Since the initial description of the phenomenon by Jennings et al 50 years ago, our understanding of the underlying mechanisms of reperfusion injury has grown significantly. Its pathogenesis reflects the confluence of multiple pathways, including ion channels, reactive oxygen species, inflammation, and endothelial dysfunction. The purposes of this review are to examine the current state of understanding of ischemia-reperfusion injury, as well as to highlight recent interventions aimed at this heretofore elusive target. In conclusion, despite its complexity our ongoing efforts to mitigate this form of injury should not be deterred, because nearly 2 million patients annually undergo either spontaneous (in the form of acute myocardial infarction) or iatrogenic (in the context of cardioplegic arrest) ischemia-reperfusion.
Each year in the United States, there are approximately 1 million myocardial infarctions (MI), and 700,000 patients undergo cardioplegic arrest for various cardiac surgeries. Minimizing ischemic time in these clinical scenarios has appropriately received a great deal of attention because of the long-established relation between the duration of ischemia and the extent of myocardial injury. Once coronary flow is restored, however, the myocardium is susceptible to another form of insult stemming from reperfusion of the previously ischemic tissue. Given that cardiac ischemia is either unpredictable (MI) or inevitable (in the operating room), there is great interest in developing strategies to minimize reperfusion-mediated injury.
Historical Perspective
The seminal observation that reperfusion after ischemia was associated with myocardial injury was made in 1960 by Jennings et al. Their report was based on experiments with canine hearts subjected to coronary ligation in which reperfusion appeared to accelerate the development of necrosis. For example, those investigators noted that the histologic changes seen after only 30 to 60 minutes of ischemia-reperfusion (IR) were comparable to the degree of necrosis normally seen after 24 hours of permanent coronary occlusion.
Whether reperfusion is independently responsible for tissue injury or simply hastens the demise of cells otherwise destined for necrosis remained a matter of debate for some years. Evidence for direct myocardial reperfusion–dependent injury was summarized in 1985 in the classic editorial by Braunwald and Kloner. However, it was not until the discovery of ischemic preconditioning that the independent effects of ischemia and reperfusion began to be unraveled from each other.
In 1986, Murry et al described a process whereby repetitive short bouts of ischemia preceding prolonged periods of ischemia with reperfusion resulted in significantly decreased infarct sizes in dogs. Subsequently, this “ischemic preconditioning” was confirmed in a number of animal models, including humans, highlighting it as an evolutionarily conserved mechanism. Subsequent experiments revealed that the reperfusion event is key to the initiation of a molecular cascade leading to cardioprotection, thereby serving to solidify the important distinction between ischemia and subsequent reperfusion.
Mechanisms of Ischemia-Reperfusion Injury
Molecular and cellular events underlying IR injury are complex, representing the confluence of divergent biologic pathways. Furthermore, the extent to which each of these pathways is relevant to human disease remains unclear, as animal models do not always faithfully recapitulate the IR disease process in humans. These limitations notwithstanding, several key pathophysiologic features of clinically relevant IR have emerged ( Table 1 ).
• Ion accumulation |
∘ Intracellular calcium overload |
∘ Increased intracellular sodium |
∘ Decrease in pH with rapid normalization upon reperfusion |
• Dissipation of mitochondrial membrane potential |
∘ mPTP |
• Free radical formation/ROS |
∘ Generation from xanthine oxidase |
∘ Release of reactive mitochondrial intermediates |
∘ Neutrophil infiltration |
∘ ROS-induced ROS |
• NO metabolism |
∘ Loss of NO-vasodilation |
∘ Accumulation of reactive peroxynitrite |
• Apoptosis and autophagy |
• Endothelial dysfunction |
∘ Cytokine and chemokine signaling |
∘ Expression of cellular adhesion markers |
∘ Impaired vasodilation |
• Platelet aggregation and microembolization |
• Immune activation |
∘ Innate immunity (e.g., complement activation, expression of Toll-like receptors) |
∘ Neutrophil accumulation |
∘ Cell-mediated damage (macrophage and T cell) |
Ischemia induces the accumulation of intracellular sodium, hydrogen, and calcium ions, culminating in tissue acidosis. Reperfusion, in turn, elicits rapid alterations in ion flux, and some evidence suggests that rapid renormalization of pH paradoxically leads to enhanced cytotoxicity. Sodium-dependent pH regulatory mechanisms, including the Na + -H + exchanger and the Na + -HCO 3 − transporter, are activated, which consequently leads to intracellular sodium accumulation. High sodium concentrations, in turn, drive increases in sarcoplasmic reticular Ca 2+ by the Na + -Ca 2+ exchange. Enhanced Ca 2+ entry by sarcolemmal L-type Ca 2+ channels and a deficient import of cytosolic Ca 2+ into the sarcoplasmic reticulum by sarcoplasmic/endoplasmic reticulum Ca 2+ –adenosine triphosphatase further promote Ca 2+ overload. The result is myofibrillar hypercontractility, adenosine triphosphate depletion, ultrastructural damage to mitochondria, and myocardial stunning.
