The aortic valve (AV) is a semilunar valve positioned at the end of the left ventricular outflow tract (LVOT) between the left ventricle and aorta. Proper functioning of this valve is critical in maintaining efficient cardiac function. This chapter explores the anatomical and physiologic properties of the AV.
Embryologic development of the AV is closely associated with development of the LVOT. In the primary heart tube, blood travels from the primitive ventricle into the bulbous cordis and out from the aortic roots. The midportion of the bulbous cordis, the conus cordis, develops into the outflow tracts of the ventricles. The distal portion of the bulbous cordis, the truncus arteriosus (TA), develops into the proximal portion of the aorta and pulmonary arteries. During the fifth week of development, pairs of opposing swellings appear in the conus cordis and TA (Fig. 26-1). These conotruncal, or bulbar, ridges grow toward each other and fuse to form the aorticopulmonary (AP) septum. The AP septum divides the conus cordis into the left and right ventricular outflow tracts and the TA into the ascending aorta and pulmonary trunk.
When partitioning of the TA is nearly completed, the AV begins to develop from three swellings of subendocardial tissue (see Fig. 26-1). Two swellings arising from the fused truncal ridges develop into the right and left AV leaflets. A third dorsal swelling develops into the posterior leaflet. These swellings are reshaped and hollowed out to form the three thin-walled cusps of the fully developed AV. The pulmonary valve develops in a similar manner.
FIGURE 26-1
Schematic representation of development of the left and right ventricular outflow tracts and aortic and pulmonary valves. (Top left) Cross-section through the cordis showing the conotruncal ridges beginning to develop. (Top right) Aorticopulmonary septum forming from fusion of the conotruncal ridges and subendocardial swellings beginning to develop into the aortic and pulmonary valve leaflets. (Bottom left) Left and right ventricular outflow tracts separated by the aorticopulmonary septum and further development of the subendocardial swellings. (Bottom right) Aortic and pulmonary valves in the adult. (Reproduced with permission from Cleveland Clinic, Cleveland, OH.)

The AV separates the terminal portion of the LVOT from the aorta. The normal AV consists of three semilunar leaflets or cusps projecting outward and upward into the lumen of the ascending aorta (Fig. 26-2). The space between the free edge of each leaflet and the points of attachment to the aorta comprise the sinuses of Valsalva. Because the coronary arteries arise from two of the three sinuses, the sinuses and the respective leaflets are named the right coronary, left coronary, and posterior (noncoronary) sinuses and leaflets. The ostia of coronary arteries arise from the upper part of the sinuses, with the ostium of the left coronary artery positioned slightly higher than the ostium of the right. The leaflets are separated from one another at the aorta by the right-left, right-posterior, and left-posterior commissures (Fig. 26-3). The area adjacent to the left-posterior commissure is the fibrous continuity, which interconnects the aorta and the mitral valve annulus. The area beneath this commissure is the aortomitral curtain, which is an important anatomical landmark for root enlargement procedures. The posterior leaflet attaches above the posterior diverticulum of the LVOT and opposes the right atrial wall. The right-posterior commissure is positioned directly above the penetrating atrioventricular bundle and membranous septum. The right-left commissure opposes the posterior commissure of the pulmonary valve and the two associated aortic cusps oppose the right ventricular infundibulum. The lateral part of the left coronary sinus is the only part of the AV that is not closely related to another cardiac chamber and is in direct relationship with the free pericardial space.
The AV leaflets meet centrally along a line of coaptation, at the center of which are the thickened nodules of Arantius. Because of the semilunar shape of the leaflets, the AV does not have a true annulus in the traditional sense of a ring-like attachment. Instead, the leaflets have semilunar attachments along a hollow cylinder or cuff of tissue interconnecting the left ventricular (LV) chamber and the proximal aorta (Fig. 26-4).1 The distal border of the cuff is the sinotubular junction, which is defined by imaginary lines connecting the commissures. The proximal border is the ventriculoarterial (VA) junction, which has both hemodynamic and anatomic parts. The hemodynamic VA junction is marked by the semilunar attachments of the leaflets, whereas the anatomic VA junction is marked by the circular attachment of the proximal aorta and the muscular and membranous ventricular septums.
