The mitral annulus is a pliable zone of discontinuous fibrous and muscular tissue that joins the left atrium and left ventricle and anchors the hinge portions of the anterior and posterior mitral leaflets.1-3 The annulus has two major collagenous structures: (1) the right fibrous trigone, which is part of the central fibrous body and is located at the intersection of the membranous septum, the tricuspid annulus, and the aortic annulus; and (2) the left fibrous trigone, which is located near the aortic annulus under the left aortic cusp (Fig. 34-1). The anterior mitral leaflet is in direct fibrous continuity with the aortic annulus under the left and noncoronary aortic valve cusps. The posterior half to two-thirds of the annulus, which subtends the posterior leaflet, is primarily muscular with little or no fibrous tissue.2
The mitral valve has two major leaflets, the larger anterior (or aortic) leaflet and the smaller posterior (or mural) leaflet; the latter usually contains three or more scallops separated by fetal clefts or subcommissures.4 The three posterior leaflet scallops are termed anterolateral (P1), middle (P2), and posteromedial (P3) scallops3 (Fig. 34-2). The portions of the leaflets near the free margin on the atrial surface are called the rough zone, with the remainder of the leaflet surface closer to the annulus being termed the smooth (or bare or membranous) zone. The ratio of the height of the rough zone to the height of the clear zone is 0.6 for the anterior leaflet and 1.4 for the posterior leaflet because the clear zone on the posterior scallops occupies only about 2 mm.4 The two leaflets are separated by the posteromedial and anterolateral commissures.
FIGURE 34-2
The operative view of the mitral valve is shown (left), and the corresponding “surgical view” obtained with real-time three-dimensional transesophageal echocardiography volume rendering (right). Images are from patient with type IIIb dysfunction (see text). A1, A2, A3, anterior mitral valve scallops; AL, anterolateral; P1, P2, P3, posterior mitral valve scallops; PM, posteromedial. (Reproduced with permission from O’Gara P, Sugeng L, Lang R, et al: The role of imaging in chronic degenerative mitral regurgitation, JACC Cardiovasc Imaging. 2008 Mar;1(2):221-237.)

The histologic structure of the leaflets includes three layers: (1) the fibrosa, the solid collagenous core continuous with the chordae tendineae; (2) the spongiosa, which is on the atrial surface and forms the leaflet leading edge (it consists of few collagen fibers but has abundant proteoglycans, elastin, and mixed connective tissue cells); and (3) a thin fibroelastic covering of most of the leaflets.2 On the atrial aspect of both leaflets, this surface (the atrialis) is rich in elastin. The ventricular side of the fibroelastic cover (the ventricularis) is much thicker and packed with elastin. The fibroelastic layers become thickened with advancing age as a result of elaboration of more elastin and more collagen formation; accelerated similar changes also accompany progression of myxomatous (degenerative or “floppy”) mitral valvular disease in young patients with Barlow syndrome. In addition to these connective tissue structures, mitral leaflets contain myocardium, smooth muscle, contractile valvular interstitial cells, and blood vessels, as well as both adrenergic and cholinergic afferent and efferent nerves.5-10 Leaflet contractile tissue is neurally controlled and plays a role in mitral valve function.11-14 The atrial surface of the anterior leaflet exhibits a depolarizing electrocardiogram spike shortly before the onset of the QRS complex, and the resulting contraction of leaflet muscle (which can be abolished by beta-blockade), along with contraction of smooth muscle and valvular interstitial cells, possibly aids leaflet coaptation before the onset of systole, as well as stiffens the leaflet in response to rising left ventricular (LV) pressure.6-8,15-23
The average mitral annular cross-sectional area ranges from 5.0 to 11.4 cm2 in normal human hearts (average is 7.6 cm2).24 The posterior annulus circumscribes approximately two-thirds of the mitral annulus. Annular area varies during the cardiac cycle and is influenced directly by both left atrial (LA) and LV contraction, size, and pressure.25,26 Varying by 20 to 40% during the cardiac cycle, annular size increases beginning in late systole and continues through isovolumic relaxation and into diastole; maximal annular area occurs in late diastole.25,27-29 Importantly, half to two-thirds of the total decrease in annular area occurs during atrial contraction (or presystolic); this component of annular area change is smaller when the PR interval is short and is abolished when atrial fibrillation or ventricular pacing is present. Annular area decreases further (if LV end-diastolic volume is not abnormally elevated) to a minimum in early to midsystole.25-27
The normal human mitral annulus is roughly elliptical, with greater eccentricity (ie, being less circular) in systole than in diastole.24-26,30 In three-dimensional (3D) space, the annulus is saddle shaped (or a hyperbolic paraboloid), with the highest point (farthest from the LV apex) located anteriorly in the middle of the anterior leaflet; this point is termed the fibrosa in the echocardiography literature and the saddle horn by surgeons. The low points are located posteromedially and anterolaterally in the commissures, and another less prominent high point is located directly posterior.25,31,32 During the cardiac cycle, annular regions adjacent to the posterior leaflet move toward (during systole) and away (during diastole) from the relatively immobile anterior annulus.25
The mitral annulus moves upward into the left atrium in diastole and toward the LV apex during systole; the duration, average rate, and magnitude of annular displacement correlate with (and perhaps influence) the rate of LA filling and emptying.25,27,28,33 The annulus moves slightly during late diastole (2 to 4 mm toward the left atrium during atrial systole). This movement does not occur in the presence of atrial fibrillation. The annulus moves a greater distance (3 to 16 mm toward the LV apex) during isovolumic contraction and ventricular ejection. This systolic motion, which aids subsequent LA filling, is related to the extent of ventricular emptying and is likely driven by LV contraction.25,28,33,34 Subsequently, the annulus moves very little during isovolumic relaxation but then exhibits rapid recoil toward the left atrium in early diastole. This recoiling increases the net velocity of mitral inflow by as much as 20%.28,35 Annular motion can be responsible for up to 20% of LV filling and ejection.36
The posterior mitral leaflet is attached to thinner chordae tendineae than the anterior leaflet, and its motion is restrained by chordae during both systole and diastole.4,37 Regions of both leaflets are concave toward the left ventricle during systole,38,39 but leaflet shape is complex, and anterior leaflet curvature near the annulus is convex to the left ventricle during systole, thus resulting in a sigmoid shape.29,31,32 Leaflet opening does not start with the free margin but rather in the center of the leaflet; leaflet curvature flattens initially and then becomes reversed (making the leaflet convex toward the left ventricle) while the edges are still approximated.29,39 The leading edge then moves into the left ventricle (like a traveling wave), and the leaflet straightens. The leaflet edges in the middle of the valve appear to separate before those portions closer to the commissures, and posterior leaflet opening occurs approximately 8 to 40 ms later.39,40 Early leaflet opening (e wave) is very rapid; once reaching maximum opening, the edges exhibit a slow to-and-fro movement (like a flag flapping in a breeze) until another less forceful opening impulse occurs, associated with the a wave.
Valve closure starts with the leaflet bulging toward the atrium at its attachment point to the annulus. The closure rate of the anterior leaflet is almost twice that of the posterior leaflet, thereby ensuring arrival of both cusps at their closed positions simultaneously (because the anterior leaflet is opened more widely than the posterior leaflet at the onset of ventricular systole).40 The anterior leaflet actually arrives at the plane of the annulus in a bulged shape (concave to the ventricle), but as the closing movement proceeds and the leaflet ascends toward the atrium, this curvature appears to run through the whole leaflet, from the annulus toward the edge, in a rolling manner. The leaflet edge is the last part of the leaflet to approach the annular plane.