Cardiac myocytes consume large quantities of energy. To accommodate this requirement, these cells host a high density of mitochondria. Thus, it is not surprising that these complex, energy-generating organelles, filled with reactive intermediates and proapoptotic signals, are intimately involved in IR injury. As part of this, the mitochondrial permeability transition pore (mPTP) has been the center of a growing amount of attention. The inner mitochondrial membrane, responsible for maintaining mitochondrial transmembrane potential, is normally impermeable to ions and proteins. Dissipation of the electrical potential across this membrane is termed “permeability transition,” a process thought to be mediated through the mPTP. Although the constituent protein components of the pore remain unknown, formation of the pore creates a nonselective channel between the inner membrane of the mitochondrion and the sarcoplasm. This results in loss of the electrochemical gradient, the release of reactive oxygen species (ROS), and apoptosome formation. Triggers for mPTP include Ca 2+ overload, rapid normalization of pH, and oxidative stress.
The generation of free radicals through incomplete reduction of oxygen during IR has been well described. These oxygen species are highly reactive and can quickly overwhelm a cell’s endogenous free radical scavenging system. This in turn triggers cellular injury by reactions with lipids, proteins, and nucleic acids. The enzyme xanthine oxidase has been particularly implicated as a generator of free radicals in the reperfused heart, as its substrates (xanthine and hypoxanthine) accumulate during ischemia. In addition to damaging nuclear and cytosolic elements, ROS can trigger the opening of the mPTP. This results in a positive feedback loop of additional free radical release from the mitochondria (“ROS-induced ROS release”).
Not only is IR injury dependent on events occurring within cardiomyocytes, but the endothelium is an active participant as well. The endothelium is the major source of the evanescent molecule, nitric oxide (NO). Under normal conditions, NO generation elicits vasodilation, which has beneficial, protective effects during IR, likely by influencing oxygen consumption, platelet aggregation, leukocyte adhesion, and free radical scavenging. Paradoxically, in high concentrations, NO may potentiate ROS-mediated toxicity by promoting the formation of highly reactive species, such as peroxynitrite. Beyond NO, the coronary endothelium has several other pathophysiologic roles in IR, such as serving as a source of vasoactive substances and by activating the immune system through expression of cytokines, chemokines, and adhesion molecules.
Recent work has implicated autophagy, an evolutionarily ancient mechanism of controlled cellular cannibalism, in the pathogenesis of IR. Time will tell whether this mechanism is a suitable target for therapeutic manipulation in this and other heart disease–related contexts.
Endothelial activation and injury increase vascular permeability and recruitment of inflammatory cells. Cellular adhesion molecules elicited by the injured endothelium (e.g., intercellular cell adhesion molecule–1, vascular cell adhesion molecule–1, E-selectin) promote tissue invasion by inflammatory cells. These infiltrating cells, including (and in particular) neutrophils, are directly toxic to the myocardium by secreting proteases, generating ROS, and occluding the microvasculature. Other components of the innate immune system, such as Toll-like receptors, mannose-binding lectin, and the complement cascade, also appear to participate in the pathogenesis of IR stress. Additionally, there is a growing appreciation of the role of cell-mediated immunity (i.e., T-cells and macrophages) in the pathogenesis of myocardial damage after reperfusion.
Mechanisms of Ischemia-Reperfusion Injury
Molecular and cellular events underlying IR injury are complex, representing the confluence of divergent biologic pathways. Furthermore, the extent to which each of these pathways is relevant to human disease remains unclear, as animal models do not always faithfully recapitulate the IR disease process in humans. These limitations notwithstanding, several key pathophysiologic features of clinically relevant IR have emerged ( Table 1 ).
