Fig. 1
Role of Immune system activation, brain and bone marrow in hypertension. Immune system activation via T cells, macrophages and various cytokines results in central and peripheral inflammation in the vasculature and kidney contributing to hypertension. Neuro-inflammation from hypertensive stimuli promotes sympathetic nervous system (SNS) activity causing hypertension. SNS activity in bone marrow increases recruitment and release of hematopoietic stem cell progenitors (HSPC) and cytokines, furthering the pro-inflammatory state
5 Vasculo-Protective RAAS Pathways
RAAS blockade generates alternate metabolites of Ang that can exert an anti-hypertensive effect. Vasculo-protective RAS pathways include Angiotensinase A-Ang III-Ang II type 2 (AT2) receptor pathway and ACE 2-Ang-(1–7)-Mas receptor pathway (Te Riet et al. 2015).
5.1 AT2 Receptor Pathway
Upregulation of AT2 receptors counteracts the vaso-deleterious effects of angiotensin (AT1) type 1 receptor. Mice deficient in AT2 receptor exhibit increased blood pressure and baroreflex sensitivity, and decreased pressure-natriuresis and AT1 receptor expression (Gross et al. 2004; Tanaka et al. 1999; Gembardt et al. 2008). Overexpression of AT2 receptor reverses these effects (Tsutsumi et al. 1999). Four AT2 agonist molecules are currently being developed for clinical use in hypertension namely: peptidergic agonists β-Tyr4-Ang II, β-Ile5-Ang II and LP2-3 and the non-peptide agonist Compound 21 (C21). AT2 receptor mediated aortic vasodilation was reported in mice treated with peptidergic agonists (Jones et al. 2011; Wagenaar et al. 2013). Interestingly, vasodilatory effects of AT2 receptor are appreciated most on a background of partial AT1 receptor blockade (Esch et al. 2009; Sevá Pessôa et al. 2012). Indeed, simultaneous administration of β-Ile5-Ang II and candesartan lowered BP in spontaneously hypertensive rat (Jones et al. 2011).
5.2 ACE2-Ang-(1–7)-Mas Receptor Pathway
Angiotensin converting enzyme 2 (ACE 2) acts on angiotensin II to produce angiotensin (Ang)-(1–7) (Santos et al. 2003). Interaction of Ang-(1–7) and Mas receptor oppose AT1 receptor functions. Further, Ang-(1–7) bind to AT2 receptors and even AT1 receptors at high doses. Several cardiovascular benefits have been attributed to Ang-(1–7), however its role in hypertension at present is unclear (Te Riet et al. 2015). Ang (1–7) has weak vasodilatory effects and has not uniformly exhibited anti-hypertensive effects in animal models (Sevá Pessôa et al. 2012; Durik et al. 2012).
Nevertheless, therapeutic options utilizing the vasoprotective effects of Ang-(1–7) have been explored. Cyclodextrin-encapsulated Ang-(1–7), non-peptide drug AVE0091 and peptide drug CGEN856S have shown BP-lowering effects in hypertensive animals (Sevá Pessôa et al. 2012; Ferreira et al. 2010).
Anti-hypertensive effects of ACE 2 have also been questioned. Overexpression of ACE 2 does lower blood pressure, however, its ability of degrade Ang-II could also account for anti-hypertensive effect (Gurley et al. 2006). Hypotension due to ACE 2 agonists Diminazine and XNT was recently shown to be independent of ACE2-Ang-(1–7)-Mas pathway further weakening the evidence in support of its role in the pathogenesis of hypertension (Haber et al. 2014).
5.3 Alamandine
Alamandine, a recently discovered hormone of RAS, shares similar biological structure and activity to that of Ang-(1–7) including promotion of vasodilation and anti-hypertensive effects. ACE 2 hydrolyzation of Ang A and decarboxylation of Ang-(1–7) have both been shown to produce this endogenous peptide within the body (Villela et al. 2014). Lautner et al. demonstrated that alamandine interacts with its own specific MrgD receptor (Mas-related G-protein coupled receptor, member D) (Lautner et al. 2013). Oral administration of alamandine as a HP-B Cyclodextrin inclusion compound produced long term anti-hypertensive effect in spontaneously hypertensive rats (Lautner et al. 2013). Moreover, microinjections of almandine into the medullary brain demonstrated cardiovascular effects similar to that of Ang-(1–7), indicating a possible central role of BP control by alamandine (Villela et al. 2014; Mendoza-Torres et al. 2015). Further studies are required to delineate the role Alamandine plays in the RAAS, and as a anti-hypertensive agent.