The valve leaflets consist of three layers of endothelially invested connective tissue of distinctly different density and composition. There is no demarcation between the outer layers of the aortic and ventricular sides of the leaflets and outer layers of the corresponding aortic and ventricular walls; that is, leaflet endothelial cells form a continuum with aortic and ventricular endothelial cells (Fig. 26-5).2,3 Beneath the endothelium, there are extensions of the aortic intima and ventricular endocardium, termed the arterialis and ventricularis, respectively. The next layer is the lamina fibrosa, which is composed of dense collagen mostly in a circumferential pattern. Because of the thickness and density of this layer, it is the strongest layer and important for bearing the stress of diastolic pressure. The middle layer, referred to as the spongiosa, forms the core of the leaflets at their bases. It is made of loose connective tissue consisting of water and glycosaminoglycans with sparse fibers and cellularity. The semifluid nature of this layer gives the leaflet considerable plasticity.
FIGURE 26-5
Schematic representation of a cross-section through the aortic valve leaflet showing the continuity of endocardial and endothelial components with the aortic valve. Inset illustrates the radial and circumferential axes of the valve leaflet. (Reproduced with permission from Cleveland Clinic, Cleveland, OH.)

The AV passively opens and closes in response to pressure differences between the left ventricle and aorta during the cardiac cycle. Pressure generated from ventricular contraction leads to valve opening, and the subsequent relatively higher pressure of the aorta leads to valve closure. The mechanical properties of the AV allow it to open with minimal transvalvular gradient and close completely with minimal flow reversal.
Pressure differences between the aorta and the ventricle coupled with compliance of the aortic root cause aortic root dilation and constriction during the cardiac cycle. This dynamic motion of the root plays an important role in opening and closing of the AV. During late diastole, as blood fills the ventricle, a 12% expansion of the aortic root occurs 20 to 40 ms before AV opening.4 The leaflets begin to open as a result of root dilation even before any generation of positive pressure from ventricular contraction. Root dilation alone opens the leaflets about 20%.5 As pressure rises in the LVOT, tension across the leaflets produced from root dilation lessens. As pressure continues to rise, the pressure difference across the leaflets is minimal, and no tension is present within the leaflet.6 This loss of tension allows the aortic root to further dilate and allows the valve to open rapidly at the beginning of ejection. Under normal circumstances, the AV presents little or no obstruction to flow because the specific gravity of the leaflets is equal to that of blood.7 These mechanisms permit rapid opening of the valve and minimal resistance to ejection.8
Closure of the AV is an elegant mechanism, which has interested investigators since the time of Leonardo da Vinci.9 A principal theory involved in closure is vortex theory, which recognizes the importance of the sinuses of Valsalva in valve closure.10 As ejection occurs, blood creates small eddy currents or vortices along the aortic wall. At the end of ejection and before valve closure, these vortices fill the sinuses and balloon the leaflets away from the aortic wall toward the aortic axis. After the pressure difference across the open AV equalizes, a small flow reversal forces the leaflets completely closed. Apposition of the valve leaflets occurs briskly and the ensuing second heart sound occurs after complete closure of the AV. Upon closing, the elastic leaflets stretch and recoil to generate compression and expansion of blood. The subsequent pressure changes produce the second heart sound; the sound is not produced by physical apposition of the valve leaflets.11
Aortic stenosis (AS) is incomplete opening of the AV, which restricts blood flow out of the left ventricle during systole.
In developed countries, AS is the most prevalent valvular heart disease in adults.12 Observational echocardiography studies demonstrate that 2% of people 65 years of age or older have isolated calcific AS, whereas 29% exhibit age-related AV sclerosis without stenosis.13 AS is more common in men and its prevalence increases with age.14 In patients aged 65 to 75 years, 75 to 85 years, and greater than 85 years, the prevalence of AS is 1.3, 2.4, and 4%, respectively.15 The most common causes of AS are acquired degenerative disease, bicuspid AV, and rheumatic heart disease.
The most common cause of AS is degenerative calcification of the AV, which typically occurs in septuagenarians and octogenarians. Progressive calcification, initially along the flexion lines at the leaflet bases, leads to immobilization of the cusps. The characteristic pathologic findings are discrete, focal lesions on the aortic side of the leaflets that can extend deep into the aortic annulus. The deposits may involve the sinuses of Valsalva and the ascending aorta. Although long considered to be the result of years of mechanical stress on an otherwise normal valve, it is now understood that the mechanical stress leads to proliferative and inflammatory changes, with lipid accumulation, upregulation of angiotensin-converting enzyme (ACE) activity, and infiltration of macrophages and T lymphocytes in a process similar to atherosclerosis.16-20 The risk factors for the development of calcific AS are similar to those for atherosclerosis and include elevated serum levels of low-density lipoprotein (LDL) cholesterol, diabetes, smoking, and hypertension.15 Therefore, coronary artery disease is commonly present in patients with AS. Age-related AV sclerosis is associated with an increased risk of cardiovascular death and myocardial infarction (MI).