• Ion accumulation |
∘ Intracellular calcium overload |
∘ Increased intracellular sodium |
∘ Decrease in pH with rapid normalization upon reperfusion |
• Dissipation of mitochondrial membrane potential |
∘ mPTP |
• Free radical formation/ROS |
∘ Generation from xanthine oxidase |
∘ Release of reactive mitochondrial intermediates |
∘ Neutrophil infiltration |
∘ ROS-induced ROS |
• NO metabolism |
∘ Loss of NO-vasodilation |
∘ Accumulation of reactive peroxynitrite |
• Apoptosis and autophagy |
• Endothelial dysfunction |
∘ Cytokine and chemokine signaling |
∘ Expression of cellular adhesion markers |
∘ Impaired vasodilation |
• Platelet aggregation and microembolization |
• Immune activation |
∘ Innate immunity (e.g., complement activation, expression of Toll-like receptors) |
∘ Neutrophil accumulation |
∘ Cell-mediated damage (macrophage and T cell) |
Ischemia induces the accumulation of intracellular sodium, hydrogen, and calcium ions, culminating in tissue acidosis. Reperfusion, in turn, elicits rapid alterations in ion flux, and some evidence suggests that rapid renormalization of pH paradoxically leads to enhanced cytotoxicity. Sodium-dependent pH regulatory mechanisms, including the Na + -H + exchanger and the Na + -HCO 3 − transporter, are activated, which consequently leads to intracellular sodium accumulation. High sodium concentrations, in turn, drive increases in sarcoplasmic reticular Ca 2+ by the Na + -Ca 2+ exchange. Enhanced Ca 2+ entry by sarcolemmal L-type Ca 2+ channels and a deficient import of cytosolic Ca 2+ into the sarcoplasmic reticulum by sarcoplasmic/endoplasmic reticulum Ca 2+ –adenosine triphosphatase further promote Ca 2+ overload. The result is myofibrillar hypercontractility, adenosine triphosphate depletion, ultrastructural damage to mitochondria, and myocardial stunning.
Cardiac myocytes consume large quantities of energy. To accommodate this requirement, these cells host a high density of mitochondria. Thus, it is not surprising that these complex, energy-generating organelles, filled with reactive intermediates and proapoptotic signals, are intimately involved in IR injury. As part of this, the mitochondrial permeability transition pore (mPTP) has been the center of a growing amount of attention. The inner mitochondrial membrane, responsible for maintaining mitochondrial transmembrane potential, is normally impermeable to ions and proteins. Dissipation of the electrical potential across this membrane is termed “permeability transition,” a process thought to be mediated through the mPTP. Although the constituent protein components of the pore remain unknown, formation of the pore creates a nonselective channel between the inner membrane of the mitochondrion and the sarcoplasm. This results in loss of the electrochemical gradient, the release of reactive oxygen species (ROS), and apoptosome formation. Triggers for mPTP include Ca 2+ overload, rapid normalization of pH, and oxidative stress.
The generation of free radicals through incomplete reduction of oxygen during IR has been well described. These oxygen species are highly reactive and can quickly overwhelm a cell’s endogenous free radical scavenging system. This in turn triggers cellular injury by reactions with lipids, proteins, and nucleic acids. The enzyme xanthine oxidase has been particularly implicated as a generator of free radicals in the reperfused heart, as its substrates (xanthine and hypoxanthine) accumulate during ischemia. In addition to damaging nuclear and cytosolic elements, ROS can trigger the opening of the mPTP. This results in a positive feedback loop of additional free radical release from the mitochondria (“ROS-induced ROS release”).
Not only is IR injury dependent on events occurring within cardiomyocytes, but the endothelium is an active participant as well. The endothelium is the major source of the evanescent molecule, nitric oxide (NO). Under normal conditions, NO generation elicits vasodilation, which has beneficial, protective effects during IR, likely by influencing oxygen consumption, platelet aggregation, leukocyte adhesion, and free radical scavenging. Paradoxically, in high concentrations, NO may potentiate ROS-mediated toxicity by promoting the formation of highly reactive species, such as peroxynitrite. Beyond NO, the coronary endothelium has several other pathophysiologic roles in IR, such as serving as a source of vasoactive substances and by activating the immune system through expression of cytokines, chemokines, and adhesion molecules.