5.4 Angiotensin III Inhibitors
Angiotensin III is a recognized AT2 receptor agonist in the kidney and vasculature (Kemp et al. 2012). However, in the brain, it is believed to be the preferred AT1 receptor agonist and therefore can cause hypertension (Wright et al. 2003). Aminopeptidase A inhibitors that act preferentially in the CNS to block Ang II conversion to Ang III are being developed. An orally administered prodrug (RB150), that converts to an aminopeptidase A inhibitor EC33 in the brain, exhibited antihypertensive effects in animal models. RB 150 is currently being evaluated in a phase Ib clinical study (Gao et al. 2014).
6 Aldosterone Mineralocorticoid Receptor Pathway
Aldosterone hormone activation of mineralocorticoid receptors (MR), a member of the steroid receptor family, is known to promote vascular cell oxidative stress, inflammation, proliferation, migration and extracellular matrix production (McCurley and Jaffe 2012; Lother and Hein 2016). Vaso-deleterious effects caused by MR activation include: vasoconstriction, atherosclerosis, and vascular remodeling and fibrosis (Lother and Hein 2016; Udelson et al. 2010). Nishiyama et al. demonstrated in animal models that mineralocorticoid-induced hypertension is associated with increased vascular oxidative stress and ROS production (McCurley and Jaffe 2012; Nishiyama and Abe 2004; Iglarz et al. 2004). Subsequently, MR antagonism showed a decrease in NADPH-oxidase activity, resulting in less ROS production, and thus lower BP (Keidar et al. 2003; Sanz-Rosa et al. 2005).
Activation of MR in the vascular bed is also associated with increased expression of proinflammatory factors including ICAM1, monocyte chemoattractant protein (MCP-1), cytokines, placental growth factor (PGF), COX-2 and transcription factor NF-κB (McCurley and Jaffe 2012; Rocha et al. 2002). Aortas from patients with atherosclerosis demonstrated a decrease in PGF and connective tissue growth factor when treated with spironolactone (a MR antagonist) (Jaffe et al. 2010; Newfell et al. 2011). Furthermore, the controlled Prevention and Treatment of Hypertension With Algorithm-Based Therapy (PATHWAY)-2 clinical trial showed a decrease in blood pressure by 8.7 mmHg with spironolactone treatment in patients with resistant hypertension (Lother and Hein 2016; MacDonald et al. 2015). Such findings support the role of MR-activation induced inflammation as a contributing factor to hypertension. However, further trials exploring the use of MR antagonists as anti-hypertensive agents are required to confirm these results.
7 VEGF Inhibitors
Anti-angiogenesis therapy targeting vascular endothelial growth factor (VEGF) and its receptors as treatment for anti-tumor growth has long been associated with adverse side effects of hypertension and renal toxicity manifesting as proteinuria and renal function impairment (Lankhorst et al. 2016; van den Meiracker and Danser 2016). Physiologically, VEGF mediates vasodilation through increased nitric oxide (NO) production by upregulating the NOS gene expression and increased endothelial NOS phosphorylation (Hood et al. 1998; Shen et al. 1999). The subsequent decrease in NO availability following VEGF inhibition contributes to the pathogenesis of hypertension during anti-angiogenesis therapy (van den Meiracker and Danser 2016; Facemire et al. 2009). Recent investigations of the role of the Endothelin (ET) system, specifically Endothelin-1, suggest this system as a more significant contributor to the rise in BP compared to NO deprivation. A rise in ET-1 is noted with administration of sunitinib, a receptor tyrosine kinase (RTK) inhibitor that targets VEGF receptors (Kappers et al. 2010). Endothelin-1 (ET-1) interacts with G-protein-coupled membrane bound ETA and ETB receptors on vascular smooth muscle to mediate vasoconstriction. Furthermore, ETA receptor antagonism prevented anti-angiogenic induced hypertension in mice (Li et al. 2012). Currently, there is no established guideline for reversing hypertension induced by VEGF-targeted therapies (Hayman et al. 2012).
