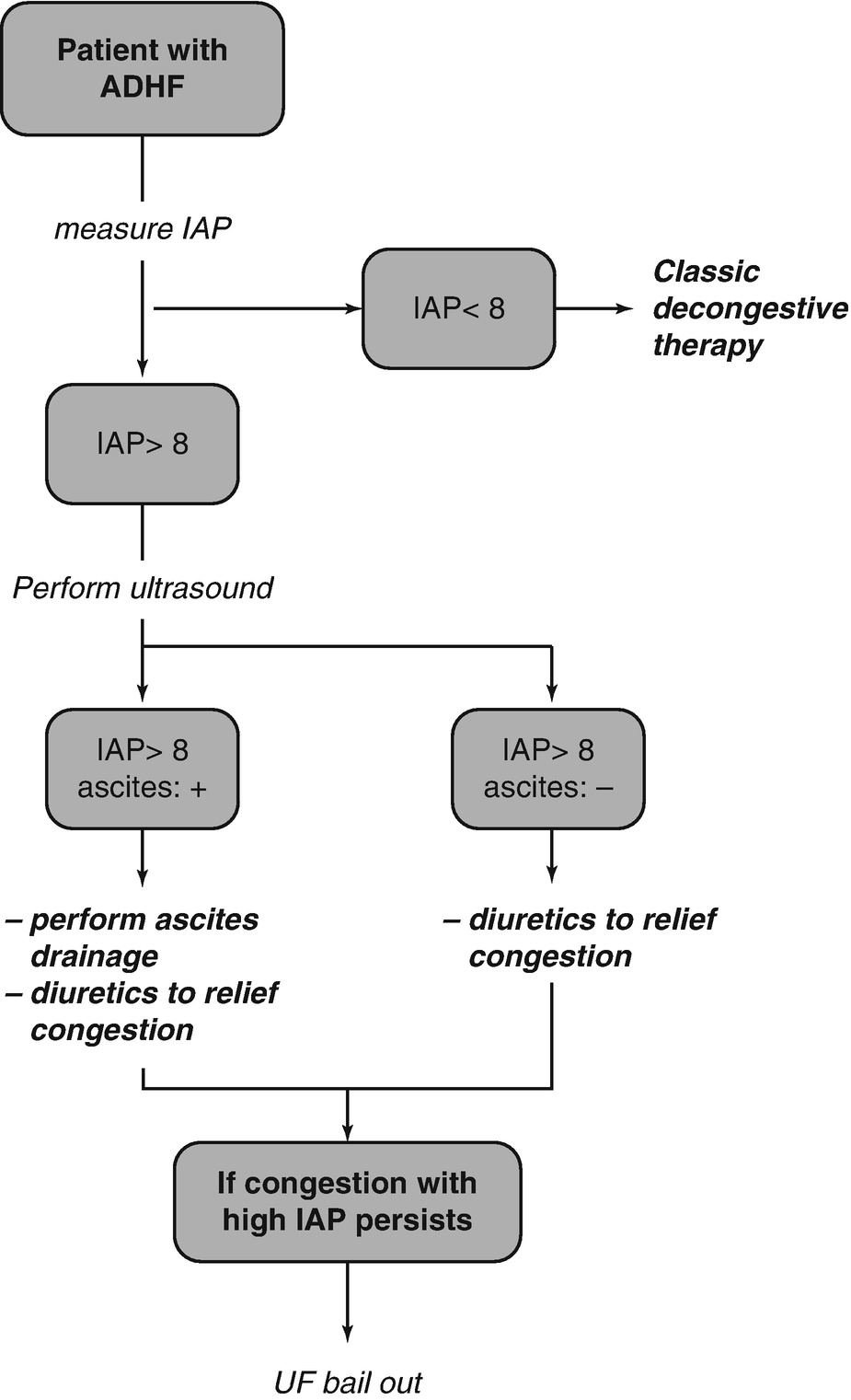
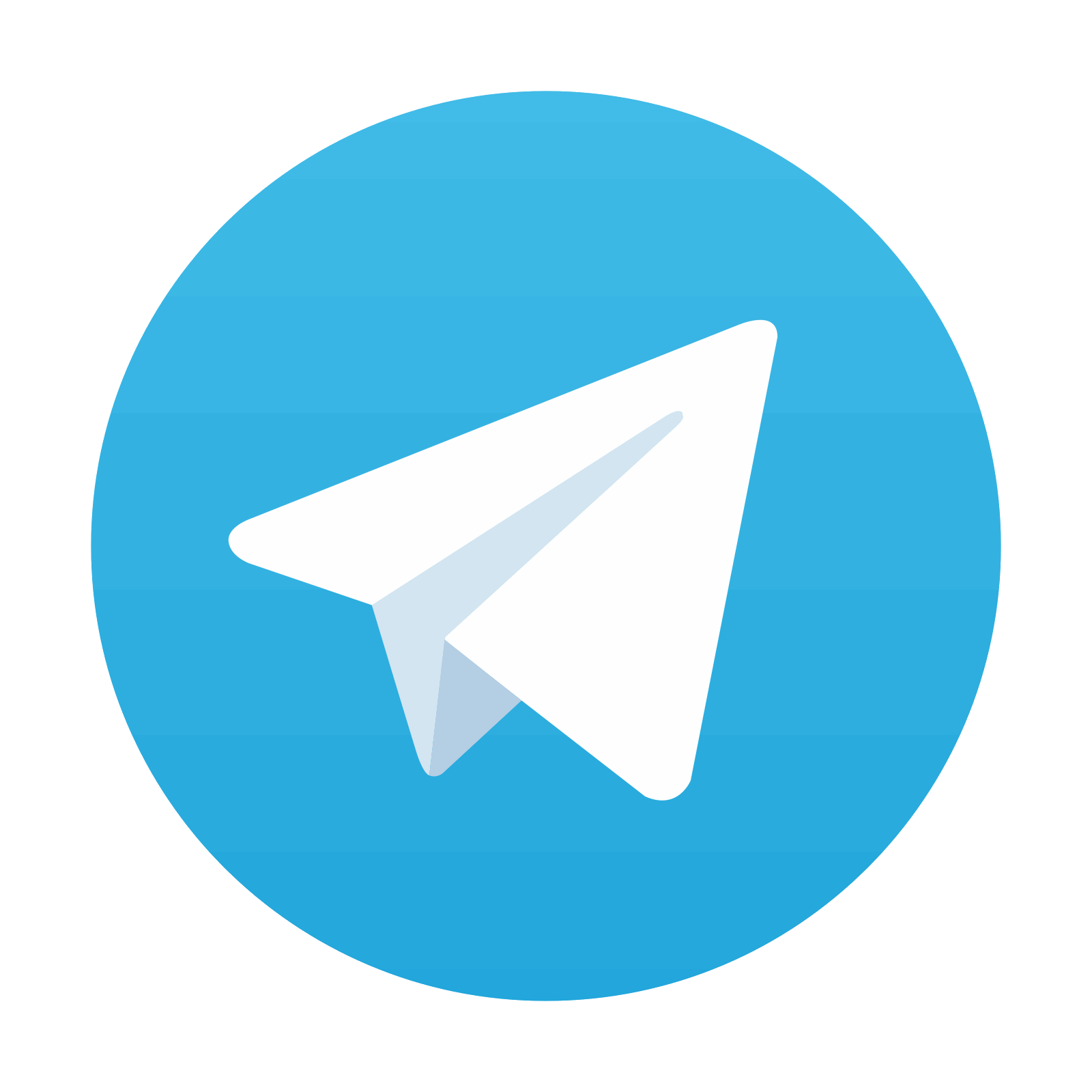
Stay updated, free articles. Join our Telegram channel

Full access? Get Clinical Tree
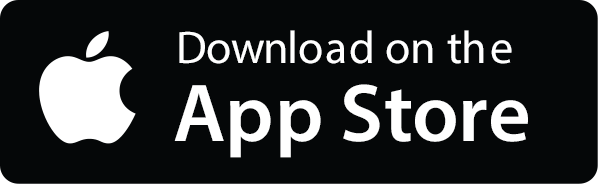
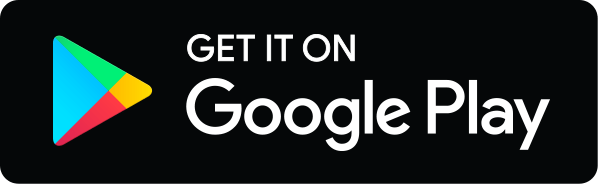