Epicardial fibers in the left ventricle descend from the base of the heart and proceed inward at the apex to form the two papillary muscles, which are characterized by vertically oriented myocardial fibers.41,42 The anterolateral papillary muscle usually has one major head and is a more prominent structure; the posteromedial papillary muscle can have two or more subheads and is flatter.4 The posteromedial papillary muscle usually is supplied by the right coronary artery (or a dominant left circumflex artery in 10% of patients); the anterolateral papillary muscle is supplied by blood flow from both the left anterior descending and circumflex coronary arteries.3,41,43
The posteromedial and anterolateral papillary muscles give rise to chordae tendineae going to both leaflets4,44 (Fig. 34-3). Classically, the chordae are divided functionally into three groups.41,45 First-order chordae originate near the papillary muscle tips, divide progressively, and insert on the leading edge of the leaflets; these primary chordae prevent valve-edge prolapse during systole. The second-order chordae (including two or more larger and less branched “strut” chordae) originate from the same location and tend to be thicker and fewer in number.4,45 They insert on the ventricular surface of the leaflets at the junction of the rough and clear zones, which is demarcated by a subtle ridge on the leaflet corresponding to the line of leaflet coaptation. The second-order chordae (including the strut chordae) serve to anchor the valve, are more prominent on the anterior leaflet, and are important for optimal ventricular systolic function. Second-order chordae may arborize from large chordae that also give rise to first-order chordae. The third-order chordae, also called tertiary or basal chordae, originate directly from the trabeculae carneae of the ventricular wall, attach to the posterior leaflet near the annulus, and can be identified by their fan-shaped patterns.45 Additionally, distinct commissural chordae and cleft chordae exist in the commissures. In total, about 25 major chordal trunks (range 15 to 32) arise from the papillary muscles in humans, equally divided between those going to the anterior and posterior leaflets; on the other end, greater than 100 smaller individual chordae attach to the leaflets.45
FIGURE 34-3
Mitral valve and subvalvular apparatus. ALPM, anterolateral papillary muscle; Ant.Com.L., anterior commissural leaflet; Ant.Scal., anterior scallop; AoL, aortic leaflet; h, height of leaflet; l, length of attachment of leaflet; Lt.Trigone, left fibrous trigone; Mid.Scal., middle scallop; PMPM, posteromedial papillary muscle; Post.Com.L., posterior commissural leaflet; Post.Scal., posterior scallop; Right Trigone, right fibrous trigone; 1 = anterior main chorda; 2 = posterior main chorda; 3 = anterior paramedial chorda; 4 = posterior paramedial chorda; 5 = anterior paracommissural chorda; 6 = posterior paracommissural chorda; 7 = anterior commissural chorda; 8 = posterior commissural chorda; 9 = anterior cleft chorda; 10 = posterior cleft chorda. (Reproduced with permission from Sakai T, Okita Y, Ueda Y, et al: Distance between mitral anulus and papillary muscles: anatomic study in normal human hearts, J Thorac Cardiovasc Surg. 1999 Oct;118(4):636-641.)

During diastole, the papillary muscles form an inflow tract. During systole, they create an outflow tract that later becomes obliterated owing to systolic thickening of the papillary muscles, thereby augmenting LV ejection by volume displacement.42 The contribution of the papillary muscles to LV chamber volume is 5 to 8% during diastole but 15 to 30% during systole.42,46 The anterolateral and posteromedial papillary muscles contract simultaneously and are innervated by both sympathetic and parasympathetic (vagal) nerves.47,48 Excited synchronously with adjacent endocardium, the papillary muscles develop tension that significantly lags LV pressure and change length in concert with LV volume49-51 (Fig. 34-4). At end of isovolumic contraction, therefore, very little papillary tension and/or contraction is available to hold the closed leaflets in place against systolic LV pressure. This has led to the suggestion that the leaflets in the closed valve are virtually self-supporting, which, in turn, has led to the following hypotheses51: (1) The chordae, having precise lengths and anchored by papillary tips that maintain a fixed geometric relationship to the mitral annulus, guide the leaflets into precise geometric shapes and positions at the moment of valve closure. If these initial geometric conditions are set properly at this instant (ie, the complex leaflet curvatures are set precisely and all leaflet edges are positioned precisely with respect to one another in the LV chamber), the resulting leaflet assemblage becomes a near-rigid body whose geometry is virtually independent of flow and pressure throughout systole. (2) Subsequent papillary force development, transmitted through secondary chords, contributes about 10% to the total force of LV contraction, particularly in mid-to-late systole. This may underlie the findings, originally by Lillehei and colleagues52 but validated many times since, that clinical outcomes are improved by chordal preservation during mitral valve replacement. (3) Papillary muscle force and shortening, continuing during and after ventricular relaxation, plays an active role in valve opening. (4) Anterior leaflet strut chords, always in tension, prevent leaflet intrusion into the outflow tract (ie, systolic anterior motion of the mitral valve) and bias leaflet motion toward closure throughout diastole.53
FIGURE 34-4
(Left panel) Anterior and posterior papillary muscle force in relation to LVP. (Right panel) Anterior and posterior papillary muscle length in relation to LVP. (Reproduced with permission from Ingels NB, Jr. and Karlsson M. Mitral valve mechanics. Linkoping Sweden: Linkoping Press; 2015.)

Mitral stenosis generally is the result of rheumatic heart disease.54-62 Nonrheumatic causes of mitral stenosis or LV inflow obstruction include severe mitral annular and/or leaflet calcification in the elderly, congenital mitral valve deformities, malignant carcinoid syndrome, neoplasm, LA thrombus, endocarditic vegetations, certain inherited metabolic diseases, and those cases related to previous commissurotomy or an implanted prosthesis.55-59,63,64 A definite history of rheumatic fever can be obtained in only about 50 to 60% of patients; women are affected more often than men by a 2:1 to 3:1 ratio. Nearly always acquired before age 20, rheumatic valvular disease becomes clinically evident one to three decades later.
Globally, there are approximately 20 million cases of rheumatic fever, the greatest burden of which is evident as rheumatic heart disease with 282,000 new cases and 233,000 deaths annually.60-62,65 In the United States, Western Europe, and other developed countries, the prevalence of mitral stenosis has decreased markedly. The etiologic agent for acute rheumatic fever is group A beta-hemolytic streptococcus, but the specific immunologic and inflammatory mechanisms leading to the valvulitis are less clear.61,62,65-68 Streptococcal antigens cross react with human tissues, known as molecular mimicry, and may stimulate immunologic responses. Components implicated in the organism’s virulence include the hyaluronic acid capsule and the antigenic streptococcal M-protein and its peptides.61,65-67 Mimicry between streptococcal antigens and heart tissue proteins, combined with high inflammatory cytokine and low interleukin-4 production, leads to the development of autoimmune reactions and cardiac tissue damage.61,66,67 In acute rheumatic fever and rheumatic heart disease, M-protein can cross react with cardiac myosin, which induces T-cell-mediated injury of cardiac tissue and valves.61
In addition to valvular involvement, rheumatic heart disease is a pancarditis affecting to various degrees the endocardium, myocardium, and pericardium54,58,61 (Fig. 34-5). In rheumatic valvulitis, mitral valve involvement is the most common (isolated mitral stenosis is found in 40% of patients), followed by combined aortic and mitral valve disease, and least frequently, isolated aortic valve disease. Pathoanatomical characteristics of mitral valvulitis include commissural fusion, leaflet fibrosis with stiffening and retraction, and chordal fusion and shortening59 (Fig. 34-6). Leaflet stiffening and fibrosis can be exacerbated over time by increased flow turbulence. Valvular regurgitation can develop as a result of chordal fusion and shortening. The degree of calcification varies and is more common and of greater severity in men, older patients, and those with a higher transvalvular gradient.54 In some cases, rheumatic myocarditis results in LV dilatation and progressive heart failure.
