scavenger receptor class B type I, and aqueous diffusion—and captured by native, cholesterol-poor high-density lipoproteins (HDLs) in the reverse cholesterol transport process.
![]() FIGURE 95.1 Pathophysiology of atherosclerosis. The figure is divided into four steps in the atherogenic process and its characteristics: (A) initiation: endothelial activation, subendothelial apoB-containing lipoprotein retention and fatty streak formation; (B) progression: migration and proliferation of smooth muscle cells, extracellular matrix secretion, and plaque development; (C) stability: a developed thick fibrous cap, formation of the necrotic core and calcification; (D) vulnerable plaque/rupture: progression of necrotic core because of secondary necrosis, release of oxidation products, MMP-3, proteinases and inflammatory mediators, thinning of the fibrous cap promotion, and plaque rupture. ECM, extracellular matrix; FGF, fibroblast growth factor; ICAM, intercellular adhesion molecule; LDL, low-density lipoprotein cholesterol; MMP3, matrix metalloproteinase 3; NO, nitric oxide; PDGF, platelet-derived growth factor; ROS, reactive oxygen species; SMC, smooth muscle cell; TGF-β, transforming growth factor beta; TLR, toll-like receptor; VCAM, vascular cell adhesion molecule. |
However, with atheroma progression, the process of clearing dead cells and associated cellular debris (called efferocytosis) becomes dysfunctional, thereby accumulation of cellular debris and deposition of cholesterol crystals contribute to the formation of the necrotic core. Smooth muscle cell migration and proliferation continue, building additional cell layers that result in a thick fibrous cap that covers the necrotic core. This cover protects the plaque against rupture and its consequent core exposure to prothrombotic factors.
TABLE 95.1 Causes of Endothelial Damage | ||||||||||||||||||
---|---|---|---|---|---|---|---|---|---|---|---|---|---|---|---|---|---|---|
|
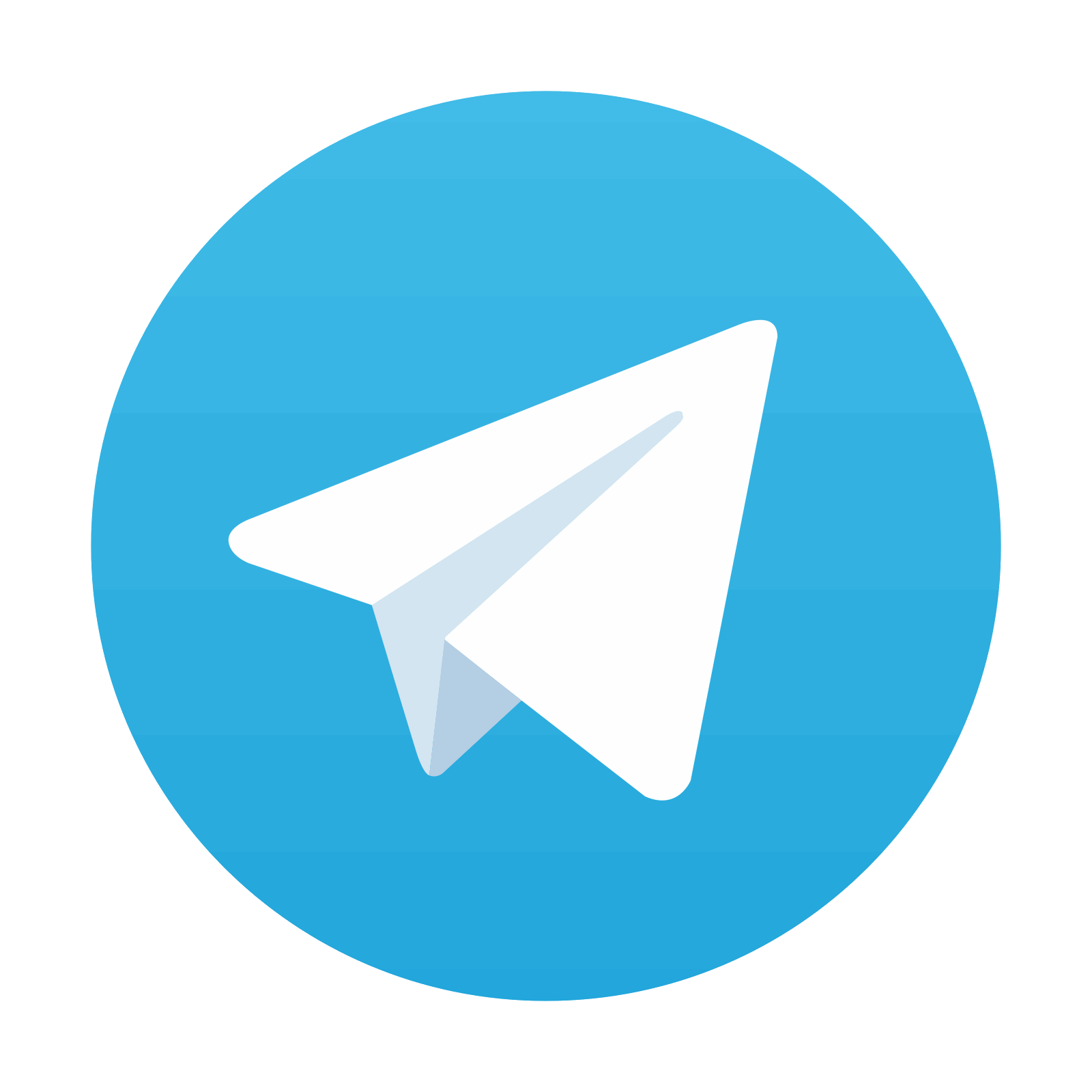
Stay updated, free articles. Join our Telegram channel

Full access? Get Clinical Tree
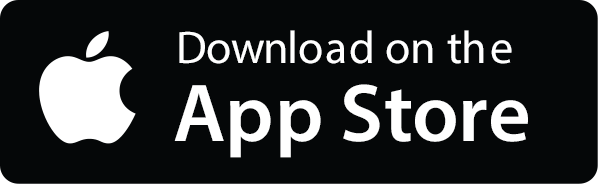
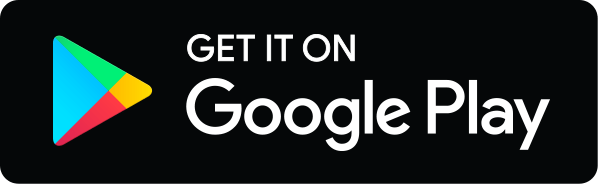