8 Asymmetric Dimethylarginine
Asymmetric dimethyarginine (ADMA) is a naturally occurring amino acid synthesized when arginine residues in proteins undergo methylation by arginine methyltransferases (PRMTs) (Vallance and Leiper 2004; Vallance et al. 1992). An additional by-product of this interaction is NG-monomethyl- L-arginine (L-NMMA), a methylarginine similar to ADMA in its biological activity. Following hydrolysis of methylated proteins ADMA and L-NMMA are released in the cytosol. ADMA is found in various tissues, circulates in plasma and is excreted in urine (Vallance and Leiper 2004). In addition to renal clearance, ADMA is extensively metabolized by dimethylarginine dimethyl aminohydrolases (DDAHs) resulting in conversion to citrulline (Achan et al. 2003).
Both ADMA and L-NMMA competitively inhibit all three isoforms NOS, thereby causing vasoconstriction, impaired endothelium-mediated vasodilatation, increased endothelial adhesiveness, and hypertension (Vallance et al. 1992; Achan et al. 2003; Hasegawa et al. 2007; Kielstein et al. 2004; Barba et al. 2000; Böger et al. 2000). Targeted deletion of DDAH gene and use of specific inhibitors of DDAH lead to accumulation of ADMA, a reduction in NO signaling and subsequent vascular dysfunction, increased systemic vascular resistance and blood pressure (Leiper et al. 2007). Transgenic mice overexpressing DDAH demonstrated reduced ADMA levels and increased cardiac NO levels. However, transgenic mice showed no changes in systemic BP compared to wild type controls under normal conditions (Hasegawa et al. 2007). Two-week treatment with angiotensin II increased ADMA levels, cardiac oxidative stress and vascular injury while DDAH overexpression attenuated these changes (Hasegawa et al. 2007). Conversely, Jacobi et al. reported no increase in ADMA levels following 4-week Ang II infusion (Jacobi et al. 2008). Overexpression of DDAH however attenuated ang-II medicated end organ damage (Jacobi et al. 2008).
Small clinical studies have noted an association between hypertension and increased ADMA levels (Surdacki et al. 1999; Perticone et al. 2005). Larger scale clinical studies have however not corroborated these findings (Meinitzer et al. 2007; Schnabel et al. 2005). Trials evaluating the effect of antihypertensive agents on ADMA levels have yielded conflicting results. Some investigators reported significant reductions in ADMA levels following treatment with RAS, (Chen et al. 2002; Delles et al. 2002; Ito et al. 2002; Napoli et al. 2004; Aslam et al. 2006) while others have failed to confirm these findings (Fliser et al. 2005; Warnholtz et al. 2007). Differences in study design, treatment regimens, methods of determining ADMA levels may account for the discrepant findings of the aforementioned studies.
ADMA has been shown to have a negative correlation with endothelial-dependent vasodilation (EDV) and a positive correlation with carotid artery intima-media thickness (IMT) in hypertensive patients with administration of salbutamol and nitroglycerine (Serg et al. 2011). Cakar and colleagues evaluated arterial stiffness markers for endothelial dysfunction and found that ADMA levels significantly correlated with augmentation index (Aix) and CRP levels. However, there was no correlation between PWV and central aortic pressure (CAP) and ADMA (Cakar et al. 2015). Similarly, the PREVENCION study showed that ADMA and NMMA did not predict carotid-femoral PWV, blood pressure or hemodynamic abnormalities (Chirinos et al. 2008). Therefore, while it is very clear that ADMA plays a role in endothelial dysfunction and can cause significant changes in vascular flow, it still remains to be seen whether ADMA directly play a role in hypertension.
Therapeutic approaches specifically targeting ADMA pathways have been studied. Increased L-citrulline supplementation overcame ADMA inhibition, resulting in improved NO production in porcine hearts (Xuan et al. 2015). The effects of L-arginine on endothelial function, blood pressure, and ADMA levels in patients with hypertension (NCT02392767) and in patients with preeclampsia are currently being evaluated (NCT00275158).