FIGURE 34-6
Pathology of the mitral valve in mitral stenosis. Thickened, rigid nodular appearance of the mitral valve leaflets viewed from the atria (A) and ventricle (B). Calcium is present in the commissure and the commissures are fused, resulting in a valve shaped like a fish mouth. Subvalvular apparatus is thick, fused, and shortened (B, C). Healthy mitral valve leaflets (D). (Reproduced with permission from Chandrashekhar Y, Westaby S, Narula J: Mitral stenosis, Lancet. 2009 Oct 10;374(9697):1271-1283.)

Mitral annular calcification may progress to mitral sclerosis and stenosis in the elderly or those who are dialysis dependent.59,64 The anterior leaflet can become thick and immobile; LV inflow obstruction also results from calcification of the posterior mitral valve leaflet. Calcific protrusions into the ventricle and extension of the calcium into the leaflets further narrow the valve orifice, resulting in mitral stenosis.59,64 In these cases, the left ventricle typically is small, hypertrophied, and noncompliant.
In patients with mitral stenosis, an early, middle, and late diastolic transvalvular gradient is present between the left atrium and ventricle; as the degree of mitral stenosis worsens, a progressively higher gradient occurs, especially late in diastole.55,69,70 The average LA pressure in patients with severe mitral stenosis may be in the range of 15 to 20 mm Hg at rest, with a mean transvalvular gradient of 10 to 15 mm Hg.69,70 With exercise, the LA pressure and gradient rise substantially.
Another physiologic measurement in patients with mitral stenosis is the (derived) cross-sectional valve area, which is calculated from the mean transvalvular pressure gradient and cardiac output. The transvalvular pressure gradient is a function of the square of the transvalvular flow rate; for example, doubling the flow quadruples the gradient. Mitral transvalvular flow depends on cardiac output and heart rate. An increase in heart rate decreases the duration of transvalvular LV filling during diastole; the transvalvular mean gradient increases, and consequently, so does LA pressure.71 A high transvalvular gradient may be associated with a normal cardiac output. Conversely, if cardiac output is low, only a modest transvalvular gradient may be present.
Because of effective atrial contraction, the mean LA pressure in patients with mitral stenosis and normal sinus rhythm is lower than that in patients with atrial fibrillation.72,73 Sinus rhythm further augments flow through the stenotic valve, thereby helping to maintain adequate forward cardiac output. The development of atrial fibrillation decreases cardiac output by 20% or more; atrial fibrillation with a rapid ventricular response can lead to acute dyspnea and pulmonary edema.72
In patients with isolated mitral stenosis and restricted LV inflow, LV end-diastolic volume is normal or smaller than normal, and the end-diastolic pressure typically is low.55,74,75 The peak filling rate is reduced, as is stroke volume. Cardiac output thus is diminished as a result of inflow obstruction leading to underfilling of the ventricle rather than LV pump failure.76 During exercise, LV ejection fraction (LVEF) may increase slightly; however, LV filling is compromised by the shorter diastolic filling periods at higher heart rates, resulting in a smaller end-diastolic volume (or LV preload). Therefore, stroke volume and a blunted increase (or even decrease) in cardiac output can occur.75
Approximately 25 to 50% of patients with severe mitral stenosis have LV systolic dysfunction as a consequence of associated problems (eg, mitral regurgitation, aortic valve disease, ischemic heart disease, rheumatic myocarditis or pancarditis, and myocardial fibrosis) or internal constraint of a rigid mitral valve, reduced preload, and reflex increase in afterload.54,59,70,75 In these patients, LV end-systolic and diastolic volumes may be larger than normal. Diastolic dysfunction and abnormal chamber compliance are sometimes evident.59 Also, because right ventricular afterload increases as pulmonary hypertension develops in these patients, right ventricular systolic performance deteriorates.55,77
In patients with mitral stenosis who are in normal sinus rhythm, the LA pressure tracing is characterized by an elevated mean LA pressure with a prominent a wave.73 Because of the stenotic valve, coordinated LA contraction is important in maintaining adequate transvalvular flow.73 The high LA pressure gradually leads to LA hypertrophy and dilatation, atrial fibrillation, and atrial thrombus formation.54,75,76 The degree of LA enlargement and fibrosis does not correlate with the severity of the valvular stenosis partly because of the marked variation in duration of the stenotic lesion and atrial involvement by the underlying rheumatic inflammatory process.75 Disorganized atrial muscle fibers are associated with abnormal conduction velocities and inhomogeneous refractory periods. Premature atrial activation owing to increased automaticity or reentry eventually may lead to atrial fibrillation, which is present in more than half of patients with either pure mitral stenosis or mixed mitral stenosis and regurgitation.78 Major determinants of atrial fibrillation in patients with rheumatic heart disease include older age and larger LA diameter.78
In patients with mild-to-moderate mitral stenosis, pulmonary vascular resistance is not increased, and pulmonary arterial pressure may remain normal at rest, rising only with exertion or increased heart rate.69 In severe chronic mitral stenosis with elevated pulmonary vascular resistance, pulmonary arterial pressure is elevated at rest and can approach systemic pressure with exercise. A pulmonary arterial systolic pressure greater than 60 mm Hg significantly increases impedance to right ventricular emptying and produces high right ventricular end-diastolic and right atrial pressures.
LA hypertension produces pulmonary vasoconstriction, which exacerbates the elevated pulmonary vascular resistance.54,77 As the mean LA pressure exceeds 30 mm Hg above oncotic pressure, transudation of fluid into the pulmonary interstitium occurs, leading to reduced lung compliance. Pulmonary hypertension develops as a result of passive transmission of high LA pressure, pulmonary venous hypertension, pulmonary arteriolar constriction, and eventually, pulmonary vascular obliterative changes. Early changes in the pulmonary vascular bed may be considered protective in that the elevated pulmonary vascular resistance protects the pulmonary capillary bed from excessively high pressures. However, the pulmonary hypertension worsens progressively, leading to right-sided heart failure, tricuspid insufficiency, and occasionally, pulmonic valve insufficiency.55,77,60
Because of the gradual development of mitral stenosis, patients may remain asymptomatic for many years.59,60,69,79,80 Characteristic symptoms of mitral stenosis eventually develop and are associated primarily with pulmonary venous congestion or low cardiac output, for example, dyspnea on exertion, orthopnea, or paroxysmal nocturnal dyspnea and fatigue. With progressive stenosis (valve area between 1 and 2 cm2), patients become symptomatic with less effort. Correspondingly, the transmitral mean gradient is usually between 5 and 10 mm Hg at a normal heart rate.80 The mean pressure gradient is dependent on the transvalvular flow and diastolic filling period and will vary with changes in heart rate. When mitral valve area decreases to about 1 cm2, symptoms become more pronounced. As pulmonary hypertension and right-sided heart failure subsequently develop, signs of tricuspid regurgitation, hepatomegaly, peripheral edema, and ascites can be found.