Calcific AS is also observed in a number of other conditions, including Paget’s disease of bone and end-stage renal disease.21 Ochronosis with alkaptonuria is another rare cause of AS, which also can cause a rare greenish discoloration of the AV.22
A calcified bicuspid AV represents the most common form of congenital AS. Bicuspid AVs are present in approximately 2% of the general population. Gradual calcification of the bicuspid AV results in significant stenosis most often in the fifth and sixth decades of life, earlier in unicommissural than bicuspid valves and earlier in men than women.23,24 The abnormal architecture of the unicommissural or bicuspid AV induces turbulent flow, which injures the leaflets and leads to fibrosis, increased rigidity, leaflet calcification, and narrowing of the AV orifice.25 Several genes have been associated with bicuspid AV. Mutations in the signaling and transcriptional regulator NOTCH1 result in abnormal valve development and subsequent calcium deposition, providing a link between genetic mutations, valve morphology, and subsequent calcification associated with bicuspid AS.26-29 Structural abnormalities in microfibrils within the AV and aortic root lead to decreased structural integrity, resulting in dilation, aneurysms, and dissection.25,26,30
In Western countries, rheumatic AS represents the least common form of AS in adults.31,32 Rheumatic AS is rarely an isolated disease and usually occurs in conjunction with mitral valve stenosis.33,34 Rheumatic AS is characterized by diffuse fibrous leaflet thickening with fusion, to a variable extent, of one or more commissures. The progression of rheumatic AS is slower than that of degenerative calcific disease.24,35 The early stage of rheumatic AS is characterized by edema, lymphocytic infiltration, and revascularization of the leaflets, whereas the later stages are characterized by thickening, commissural fusion, and scarred leaflet edges.
In adults with calcific disease, the AV slowly thickens over time. Early, it causes little hemodynamic disturbance as the valve area is reduced from the normal 3 to 4 cm2 to 1.5 to 2 cm2.24,35 Past this point, hemodynamically significant obstruction of LV outflow develops with a concomitant increase in LV pressure and lengthening of LV ejection time. The elevated LV pressure increases wall stress, which is normalized by increased wall thickness and LV hypertrophy (LVH). As it hypertrophies, the left ventricle becomes less compliant and LV end-diastolic pressure (LVEDP) increases without chamber dilatation. This reflects diastolic dysfunction and the ventricle becomes increasingly dependent on atrial systole for filling.36 Hence, if a patient develops an atrial arrhythmia, he or she can rapidly decompensate.
Although adaptive, the concentric hypertrophy that develops has adverse consequences. LVH, increased systolic pressure, and prolonged ejection time all contribute to an increase in myocardial oxygen consumption. Increased diastolic pressure increases endocardial compression of the coronary arteries, reducing coronary flow reserve (or maximal coronary flow).37 Prolonged ejection also results in decreased time in diastole and therefore reduced myocardial perfusion time. The increased demand of the hypertrophied ventricle and decreased delivery capacity can yield subendocardial ischemia with activity. This can result in angina and LV dysfunction. LVH also makes the heart more susceptible to ischemic injury. Severe LVH is only partly reversed by AV replacement (AVR) and is associated with decreased long-term survival even after initially successful surgery.38
In late stages of severe AS, the left ventricle decompensates with resulting dilated cardiomyopathy and heart failure. Cardiac output (CO) declines and the pulmonary artery pressure rises, leading to pulmonary hypertension.