Recent work has implicated autophagy, an evolutionarily ancient mechanism of controlled cellular cannibalism, in the pathogenesis of IR. Time will tell whether this mechanism is a suitable target for therapeutic manipulation in this and other heart disease–related contexts.
Endothelial activation and injury increase vascular permeability and recruitment of inflammatory cells. Cellular adhesion molecules elicited by the injured endothelium (e.g., intercellular cell adhesion molecule–1, vascular cell adhesion molecule–1, E-selectin) promote tissue invasion by inflammatory cells. These infiltrating cells, including (and in particular) neutrophils, are directly toxic to the myocardium by secreting proteases, generating ROS, and occluding the microvasculature. Other components of the innate immune system, such as Toll-like receptors, mannose-binding lectin, and the complement cascade, also appear to participate in the pathogenesis of IR stress. Additionally, there is a growing appreciation of the role of cell-mediated immunity (i.e., T-cells and macrophages) in the pathogenesis of myocardial damage after reperfusion.
Ischemia-Reperfusion in Acute Myocardial Infarction
Although “reperfusion injury” in the most general sense refers to that component of the infarction process related to restoration of epicardial patency and anterograde blood flow, in the catheterization laboratory, “IR injury” is often synonymous with the “no-reflow” phenomenon. The term was first applied to myocardial ischemia after coronary ligation in dogs. Regarded as a dreaded complication of acute MI intervention, it is estimated to occur in >30% of cases and is associated with adverse prognosis. No reflow is thought to be related in part to microvascular plugging by vasoactive debris. Although dramatic, no reflow is probably just the most angiographically apparent form of IR injury in acute MI, and it should be recognized that significant reperfusion injury occurs even without the obvious “hang-up” of contrast dye.
Deciphering the contribution of IR to myocardial infarct size in humans is more challenging than in animal models. Acute MI in humans is generally associated with thrombotic occlusion of an epicardial coronary, and this prothrombotic and proinflammatory event is not well captured in models involving surgical ligation of the artery. This may be particularly important, as microvascular plugging with leukocytes and platelet “debris” has been implicated as an important component of the IR process. Another complexity relates to patient co-morbidities that influence the myocardial substrate during IR. Factors such as left ventricular hypertrophy, diabetes mellitus, and chronic ischemia preceding artery total occlusion (i.e., recapitulating ischemic preconditioning) potentially influence sensitivity to IR injury. The resulting heterogeneity in the human myocardial phenotype renders analyses of experimental models challenging and limits the degree to which the findings can be extrapolated to the human case. Finally, the emergent nature of most acute MIs (ST-segment elevation MIs in particular) makes it challenging, often both ethically and logistically, to study these patients, as issues arise of informed consent and seeming coercion during the race to achieve vessel patency. Nonetheless, these limitations have not precluded the testing of a series of therapeutic interventions in patients over the past 3 decades.
Therapeutic Interventions Targeting Ischemia-Reperfusion Injury in Acute Myocardial Infarction
Despite the substantial progress in understanding mechanisms of IR on the basis of models of acute MI, and the associated enthusiasm for translating these findings into patient care, the results of clinical studies have been largely disappointing. Whether this reflects our still incomplete understanding of the biology of IR or just a naive belief that a single intervention could be protective against a process involving multiple major pathophysiologic components is not clear. Initial pilot successes have been met with subsequent failures in larger confirmatory trials. The results of these trials have been summarized elsewhere, but interventions have included a spectrum of targets, including oxidant, inflammatory, sodium-hydrogen exchange, NO metabolism, and metabolic pathways ( Table 2 ).