As a result of high LA pressure and increased pulmonary blood volume, hemoptysis may develop secondary to rupture of dilated bronchial veins (or submucosal varices).59,77,79 Over time, pulmonary vascular resistance becomes higher, and the likelihood of hemoptysis decreases. Hemoptysis also may result from pulmonary infarction, which is a late complication of chronic heart failure. Acute pulmonary edema with pink frothy sputum can occur as a result of alveolar capillary rupture.
Systemic thromboembolism, occurring in approximately 20% of patients, may be the first symptom of mitral stenosis; recurrent embolization occurs in 25% of patients.79,81 The incidence of thromboembolic events is higher in patients with mitral stenosis or mixed mitral stenosis-mitral regurgitation than in those with pure mitral regurgitation. At least 40% of all clinically important embolic events involve the cerebral circulation, approximately 15% involve the visceral vessels, and 15% affect the lower extremities.79,82 Embolization to coronary arteries may lead to angina, arrhythmias, or myocardial infarction; renal embolization can result in hypertension.79 Factors that increase the risk of thromboembolic events include low cardiac output, LA dilatation, atrial fibrillation, LA thrombus, absence of tricuspid or aortic regurgitation, and the presence of echocardiographic “smoke” in the atrium, an indicator of stagnant flow. Patients with these risk factors should be anticoagulated.79,81,82 If an episode of systemic embolization occurs in patients in sinus rhythm, infective endocarditis, which is more common in mild than in severe mitral stenosis, should be considered.
Patients with chronic mitral stenosis are often thin and frail (cardiac cachexia), indicative of longstanding low cardiac output, congestive heart failure, and inanition.79 Heart size usually is normal, with a normal apical impulse on chest palpation. An apical diastolic thrill may be present. In patients with pulmonary hypertension, a right ventricular lift can be felt in the left parasternal region. Auscultatory findings include a presystolic murmur, a loud S1, an opening snap, and an apical diastolic rumble.59,79,83,84 The presystolic murmur, which occurs because of closing of the anterior mitral leaflet, is a consistent finding and begins earlier in those in sinus rhythm than in those in atrial fibrillation.84 S1 is accentuated in mitral stenosis when the leaflets are pliable but diminished in later phases of the disease when the leaflets are thickened or calcified. As pulmonary artery pressure becomes elevated, S2 becomes prominent.85 With progressive pulmonary hypertension, the normal splitting of S2 narrows because of reduced pulmonary vascular compliance. Other signs of pulmonary hypertension include a murmur of tricuspid and/or pulmonic regurgitation and an S4 originating from the right ventricle. Best heard at the apex, the early diastolic mitral opening snap is caused by sudden tensing of the pliable leaflets during valve opening and is absent when the leaflets are rigid or immobile.79,83 In mild mitral stenosis, the diastolic rumble is soft and of short duration; a long or holodiastolic murmur indicates severe mitral stenosis. The intensity of the murmur does not necessarily correlate with the severity of the stenosis; indeed, no diastolic murmur may be detectable in patients with severe stenosis, calcified leaflets, or low cardiac output.84
On chest radiography, LA enlargement is the earliest change found in patients with mitral stenosis; it is suggested by posterior bulging of the left atrium seen on the lateral view, a double contour of the right heart border seen on the posteroanterior film, and elevation of the left main stem bronchus.69 The overall cardiac size often is normal. Prominence of the pulmonary arteries coupled with LA enlargement may obliterate the normal concavity between the aorta and left ventricle to produce a straight left heart border. In the lung fields, pulmonary congestion may be recognized as distention of the pulmonary arteries and veins in the upper lung fields and pleural effusions. If mitral stenosis is severe, engorged pulmonary lymphatics are seen as distinct horizontal linear opacities in the lower lung fields (Kerley B lines).
The electrocardiogram is not accurate in assessing the severity of mitral stenosis and in many cases may be completely normal. In patients with severe mitral stenosis and normal sinus rhythm, LA enlargement is the earliest change (a wide notched P wave in lead II and a biphasic P wave in lead V1).59,69,86 Atrial arrhythmias are common in patients with advanced degrees of mitral stenosis. In those with pulmonary hypertension, right ventricular hypertrophy may develop and is associated with right axis deviation, a tall R wave in V1, and secondary ST-T-wave changes; however, the electrocardiogram is not a sensitive indicator of right ventricular hypertrophy or the degree of pulmonary hypertension.86
Echocardiography has become the primary diagnostic method for assessing mitral valve pathology and pathophysiology.3,59,60,80,87-89 Cross-sectional valve area and LA and LV dimensions can be quantified using two-dimensional (2D) transthoracic echocardiography (TTE). Best appreciated in the parasternal long-axis view, features of rheumatic mitral stenosis include reduced diastolic excursion of the leaflets (Carpentier type IIIa leaflet motion, see below) and thickening or calcification of the valvular and subvalvular apparatus (Fig. 34-7A). M-mode findings include thickening, reduced motion, and parallel movement of the anterior and posterior leaflets during diastole. Doppler echocardiography accurately determines peak and mean transvalvular mitral pressure gradients that correlate closely with cardiac catheterization measurements.87 To estimate mitral valve area, the pressure half-time (time required for the initial diastolic gradient to decline by 50%) has been employed; the more prolonged the half-time, the more severe is the reduction in orifice area.60,87 Using the pressure half-time determination, mitral valve area is equal to 220 (an empirical value) divided by the pressure half-time. Deriving mitral valve area using the pressure half-time method, however, generally has fallen out of favor. The mean mitral gradient at rest and with bicycle or supine exercise measured using Doppler echocardiography is more useful clinically than estimating mitral valve area; the simultaneous increase in right ventricular systolic pressure (estimated from continuous-wave or pulse-wave Doppler envelopes of the tricuspid regurgitation signal) during exercise is also revealing. The mitral separation index, which is the average of the maximum separation of the mitral leaflet tips in diastole in parasternal and apical four-chamber views, shows good correlation with mitral valve area measured by planimetry and pressure half-time and may be able to discriminate between hemodynamically significant and insignificant mitral stenosis.90 Transesophageal echocardiography (TEE) is better than the transthoracic approach for visualizing details of valvular pathology, such as valve mobility and thickness, subvalvular apparatus involvement, and extent of leaflet or commissural calcification.59,60,87,88
FIGURE 34-7
(A) Echocardiogram (long axis) transthoracic view of a patient with leaflet thickening and severe mitral stenosis caused by rheumatic heart disease. A thickened, stenotic valve separates an enlarged left atrium (right) and the left ventricle (left). (B) The immobility of the posterior leaflet and the doming of the anterior leaflet as typical morphological characteristics of rheumatic mitral valve disease are shown in a three-dimensional transesophageal image. The three-dimensional transesophageal images (left atrial aspect (C) and left ventricular aspect (D)) show the fusion of both commissures (red arrows). AML, anterior mitral leaflet; PML, posterior mitral leaflet. (Reproduced with permission from Wunderlich NC, Beigel R, Siegel RJ: Management of mitral stenosis using 2D and 3D echo-Doppler imaging, JACC Cardiovasc Imaging. 2013 Nov;6(11):1191-1205.)