Myocardial hypertrophy in patients with AS is characterized by increased gene expression for collagen I and II, and fibronectin that is associated with activation of the renin-angiotensin system.39 Reduction in renin-angiotensin parallels regression of hypertrophy after AVR.40 Experimental studies have indicated a role of apoptotic mechanisms in the progression to LVH and heart failure in patients with AS.41,42 Patients who present with symptoms of congestive heart failure (CHF) have a 1-year survival of approximately 60%.43,44
The severity of AS can be assessed by measuring the AV orifice area (AVA), mean pressure gradient, and peak jet velocity. Effective AVA is calculated using the cross-sectional area of the LVOT (CSALVOT), flow velocity through the LVOT (VTILVOT), and AS jet velocity (VTIAV) using the continuity equation, which is based on the concept that stroke volume through the LVOT and AV are equal.45-49 The continuity equation is
Valve areas of less than 1.0 cm2 represent severe AS. Although the effective AVA is smaller than the anatomic AVA, it is a reliable predictor of clinical outcomes and is generally used in clinical decision making.47,50
The Gorlin formula, which describes the fundamental relationships linking the area of an orifice to the flow and pressure drop across the orifice, is used to calculate anatomic AVA using a Fick or thermodilution CO measurement taken during catheterization.49,51 The Gorlin formula uses the time from AV opening to closure (SEP) as
The Gorlin formula can also be used to calculate AVA from Doppler but is less favorable than the continuity equation as it underestimates the AVA in low-output states.52,53
In patients with AS, the transvalvular pressure gradient can be measured by simultaneous catheter pressure measurements in the left ventricle and proximal aorta. The peak-to-peak gradient, measured as the difference between peak LV pressure and peak aortic pressure, is used commonly to quantify the valve gradient.
Invasive measurements of AS severity have been largely replaced by echocardiographic measurements, which are currently the clinical standard.47,49 The gradient can be calculated from the Doppler acquired VTIAV using the Bernoulli equation as
Transesophageal echocardiography (TEE) is an alternative method for assessment of AVA that uses planimetry of the systolic short-axis view of the AV (Fig. 26-6).54 Planimetry of the valve area is challenging because the valve orifice is a complex, three-dimensional shape, and area measurements assume the valve orifice lies entirely within the image plane. Thus, it is only recommended when Doppler estimation of flow velocities is unreliable.47
FIGURE 26-6
Transesophageal echocardiographic image of aortic stenosis resulting from severe degenerative calcification. Transverse section of the aortic root at the level of the aortic valve orifice showing the aortic ring (arrow), the sinuses of Valsalva (asterisks), and a significantly reduced aortic valve orifice area of 0.44 cm2 (dotted line).

The cardinal symptoms of AS are angina pectoris, syncope, and symptoms of CHF (dyspnea, orthopnea, and paroxysmal nocturnal dyspnea).55 Although the mechanisms of angina and heart failure are well understood, the mechanism of syncope is less clear. A common theory is that the augmented stroke volume that usually accompanies exercise is limited by the narrowed outflow orifice. With exercise-induced reduction in peripheral arterial resistance, blood pressure drops, leading to cerebral hypoperfusion and syncope.56 Syncope also may be the result of dysfunction of baroreceptor mechanisms and a vasodepressor response to the increased LV systolic pressure during exercise. Besides these cardinal symptoms, patients also commonly present with more subtle symptoms, such as fatigue, decreased exercise tolerance, and dyspnea on exertion.57
A rare presentation of AS is gastrointestinal bleeding secondary to angiodysplasia occurring predominantly in the right colon, as well as in the small bowel or stomach. This complication arises from shear-stress-induced platelet aggregation with reduction in high-molecular-weight multimers of von Willebrand factor and increases in proteolytic subunit fragments. These abnormalities correlate with the severity of AS and are correctable by AVR.58 Other late manifestations of severe AS include atrial fibrillation and pulmonary hypertension. Infective endocarditis can occur in younger patients with AS; it is less common in elderly patients with a severely calcified valve.
Patients who develop severe AS have a long period of asymptomatic progression in which morbidity and mortality is relatively low (Fig. 26-7).55 Sudden death from AS before the onset of symptoms is estimated to be approximately 1% per year.59 With the onset of symptoms, the mortality rate is 25% per year without surgical intervention.12 Of the 35% of patients who present with angina, 50% survive for 5 years. Of the 15% who present with syncope, 50% survive for 3 years, and mean survival for those who present with CHF is 2 years.55
AS is frequently first diagnosed before symptom onset by auscultation of a murmur on physical examination. Classically, AS causes a systolic crescendo-decrescendo murmur, heard loudest at the right upper sternal border. Another sign of AS is a delayed second heart sound (S2) because of prolongation of the systolic ejection time. S2 also may be single when the aortic component is absent, and if the aortic component is audible, this may give rise to a paradoxical splitting of S2.