Acute MI |
• Anti-inflammatory |
∘ Inhibition of leukocyte accumulation (e.g., anti-CD11/CD18) |
∘ Complement inhibition (e.g., pexelizumab) |
• Increasing local adenosine concentrations |
∘ Systemic infusions |
∘ Intracoronary bolus dose |
• Inhibition of mPTP |
∘ Cyclosporine |
• Ischemic postconditioning |
∘ Repetitive balloon inflations |
• NO metabolism |
∘ Nitroprusside |
• Prevention of intracellular calcium overload |
∘ Inhibition of Na + -H + exchange (e.g., cariporide, eniporide ) |
∘ Calcium channel blockers |
• Reducing ROS |
∘ Inhibition of xanthine oxidase (e.g., allopurinol ) |
• Vasodilation |
∘ Potassium channel opening (e.g., nicorandil ) |
Cardiac surgery |
• Metabolic additives in cardioplegic solutions |
∘ Pyruvate |
∘ Amino acids |
• Ischemic preconditioning |
∘ Remote preconditioning |
∘ Repetitive aortic cross clamping |
• Volatile anesthetics |
• Anti-inflammatory |
∘ Leukocyte filters |
∘ Steroids |
∘ Complement inhibition |
• Prevention of intracellular calcium overload |
∘ Pyridoxal-5′-phosphate |
∘ Calcium channel blockers |
∘ Inhibition of Na + -H + exchange (e.g., cariporide ) |
• Increase local adenosine levels |
∘ Acadesine |
Despite these setbacks, investigation continues in this field. Erythropoietin, for instance, is currently being investigated in clinical studies of acute MI, after the discovery of erythropoietin receptor expression in the myocardium. Erythropoietin has antiapoptotic activity and positive effects on remodeling, and it recruits endothelial progenitor cells. It is hoped that this drug may exert positive effects by more than 1 of these pathways. Other therapeutic strategies currently in clinical trials include the interleukin-1 receptor antagonist anakinra and glucagon-like peptide–1 analogues.
Although most pharmacologic interventions have been administered systemically, the availability of primary percutaneous coronary revascularization has allowed the direct administration of drug to the coronary endothelium and myocardium. One well-studied drug is adenosine. Adenosine, in addition to its well-known vasodilating properties, is intimately involved with preconditioning and postconditioning (see the following discussion), as well as inhibition of mPTP opening. Although systemic adenosine infusion did not reduce overall mortality in the Acute Myocardial Infarction Study of Adenosine II (AMISTAD-II), some effect on infarct size was noted. Subsequent smaller scale investigation into the potential adjunctive role of intracoronary administration has demonstrated some benefit with regard to electrocardiographic and angiographic end points in the setting of acute MI. Similarly, there has been some enthusiasm, although muted somewhat by the small-scale nature of the studies, for intracoronary nitroprusside in the treatment of no reflow. This direct NO donor may be of particular benefit when coadministered with intracoronary adenosine.
Ischemic postconditioning, a process wherein the myocardium is subjected to repetitive bouts of iatrogenic IR during the course of an ongoing acute MI, is also under clinical investigation. This procedure involves several short intracoronary balloon inflations after primary vessel patency has been reestablished. Data from animal models suggest that final infarction size is diminished by this strategy, and some preliminary data in humans appear promising. The mechanism of benefit is unclear, but it may involve the induction of a more gradual pH shift in the myocardium or decreasing ROS and Ca 2+ -induced mPTP opening.
There is an ever growing focus on the role of mPTP in IR. This structure, as outlined previously, appears to be the common effector of a series of upstream intracellular signals and therefore has obvious appeal as a target of therapy. Recently, the results of a pilot study of intravenous cyclosporine A, a nonspecific inhibitor of the mPTP, during ST-segment elevation MI were reported. Although a small study, the results were promising, suggesting that the inhibition of mPTP may be of benefit in larger trials.
Ischemia-Reperfusion Injury During Cardiac Surgery
IR stress caused by cardiac surgery is distinctly different from that occurring during spontaneous MI. Ischemia is induced artificially by aortic cross clamping, and myocardial preservation strategies are used throughout this ischemic period. Cardioplegia is achieved via hyperkalemic, hypothermic cardiac arrest and maintained with the intermittent use of a glucose-containing cardioplegic solution (usually mixed with blood) delivered anterograde in the aortic root and/or retrograde via the coronary sinus. These maneuvers are designed to minimize myocardial metabolic activity and consequent oxygen demand during this period of myocardial vulnerability.
Once surgery has been completed, the aortic cross clamp is released, and the heart is suddenly and globally reperfused with blood that is fully anticoagulated, immunologically primed by exposure to the cardiopulmonary bypass circuit, and characterized by a very high partial pressure of oxygen. As a result, the post–cardiac surgery myocardium is exposed to dramatic extremes of ischemia and reperfusion. And again, it is important to recognize that hearts that undergo cardiac surgery are highly heterogenous, ranging across a spectrum of co-morbidities, hypertrophy, and contractile function. Patients who undergo aortic arch surgery may have essentially normal hearts, whereas other patients may be chronically ischemic with severe contractile dysfunction. These tissue substrates would be expected to react differently to such extremes of environmental stress.