Three-dimensional echocardiography facilitates spatial recognition of intracardiac structures and can evaluate cardiac valvular and congenital heart disease using both real-time 3D TTE and TEE images60,89,91 (Fig. 34-7B-D). The addition of color-flow Doppler to 3D echocardiography provides better visualization of regurgitant lesions. Measurements of LV volume using 3D echocardiography correlate tightly with measurements obtained using both contrast ventriculography and magnetic resonance imaging (MRI). Cardiac MRI technology remains inferior to echocardiography for depiction of valvular morphology and motion.92 Multidetector computed tomography (CT) may emerge as a technique that can evaluate both cardiac structure and function. Experience with gated multidetector CT has yielded good visualization of valve leaflet hinges, commissures, and the mitral annulus.93
Cardiac catheterization is not necessary to establish the diagnosis of mitral stenosis; however, it provides information regarding coronary artery status in older patients. Left-sided heart catheterization allows determination of LV end-diastolic pressure; right-sided heart catheterization is performed to measure cardiac index and the degree of pulmonary hypertension. Rarely, cardiac catheterization is used to evaluate the reversibility of severe pulmonary hypertension using pharmacologic interventions, including inhaled nitric oxide when indicated.
Whereas LV systolic function is used to predict the natural history and postoperative prognosis of patients with other valvular lesions, there are few data linking LV function to outcome in patients with mitral stenosis. Not surprisingly, the best indicator is related to the degree of clinical impairment. Untreated mitral stenosis is associated with a poor prognosis once severe symptoms occur.59 Percutaneous balloon valvuloplasty is the mainstay of treatment of mitral stenosis provided the anatomy is favorable59,60,80 (Fig. 34-8). Generally, the technique immediately doubles mitral valve area and decreases the gradient substantially in properly selected patients with rheumatic mitral stenosis. Approximately 90% of patients improve clinically if a valve area greater than 1.5 cm2 without significant regurgitation can be achieved.94 Surgical intervention (eg, open mitral commissurotomy or mitral valve replacement) substantially improves functional capacity and long-term survival of patients with mitral stenosis; 67 to 90% of patients are alive at 10 years.86,95-97
FIGURE 34-8
Treatment strategies for mitral stenosis. MVA, mitral valve area; NYHA, New York Heart Association functional class; PAWP, pulmonary artery wedge pressure; PBV, percutaneous balloon valvuloplasty. Severe mitral stenosis is rarely asymptomatic and, for such patients, we suggest use of the algorithm for assessment of patients with moderate disease. (Reproduced with permission from Chandrashekhar Y, Westaby S, Narula J: Mitral stenosis, Lancet. 2009 Oct 10;374(9697):1271-1283.)

Despite a higher operative risk for patients with severe pulmonary hypertension and right-sided heart failure, these individuals usually improve postoperatively with a reduction in pulmonary vascular pressures.55,98 If there is not excessive scarring of the subvalvular mitral apparatus, mitral valve replacement using chordal-sparing techniques can be performed in patients with rheumatic mitral valve disease, particularly those with mixed stenotic and regurgitant lesions; this results in a reduction in LV end-systolic and end-diastolic volumes and preservation of LV systolic pump performance.99,100 Alternatively, the valve and the entire subvalvular apparatus have to be resected during mitral valve replacement when the disease process is very advanced and all structures are densely calcified and extremely scarred, which frequently is the case in older patients.
Mitral stenosis generally is caused by rheumatic heart disease. With worsening mitral stenosis, a progressively higher transvalvular pressure gradient develops. Mitral transvalvular flow depends on cardiac output and heart rate; an increase in heart rate decreases the duration of transvalvular filling during diastole and reduces forward cardiac output, causing symptoms. In mild-to-moderate mitral stenosis, pulmonary vascular resistance may not be elevated; pulmonary arterial pressure may be normal at rest and rise only with exercise or increased heart rate. In patients with severe mitral stenosis with elevated pulmonary vascular resistance, the pulmonary arterial pressure usually is high at rest. Characteristic symptoms of mitral stenosis are associated with pulmonary venous congestion and/or low cardiac output. Echocardiography remains the best technique for assessing mitral valve pathology. Percutaneous or surgical intervention can improve functional capacity and long-term survival of patients with mitral stenosis.
The functional competence of the mitral valve relies on proper, coordinated interaction of the mitral annulus and leaflets, chordae tendineae, papillary muscles, left atrium, and left ventricle, known as the valvular-ventricular complex.41,101-104 Normal LV geometry and alignment of the papillary muscles and chordae tendineae permit leaflet coaptation and prevent prolapse during ventricular systole. Dysfunction of any one or more of the components of this valvular-ventricular complex can lead to mitral regurgitation. Important causes of mitral regurgitation include myxomatous degeneration (“primary” mitral regurgitation), ischemic heart disease with ischemic mitral regurgitation (IMR), dilated cardiomyopathy [for which the general term functional mitral regurgitation (FMR) is used], rheumatic valve disease, mitral annular calcification, infective endocarditis, congenital anomalies, endocardial fibrosis, myocarditis, and collagen-vascular disorders.54,57,58,105-108 IMR is considered a specific subset of FMR, which is now termed “secondary” mitral regurgitation. Acute mitral regurgitation also may be the result of ventricular dysfunction from rapidly developing cardiomyopathy, such as Takotsubo cardiomyopathy, in which the mitral regurgitation is caused by LV outflow tract obstruction and systolic anterior motion of the mitral valve from apical ballooning.109
In general, four types of structural changes of the mitral valve apparatus may produce regurgitation: leaflet retraction from fibrosis and calcification, annular dilatation, chordal abnormalities (including rupture, elongation, or shortening), and LV systolic dysfunction with or without papillary muscle involvement.41,110-116 Carpentier classified mitral regurgitation according to three main pathoanatomic types based on mitral leaflet motion: normal leaflet motion (type I), leaflet prolapse or excessive motion (type II), and restricted leaflet motion (type III).110,111 Type III is further subdivided into types IIIa and IIIb based on leaflet restriction during diastole (type IIIa), as seen in rheumatic disease, or during systole (type IIIb), which is typically seen in IMR (Fig. 34-9). Mitral regurgitation with normal leaflet motion can result from annular dilatation, often secondary to LV dilatation, for example, patients with dilated cardiomyopathy or ischemic cardiomyopathy. Normal leaflet motion also includes patients with leaflet perforation due to endocarditis or a congenital cleft. Leaflet prolapse typically results from a floppy mitral valve with chordal elongation and/or rupture, but rarely also can be seen in patients with coronary artery disease who have papillary muscle elongation or rupture. Mitral regurgitation caused by restricted leaflet motion is associated with rheumatic valve disease (types IIIa and IIIb), ischemic heart disease (IMR with type IIIb restricted systolic leaflet motion secondary to apical tethering or tenting), and dilated cardiomyopathy (type IIIb).111,112
FIGURE 34-9
Carpentier’s functional classification of the types of leaflet and chordal motion associated with mitral regurgitation. In type I, the leaflet motion is normal. Type II mitral regurgitation is caused by leaflet prolapse or excessive motion. Type III (restricted leaflet motion) is subdivided into restriction during diastole (A) or systole (B). Type IIIb typically is seen in patients with ischemic mitral regurgitation. The course of the leaflets during the cardiac cycle is represented by the dotted lines. (Modified with permission from Carpentier A: Cardiac valve surgery—the “French correction,” J Thorac Cardiovasc Surg. 1983 Sep;86(3):323-337.)