The classic pulsus parvus (small pulse) is a sign of severe AS or decompensated AS and occurs when stroke volume and systolic and pulse pressures fall. A wide pulse pressure is also characteristic of AS. Prolongation of the ejection phase with slow rise in the arterial pressure also gives rise to the pulsus tardus (late pulse). Pulsus parvus et tardus is diagnosed by palpation.
LVH is evident as a sustained apical thrust or heave. This sign is present only when failure occurs because before failure occurs, the hypertrophy is not accompanied by dilatation, and the apical impulse is not displaced. Conversely, absence of an apical thrust (except in muscular patients, or those with emphysema or adiposity) suggests mild or moderate AS. Other physical findings of significant AS include a prominent atrial kick and prominence of the jugular venous a wave secondary to decreased right ventricular compliance caused by right ventricular hypertrophy.60
Most patients with severe AS present with QRS complex or ST-T interval abnormalities reflecting LVH. Patients with a higher gradient are more likely to show a “strain” or “systolic overload” pattern. The conduction abnormalities may result from septal trauma secondary to high intramyocardial tension from hypoxic damage to the conducting fibers or from extension of valvular calcifications into the fibrous septum.
The roentgenographic characteristics of compensated AS include concentric hypertrophy of the left ventricle without cardiomegaly, poststenotic dilatation of the aorta, and calcification of the valve cusps. With decompensation, there is cardiomegaly in the posteroanterior projection and pulmonary venous congestion. It is important to recognize that a routine chest x-ray may be within normal limits in patients with hemodynamically compensated AS. The rounding of the lower-left heart border may be subtle, the poststenotic aortic dilatation may be equivocal, and the valvular calcification may be invisible on the posteroanterior view. Of equal importance, the presence of cardiomegaly in a normotensive patient with isolated AS indicates decompensated AS.
Echocardiography is the diagnostic tool of choice for confirming the diagnosis of AS and quantification of disease severity. Echocardiography is used to define: (1) the severity and etiology of AS; (2) coexisting valvular abnormalities; and (3) cardiac chamber size and function.