9 Conclusion
The recently concluded SPRINT trial has suggested that achieving aggressive blood pressure targets reduces cardiovascular morbidity and mortality. This will require more aggressive and complex treatment regimens, in most cases with multi-drug protocols. In order to achieve better blood pressure control, it is imperative to understand the mechanisms that lead to increased blood pressure. Knowledge of the causative mechanisms will allow informed selection of agents and combinations that are additive and even synergistic. Such an approach will in turn allow the fashioning “personalized” treatment regimens that will maximally benefit each patient individually.
References
Achan V, Broadhead M, Malaki M, Whitley G, Leiper J, MacAllister R et al (2003) Asymmetric dimethylarginine causes hypertension and cardiac dysfunction in humans and is actively metabolized by dimethylarginine dimethylaminohydrolase. Arterioscler Thromb Vasc Biol 23(8):1455–1459PubMed
Agarwal D, Dange RB, Raizada MK, Francis J (2013) Angiotensin II causes imbalance between pro- and anti-inflammatory cytokines by modulating GSK-3β in neuronal culture. Br J Pharmacol 169(4):860–874PubMedPubMedCentral
Amadesi S, Reni C, Katare R, Meloni M, Oikawa A, Beltrami AP et al (2012) Role for substance p-based nociceptive signaling in progenitor cell activation and angiogenesis during ischemia in mice and in human subjects. Circulation 125(14):1774, –86–S1–19PubMedPubMedCentral
Amador CA, Barrientos V, Peña J, Herrada AA, González M, Valdés S et al (2014) Spironolactone decreases DOCA-salt-induced organ damage by blocking the activation of T helper 17 and the downregulation of regulatory T lymphocytes. Hypertension 63(4):797–803PubMed
Aslam S, Santha T, Leone A, Wilcox C (2006) Effects of amlodipine and valsartan on oxidative stress and plasma methylarginines in end-stage renal disease patients on hemodialysis. Kidney Int 70(12):2109–2115PubMed
Barba G, Vallance PJ, Strazzullo P, MacAllister RJ (2000) Effects of sodium intake on the pressor and renal responses to nitric oxide synthesis inhibition in normotensive individuals with different sodium sensitivity. J Hypertens 18(5):615–621PubMed