Myxomatous degeneration of the mitral valve, also known as floppy mitral valve or mitral valve prolapse and considered primary mitral regurgitation, is the most common cause of mitral regurgitation in patients undergoing surgical evaluation in the United States.41,108,117-120 Afflicting 2 to 3% of the general population, mitral valve prolapse is both acquired (fibroelastic deficiency in older patients) and congenital or heritable, with excess spongy, weak fibroelastic connective tissue constituting the leaflets and chordae tendineae (Barlow’s valve in younger patients)54,80,108,121,122 (Fig. 34-10). Associated with connective tissue disorders, such as Marfan syndrome, Ehlers-Danlos, Loeys-Dietz syndrome, osteogenesis imperfecta, pseudoxanthoma elasticum, and aneurysm-osteoarthritis syndrome, mitral valve prolapse appears to be the result of multiple genetic pathways and can be sporadic or familial with autosomal dominant and X-linked inheritance.108,123,124 Although three different loci on chromosomes 16, 11, and 13 (autosomal dominant) are linked to mitral valve prolapse, no specific gene has yet been incriminated.108,123,124 Also, a locus on chromosome X cosegregates with a rare form of mitral valve prolapse called X-linked myxomatous mitral valve dystrophy.108,124 Upregulation of transforming growth factor-beta and the mutations of the cytoplasmic protein filamins appear to have a pivotal role in various pathways in the pathogenesis of mitral valve prolapse.108 Because the dysfunctional valve is the disease, myxomatous degeneration of the mitral valve is considered primary mitral regurgitation.80,125
Some degree of mitral valve prolapse is seen echocardiographically in 5 to 6% of the female population.121,126 The risk of endocarditis is increased only if valvular regurgitation is present and accompanied by a murmur. Mitral valve prolapse appears to be more widespread in women, but severe mitral regurgitation resulting from mitral valve prolapse is more common in men. Subtle signs of heart failure, usually manifest as declining stamina and fatigue, may be the presenting complaint in 25 to 40% of symptomatic patients with mitral valve prolapse. As strictly defined originally by John Barlow, “Barlow’s syndrome” includes prolapse of the posterior leaflet and chest pain, and occasionally palpitations, syncope, and dyspnea; in younger patients, the initial clinical sign is a midsystolic nonejection click, which later evolves into a click followed by a late systolic murmur.121 This latter scenario is seen typically in young patients with Barlow’s valves, in which large amounts of excessive leaflet tissue and marked annular dilatation are coupled with extensive billowing of both leaflets. Because many patients have had symptoms consistent with mitral valve prolapse syndrome for many years prior to developing significant mitral regurgitation, in certain patients, beta-adrenergic receptor polymorphisms may play a role in the pathogenesis of symptoms.127
Pathologically, the atrial aspect of the prolapsing mitral leaflet often is thickened focally, whereas the changes on the ventricular surface of the leaflet consist of connective tissue thickening primarily on the interchordal segments with fibrous proliferation into adjacent chordae and onto the ventricular endocardium.54,108,121,122 Histologically, elastic fiber and collagen fragmentation and disorganization are present, and acid mucopolysaccharide material accumulates in the leaflets. Myxomatous degeneration commonly involves the annulus, resulting in annular thickening and dilatation. All these changes are pronounced in young patients with Barlow’s valves but can be minimal in older subjects with fibroelastic deficiency, in whom the noninvolved posterior leaflet scallops and anterior leaflet are normal and thin.80 It is important to recognize that these two distinct varieties of mitral valve prolapse exist and can be segregated on clinical grounds, even if pathologists have difficulty discriminating between the two, because the repair techniques differ in major ways. Many centers like the Mayo Clinic encounter mainly elderly patients with coronary artery disease and fibroelastic deficiency (78% of their surgical “flail” leaflet surgical population were greater than 60 years old and/or required concomitant coronary artery bypass grafting), in whom the valvular pathology is limited, and simple repair techniques, such as the small McGoon triangular excision of the middle scallop of the posterior leaflet, are applicable and work well.128 In contrast, other institutions may attract younger patients who have Barlow’s valves or severely myxomatous mitral valves, circumstances that demand much more extensive repair techniques and different expertise.
Only 5 to 10% of patients with mitral valve prolapse progress to develop severe mitral regurgitation, and they can remain relatively asymptomatic for many years.121 Mechanisms accounting for severe mitral regurgitation in those with mitral valve prolapse include annular dilatation and rupture or elongation of the first-order chordae (58%), annular dilatation without chordal rupture (19%), and chordal rupture without annular dilatation (19%).122 Chordal rupture, probably related to defective collagen, underlying papillary muscle fibrosis or dysfunction, or bacterial endocarditis, typically is the culprit when mitral regurgitation develops acutely in patients without any previous symptoms of heart disease or suddenly becomes worse in those with known mitral valve prolapse.41,54,56,57,106,108,129 Chordal rupture is evident in 14 to 23% of surgically excised purely regurgitant valve specimens; in 73 to 93% of these patients, the underlying pathology is degenerative or floppy mitral valves.56,57,106 Posterior chordal rupture, usually subtending just the middle scallop, is the most frequent finding, followed by anterior chordal rupture and then combined anterior and posterior chordal rupture.56,57,106
FMR is the result of incomplete mitral leaflet coaptation in the setting of LV systolic dysfunction and dilatation with or without annular dilatation (eg, dilated cardiomyopathy or ischemic cardiomyopathy) and is thus now termed secondary mitral regurgitation.80,113-116,125 LV systolic dysfunction and dilatation also may be associated with longstanding mitral regurgitation caused by severe chronic LV volume overload. Most commonly, the etiology of nonischemic cardiomyopathy is unknown or idiopathic; the second most common cause is advanced valvular disease. FMR occurs in 40% of patients with heart failure caused by dilated cardiomyopathy.116
IMR, a subset of FMR, is becoming widely appreciated as the population ages and more patients survive acute myocardial infarction. In those with acute infarction, IMR occurs in approximately 15% of patients with anterior wall involvement and up to 40% of patients with an inferior infarct.56,57,130 Generally, the severity of mitral regurgitation is related to the size of the area of LV akinesia or dyskinesia. The pathophysiology of IMR can be attributed to changes in global and regional LV function or geometry, alterations in mitral annular geometry, abnormal leaflet motion and malcoaptation, increased interpapillary distance, and papillary muscle malalignment leading to apical tethering of the leaflets with restricted systolic leaflet motion (type IIIb).110,113,114,130-139 Because of the interdependence of the elements constituting the valvular-ventricular complex in IMR, perturbation of any component, such as LV systolic function and geometry, annular geometry, leaflet motion and morphology, and papillary and chordal relationship, may result in mitral regurgitation.