The development of diastolic dysfunction in patients with AS can lead to symptom development, and may increase late mortality after AVR.38,61 Hence, the quantification of diastolic dysfunction is important in the assessment of AS. LV filling pressures can be assessed by calculating the ratio of transmitral flow velocity and annular velocity obtained at the level of the mitral annulus with tissue Doppler.62-64 In patients with normal LV function (LVF), stress echocardiography is used to determine if symptom development during exercise is due to diastolic dysfunction.65 Diastolic dysfunction in patients with normal LVF may cause exercise intolerance for several reasons: (1) elevated LV diastolic and pulmonary venous pressures increase the work of breathing and cause dyspnea; (2) patients with LVH exhibit a limited ability to use the Frank-Starling mechanism during exercise, resulting in a decrease in CO during exercise; and (3) elevated LV diastolic and pulmonary venous pressures result in abnormalities in the diastolic properties of the ventricle.
When AS is suspected, an initial Doppler echocardiogram can confirm the diagnosis and assess the severity. Re-examination should be performed upon change in signs or symptoms, or periodically, every 3 to 5 years for mild AS, every 1 to 2 years for moderate AS, and every 6 months to 1 year for severe AS, to identify worsening stenosis, LV dysfunction, LVH, and mitral regurgitation. Although AS is best understood as a disease continuum, severity can be graded by echocardiographic evaluation of hemodynamics. The current guidelines use definitions based on the AVA, mean pressure gradient, and peak jet velocity (Table 26-1).49
Exercise testing should be avoided in symptomatic patients with AS. Traditionally, severe AS has been regarded as a relative contraindication to exercise testing. Recent studies have indicated that quantitative exercise Doppler echocardiography can be performed safely in asymptomatic patients, including those with severe AS, and may be useful for identifying patients who at higher risk of becoming symptomatic and/or requiring AVR. Asymptomatic patients with severe AS who become symptomatic on exercise stress testing have a higher rate of cardiac death or progression to AVR.49,66,67 Thus, patients with provoked symptoms should be considered symptomatic. Dobutamine stress echocardiography is occasionally used in severe AS, specifically to assess contractile reserve in patients with moderate to severe AS with a low AV gradient and depressed LVF.68,69
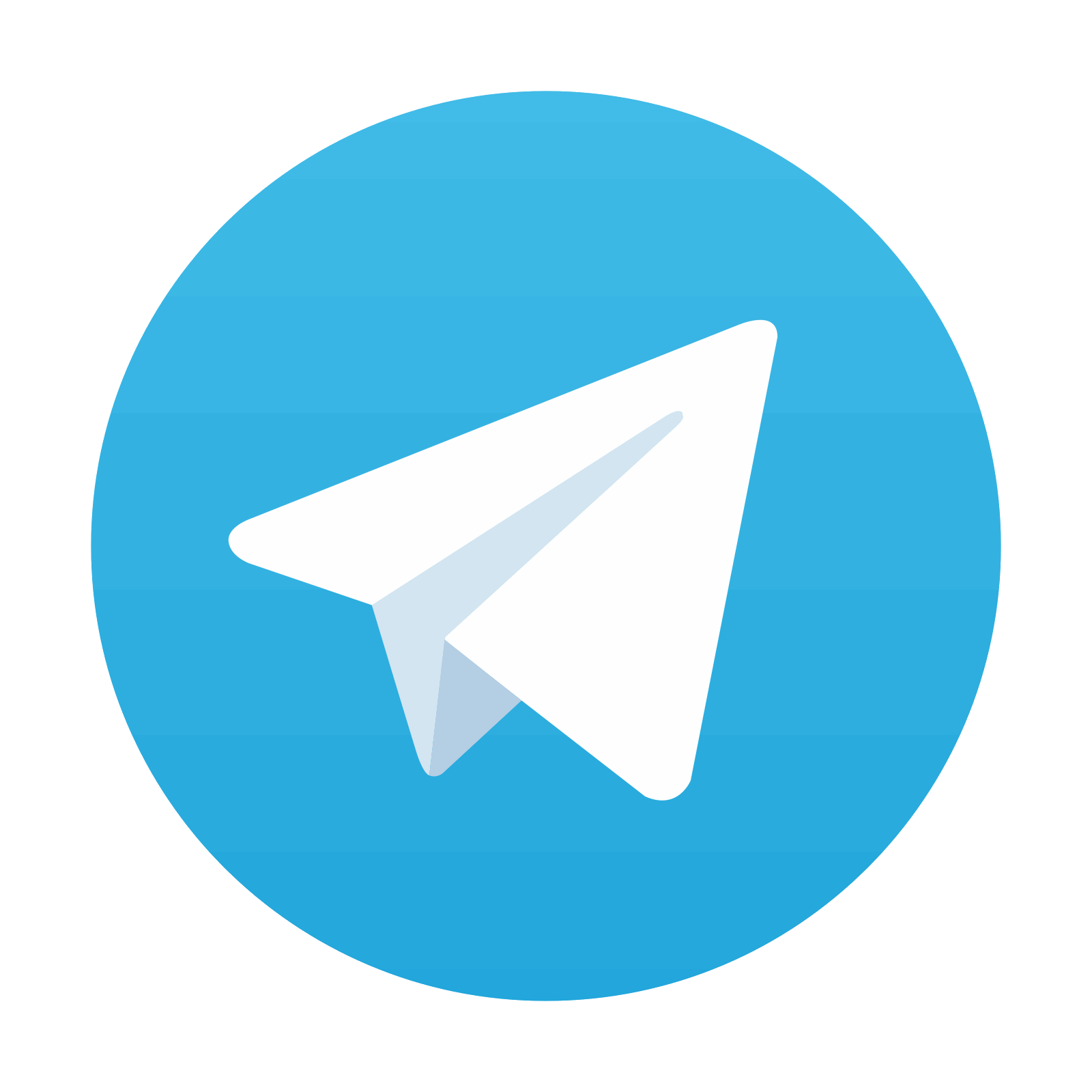
Stay updated, free articles. Join our Telegram channel

Full access? Get Clinical Tree
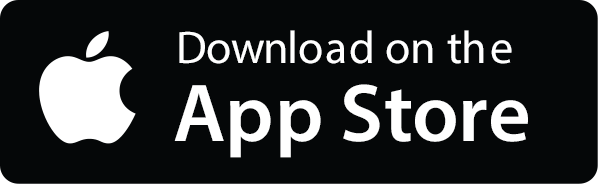
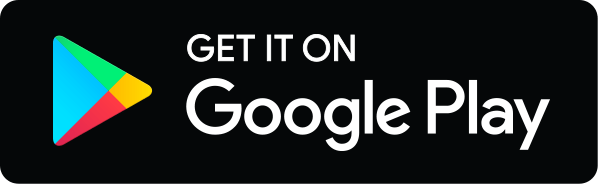