Barhoumi T, Kasal DA, Li MW, Shbat L, Laurant P, Neves MF et al (2011) T regulatory lymphocytes prevent angiotensin II-induced hypertension and vascular injury. Hypertension 57(3):469–476PubMed
Bendich A, Belisle EH, Strausser HR (1981) Immune system modulation and its effect on the blood pressure of the spontaneously hypertensive male and female rat. Biochem Biophys Res Commun 99(2):600–607PubMed
Böger RH, Bode-Böger SM, Tsao PS, Lin PS, Chan JR, Cooke JP (2000) An endogenous inhibitor of nitric oxide synthase regulates endothelial adhesiveness for monocytes. J Am Coll Cardiol 36(7):2287–2295PubMed
Bomfim GF, Dos Santos RA, Oliveira MA, Giachini FR, Akamine EH, Tostes RC et al (2012) Toll-like receptor 4 contributes to blood pressure regulation and vascular contraction in spontaneously hypertensive rats. Clin Sci 122(11):535–543PubMedPubMedCentral
Bravo Y, Quiroz Y, Ferrebuz A, Vaziri ND, Rodríguez-Iturbe B (2007) Mycophenolate mofetil administration reduces renal inflammation, oxidative stress, and arterial pressure in rats with lead-induced hypertension. Am J Physiol Renal Physiol 293(2):F616–F623PubMed
Cakar M, Bulucu F, Karaman M, Ay SA, Kurt Ö, Balta Ş et al (2015) Asymmetric dimethylarginine and augmentation index in newly diagnosed patients with hypertension. Angiology 66(1):43–48PubMed
Calhoun DA, Bakir SE, Oparil S, DiMarco J (2000) Etiology and pathogenesis of essential hypertension. In: Cardiology. Mosby International, London
Cardinale JP, Sriramula S, Mariappan N, Agarwal D, Francis J (2012) Angiotensin II-induced hypertension is modulated by nuclear factor-κBin the paraventricular nucleus. Hypertension 59(1):113–121PubMed
Chen J-W, Hsu N-W, Wu T-C, Lin S-J, Chang M-S (2002) Long-term angiotensin-converting enzyme inhibition reduces plasma asymmetric dimethylarginine and improves endothelial nitric oxide bioavailability and coronary microvascular function in patients with syndrome X. Am J Cardiol 90(9):974–982PubMed
Chirinos JA, David R, Bralley JA, Zea-Díaz H, Muñoz-Atahualpa E, Corrales-Medina F et al (2008) Endogenous nitric oxide synthase inhibitors, arterial hemodynamics, and subclinical vascular disease: the PREVENCION Study. Hypertension 52(6):1051–1059PubMed
Crowley SD, Song Y-S, Lin EE, Griffiths R, Kim H-S, Ruiz P (2010) Lymphocyte responses exacerbate angiotensin II-dependent hypertension. Am J Physiol Regul Integr Comp Physiol 298(4):R1089–R1097PubMedPubMedCentral