Although LV dilatation and dysfunction are less pronounced in the setting of inferior myocardial infarction than that affecting the anterior wall, the incidence and severity of mitral regurgitation are greater in patients with inferior infarction.56,57,130,132,134 Over time, as the left ventricle dilates and changes shape after the ischemic event (postinfarction remodeling), the degree of IMR progresses.115,134,140 Geometric changes associated with ventricular remodeling, such as posteromedial papillary muscle dislocation in the lateral axis, may lead to leaflet tenting, as reflected by a larger distance from the middle of the anterior annulus (saddle horn on echocardiography) to the posteromedial papillary muscle tip, and increased annular diameter.115,135,136,140 At the ventricular level, myocardial infarction associated with chronic IMR is associated with greater adverse perturbations in LV systolic torsion and diastolic recoil than myocardial infarction without chronic IMR.141 These abnormalities may be linked to more LV dilatation, which possibly reduces the effectiveness of fiber shortening on torsion generation. Altered LV torsion and recoil may contribute to the “ventricular disease” component of chronic IMR, with increased gradients of myocardial oxygen consumption adversely affecting cardiac efficiency and impaired early diastolic filling.141 Additionally, in a subacute model of IMR (less than 7 weeks), there is an equivalent increase in LV end-diastolic volume in those with mild mitral regurgitation compared to those with more severe mitral regurgitation, coupled with unchanged end-diastolic and end-systolic remodeling strains, including systolic circumferential, longitudinal, and radial strains; these findings in aggregate argue against an intracellular (cardiomyocyte) mechanism for the LV dysfunction.142 Instead, differences in subepicardial shear strains suggest a causal role of altered interfiber interactions, and the mechanical impairment may be in extracellular matrix between the fibers and the microtubules in the cytoskeleton that couple cardiomyocyte shortening to LV wall thickening.142
In IMR, there may be increased mitral annular area, annular stretching and flattening (involving both anterior and posterior components of the annulus), increased septal-lateral annular dimension (also termed the anteroposterior axis), which is perpendicular to the line of leaflet coaptation), lateral displacement of posteromedial papillary muscle, and apically tethered posterior leaflet with restricted closing motion, any of which may contribute to leaflet malcoaptation132,133,135,136 (Fig. 34-11). The septal-lateral annular dilatation and diminished LV systolic function determine mitral systolic tenting area, which in turn is predictive of the severity of the IMR.132 LV dilatation and larger annular dimensions after inferior myocardial infarction require the mitral leaflets to cover more area during closing, exceeding their normal redundancy or “reserve,” which is exacerbated by restricted leaflet closure (type IIIb motion) owing to apical leaflet tenting. Additionally, the distinctive saddle shape of the normal annulus, which becomes accentuated during systole, is eliminated which makes it flatter and suggests an association between maintaining the saddle shape and valvular competence.132,143,144 Furthermore, in patients with IMR, the anterior and posterior annular perimeters and annular orifice area (9.1 cm2 compared with 5.7 cm2 normally) are increased accompanied by an increase in the intertrigonal (anterior) annular distance and restriction of annular motion.136
FIGURE 34-11
Average mitral annular area (in cm2) before (blue squares) and during (red squares) acute LV ischemia induced by balloon occlusion of either the LAD artery (top), the dLCx coronary artery (middle), or the pLCx coronary artery (bottom). A 650-ms time interval centered at end-diastole (t = 0) is shown. dLCx, distal to second obtuse marginal artery; LAD, proximal left anterior descending artery; MA, mitral annular; pLCx, proximal to second obtuse marginal artery. (Reproduced with permission from Timek TA, Lai DT, Tibayan F, et al: Ischemia in three left ventricular regions: Insights into the pathogenesis of acute ischemic mitral regurgitation, J Thorac Cardiovasc Surg. 2003 Mar;125(3):559-569.)

Acute IMR from proximal left circumflex artery occlusion experimentally results in delayed valve closure in early systole (termed leaflet loitering) and increased leaflet edge separation throughout ejection in three leaflet coaptation sites across the valve, specifically near the anterior commissure, the valve center, and near the posterior commissure.131,133 In addition, there is lateral displacement of the central scallop of the posterior leaflet, suggesting that interscallop malcoaptation and septal-lateral annular dilatation, can contribute to IMR in certain circumstances.139 Clinically, chronic IMR is associated with apical systolic restriction of the posterior leaflet, thereby effectively preventing competent valve closure; this restriction results in a posteriorly directed eccentric MR jet due to anterior leaflet “pseudoprolapse.” Chronic IMR is also associated with posterior leaflet displacement in the posterior direction and lateral displacement of both leaflets. When the position of each leaflet edge is assessed independently, the anterior leaflet is not displaced apically after inferior infarction, although with more time and further remodeling, apical restriction of this leaflet may occur.135 A strong echocardiographic determinant of leaflet tenting height is the distance from the tips of the papillary muscles to the saddle horn of the anterior annulus; LV end-diastolic volume is only weakly correlated with tenting height.145 Recent human observations have revealed that in some patients with IMR/FMR, there is growth or elongation of the leaflets associated with leaflet thickening that compensates for the larger orifice area and minimizes the amount of mitral regurgitation. In others, however, the leaflets do not become larger and cannot coapt normally across the large orifice, which causes more leaks.146
In the past, the leaflet morphology in patients with FMR was considered normal, but further analyses have shown the leaflets to be biochemically different, with extracellular matrix changes associated with altered cardiac dimensions.147,148 In recipient hearts obtained at time of transplantation, mitral leaflets have up to 78% more deoxyribonucleic acid, 59% more glycosaminoglycans, 15% more collagen, but 7% less water than autopsy control leaflets.147,148 Radially and circumferentially oriented anterior mitral leaflet strips from failing hearts are 50 to 61% stiffer and less viscous.148 Thus, the mitral leaflets in heart failure have altered intrinsic structural properties, suggesting that the permanently distended and fibrotic tissue is unable to stretch sufficiently to cover the valve orifice and that mitral regurgitation in these patients is not purely functional.147,148
The papillary-annular distances in the LV long axis remain relatively constant in normal hearts throughout the cardiac cycle.114 During acute ischemia, however, these distances change, which reflects repositioning or dislocation of the papillary muscle tips with respect to the mitral annulus. This process can also contribute to apical tenting of the leaflets during systole.110,114,135,149,150 With proximal circumflex artery occlusion and resulting IMR in an ovine model, the interpapillary distance and LV end-diastolic volume both increase. There is also increased mitral annular area and displacement of both (but predominantly the posteromedial) papillary muscle tips away from the septal annulus throughout ejection and at end-systole.135,149 Posteromedial papillary muscle tip displacement probably results from failure of the ischemic papillary muscle to shorten during systole, lengthening of the ischemic papillary muscle over time, and dyskinesia of the ischemic LV wall subtending the papillary muscle. Since posterior papillary muscle displacement in the apical and posterior directions also occurs in sheep that did not develop substantial degrees of IMR, the additional posteromedial papillary muscle displacement in the lateral direction is a dominant factor in the development of IMR.135 In the setting of posterolateral ischemia, a larger distance from the papillary muscle tips to the midseptal annulus is an important determinant of mitral regurgitant jet area and volume.130,135 The nonischemic anterolateral papillary muscle may also play a role in apical leaflet restriction because this papillary muscle is displaced apically at end-systole relative to baseline. In sheep with mitral regurgitation, posteromedial papillary muscle tethering distance, papillary muscle depth, and papillary muscle angle are unchanged, and the anterolateral papillary muscle depth and papillary muscle angle decrease with decreasing ejection fraction.149 Reduced systolic shortening of either papillary muscle in isolation, on the other hand, does not result in mitral regurgitation; thus, the previous notion that “papillary muscle dysfunction” by itself is responsible for IMR is not correct. In fact, papillary muscle dysfunction paradoxically can decrease mitral regurgitation resulting from inferobasal ischemia by reducing leaflet tethering, which improves leaflet coaptation.151 Further insult to the LV wall underlying the papillary muscles is likely needed before valvular incompetence occurs. Acute IMR induced by experimental posterior LV ischemia is associated with chordal and leaflet tethering at the nonischemic commissural portion of the mitral valve and a paradoxical decrease of the chordal forces and relative prolapse at the ischemic commissural site.137 Based on cardiac MRI of humans with ischemic heart disease, the loss of lateral shortening of the interpapillary muscle distance tethers the leaflet edges and impairs their systolic closure, resulting in mitral regurgitation.150 Thus, it may be a combination of systolic annular dilatation and shape change and altered posteromedial and anterolateral papillary muscle position and motion that contributes to incomplete leaflet coaptation and IMR.
Papillary muscle dysfunction in patients with ischemic heart disease has been thought to contribute to mitral regurgitation, although the significance of its role in IMR remains in question.41,43,56,57,114,135,149,151 The papillary muscles are particularly susceptible to ischemia, more so the posteromedial papillary muscle (supplied only by the posterior descending artery in 63% of cases) than the anterolateral papillary muscle (supplied by both the left anterior descending and the circumflex arteries in 71% of cases).41,43 Hence, myocardial infarction leading to papillary muscle dysfunction occurs more frequently with the posteromedial papillary muscle after an inferior myocardial infarction. Although papillary muscle necrosis can complicate myocardial infarction, frank rupture of a papillary muscle is rare. Total papillary muscle rupture usually is fatal as a result of severe mitral regurgitation and LV pump failure; survival long enough to reach the operating room in reasonable condition is possible with rupture of one or two of the subheads of a papillary muscle, which is associated with a lesser degree of acute mitral regurgitation. Papillary muscle rupture usually occurs 2 to 7 days after myocardial infarction; without emergency operation, approximately 50 to 75% of such patients may die within 24 hours.152,153
With decreasing incidence in the United States, rheumatic fever remains a common cause of mitral regurgitation in developing countries.41,56-58,105-107,154 It is unknown why rheumatic fever leads to valvular stenosis in some patients and pure regurgitation in others. The pathoanatomical changes of the purely regurgitant rheumatic valve differ from those in a stenotic valve. In chronic rheumatic mitral regurgitation, the valves have diffuse fibrous thickening of the leaflets with minimal calcific deposits and relatively nonfused commissures; chordae tendineae usually are not extremely thickened or fused.56-58 There also may be shortening of the chordae tendineae, fibrous infiltration of the papillary muscle, and asymmetric annular dilatation primarily in the posteromedial portion. During the first episodes of rheumatic fever (average age is 9 years), patients may develop acute mitral regurgitation, which is more frequently related to annular dilatation and prolapse of the anterior or posterior mitral valve leaflet.58,154 Those with anterior leaflet prolapse tend to improve with medical management; however, those with prolapse of the posterior leaflet have a less favorable outcome and often require early surgical repair.154
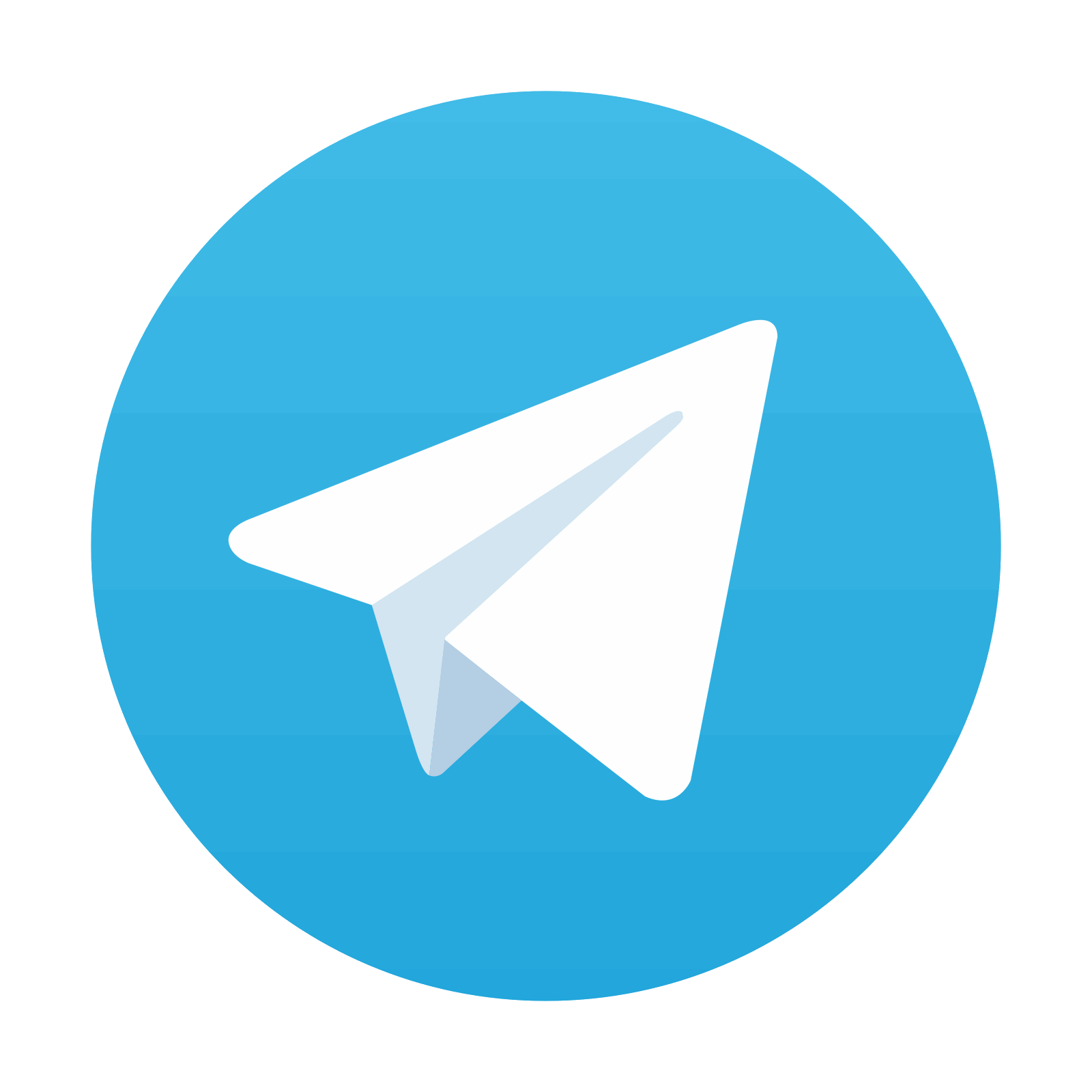
Stay updated, free articles. Join our Telegram channel

Full access? Get Clinical Tree
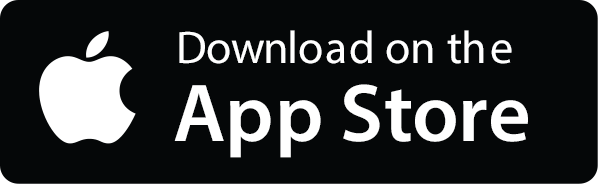
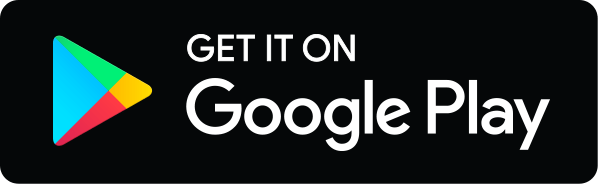