Davies PF (2008) Hemodynamic shear stress and the endothelium in cardiovascular pathophysiology. Nat Clin Pract Cardiovasc Med 6(1):16–26PubMedPubMedCentral
De Batista PR, Palacios R, Martín A, Hernanz R, Médici CT, Silva MASC, et al (2014) Toll-like receptor 4 upregulation by angiotensin II contributes to hypertension and vascular dysfunction through reactive oxygen species production. Huang Y, editor. PLoS ONE. 9(8):e104020
De Ciuceis C, Amiri F, Brassard P, Endemann DH, Touyz RM, Schiffrin EL (2005) Reduced vascular remodeling, endothelial dysfunction, and oxidative stress in resistance arteries of angiotensin II-infused macrophage colony-stimulating factor-deficient mice: evidence for a role in inflammation in angiotensin-induced vascular injury. Arterioscler Thromb Vasc Biol 25(10):2106–2113PubMed
de Kloet AD, Liu M, Rodríguez V, Krause EG, Sumners C (2015) Role of neurons and glia in the CNS actions of the renin-angiotensin system in cardiovascular control. Am J Physiol Regul Integr Comp Physiol 309(5):R444–R458PubMedPubMedCentral
Delles C, Schneider MP, John S, Gekle M, Schmieder RE (2002) Angiotensin converting enzyme inhibition and angiotensin II AT1-receptor blockade reduce the levels of asymmetrical N(G), N(G)-dimethylarginine in human essential hypertension. Am J Hypertens 15(7 Pt 1):590–593PubMed
Durik M, Sevá Pessôa B, Roks AJM (2012) The renin-angiotensin system, bone marrow and progenitor cells. Clin Sci 123(4):205–223PubMed
Dzielak DJ (1991) Immune mechanisms in experimental and essential hypertension. Am J Physiol 260(3 Pt 2):R459–R467PubMed
Esch JHM, Schuijt MP, Sayed J, Choudhry Y, Walther T, Jan Danser AH (2009) AT2 receptor-mediated vasodilation in the mouse heart depends on AT1A receptor activation. Br J Pharmacol 148(4):452–458
Facemire CS, Nixon AB, Griffiths R, Hurwitz H, Coffman TM (2009) Vascular endothelial growth factor receptor 2 controls blood pressure by regulating nitric oxide synthase expression. Hypertension 54(3):652–658PubMedPubMedCentral
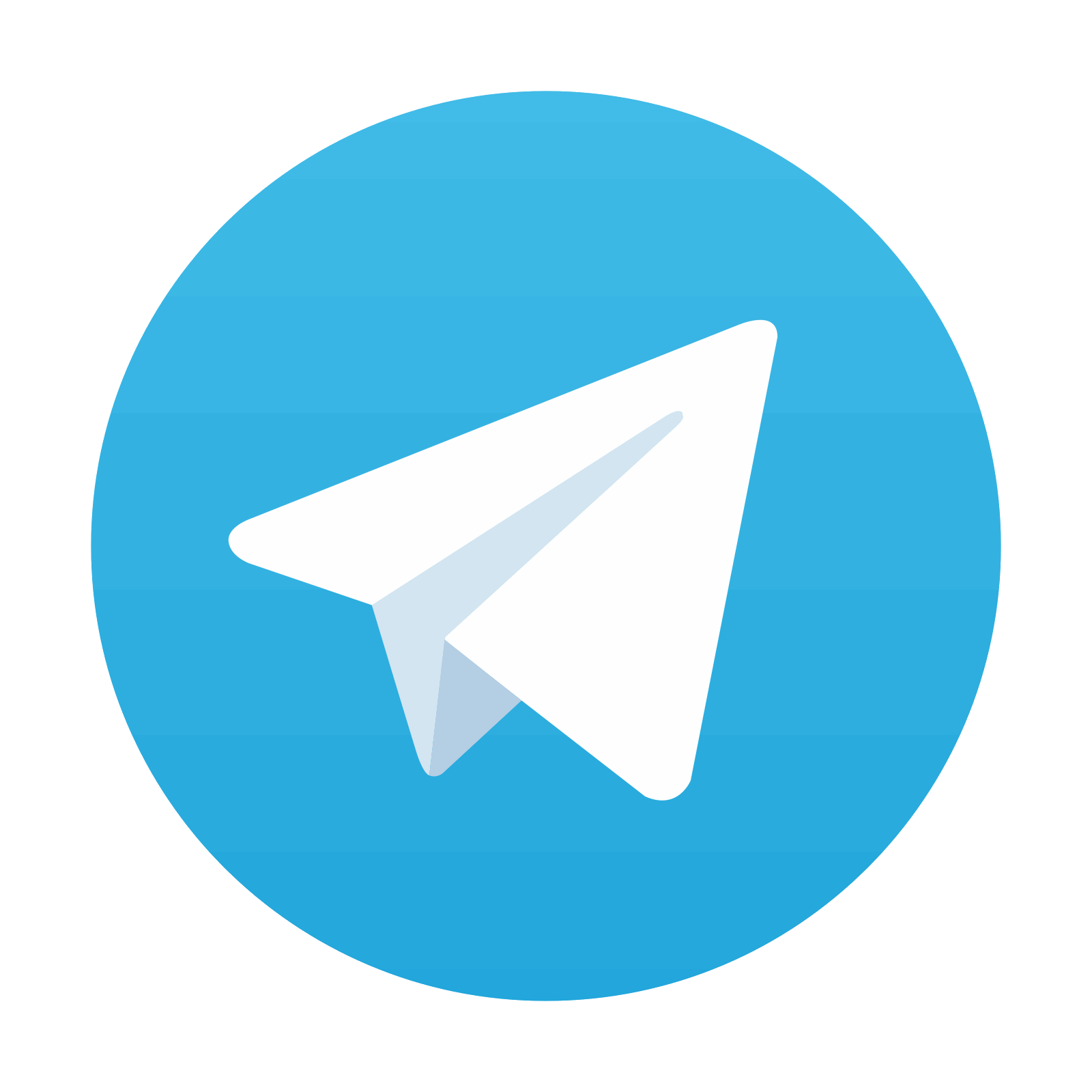
Stay updated, free articles. Join our Telegram channel

Full access? Get Clinical Tree
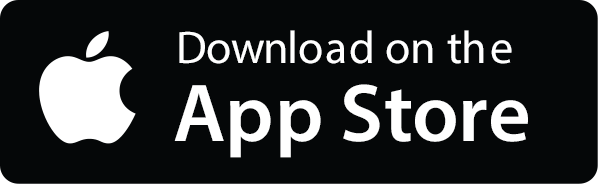
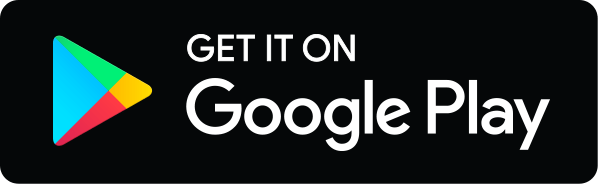