Clinically, IR injury after cardiac surgery can manifest as arrhythmia, myocardial stunning, low cardiac output, and perioperative MI. Among patients dying soon after coronary artery bypass surgery (coronary artery bypass grafting [CABG]), histologic evidence of IR on autopsy is detected in 25% to 45% of patients. Furthermore, biochemical evidence of myocardial injury (e.g., elevated levels of circulating creatine kinase-MB and/or troponin) has been clearly linked with adverse events after cardiac surgery.
Interventions Targeting Ischemia-Reperfusion Injury After Cardioplegic Arrest
Although the operating theater may appear daunting given the number of personnel, environmental factors, and pieces of equipment needed to successfully manage a patient through cardiac surgery, it is, in many ways, an ideal place to perform research. The duration of ischemia is known, electrolytes and glucose concentrations are meticulously regulated, and hemodynamics can be followed throughout the procedure, including the pre- and postoperative phases. Furthermore, local drug delivery, as opposed to systemic therapy, can be administered reliably via the cannulated coronary circulation.
The cornerstone of cardioprotection in cardiac surgery has been the cardioplegia solution. These solutions typically contain potassium, mannitol, and glucose. By arresting and cooling the heart, the metabolic demands of the myocardium are minimized. However, despite decades of experience with cardioplegia, there has been surprisingly little change in these formulations except for, perhaps, the adoption of blood cardioplegia. And despite the use of cardioplegia, IR injury still occurs.
There are some small studies supporting the use of metabolic additives, such as pyruvate and glutamate or aspartate. The goal of such metabolic supplementation is to provide the arrested myocardium with energy substrates with favorable characteristics (e.g., avoidance of fatty acid oxidation) and to replete the cell with anaplerotic substrates. However, evidence to date supporting the use of these agents has not been sufficiently convincing to lead to widespread clinical use.
The concept of ischemic preconditioning has also been applied during cardiac surgery. Either before or just after the establishment of cardiopulmonary bypass, the aorta is cross clamped for several minutes, released for several minutes, and then once again cross clamped for cardioplegia. Several groups have used this technique, and some benefit has been seen in small studies. A recent meta-analysis concluded that this form of preconditioning decreased postoperative ventricular arrhythmias and inotrope use and translated into shorter intensive care unit stays. These suggestive findings await prospective testing in patients.
Given the association of immune system activation with IR injury, together with the marked systemic inflammation elicited by surgery and by exposure to the bypass circuit, a number of investigators have focused their attention on modulating immune responses. Leukocyte filters, steroids, and specific volatile anesthetics have all been tested in small studies with no clear signal of benefit. Initial data on the anti-C5a antibody pexelizumab appeared promising and prompted 2 large randomized clinical trials. The Pexelizumab in Patients Undergoing Coronary Artery Bypass Grafting With Cardiopulmonary Bypass (PRIMO-CABG) trial demonstrated a strong trend toward decreasing death or MI, but these results were not confirmed in the larger follow-up PRIMO-CABG II study. Finally, aprotinin, an antifibrinolytic protein with anti-inflammatory properties, was documented to have favorable effects on myocardial IR in human and animal studies but was later taken off the market because of clear evidence for harm associated with its use. In aggregate, the results of inhibiting the inflammatory response occurring in cardiac surgery have been disappointing.
The naturally occurring pyridoxine metabolite and purinergic receptor antagonist pyridoxal-5′-phosphate, was studied in 2 large CABG clinical studies. Pyridoxal-5′-phosphate was found to prevent intracellular Ca 2+ overload and appeared promising for mitigating IR injury. Indeed, phase II data from the MC-1 to Eliminate Necrosis and Damage in Coronary Artery Bypass Graft Surgery (MEND-CABG) I study suggested lower postprocedural infarct sizes. On the basis of this result, a larger phase III study was undertaken. Disappointingly, however, MEND-CABG II failed to find any difference in infarct size and reported a slight early increase in mortality with pyridoxal-5′-phosphate (1.0% vs 0.3%, p = 0.03).
Another strategy to prevent intracellular Ca 2+ overload is antagonism of the sodium-hydrogen exchanger. Cariporide, a potent inhibitor of this transporter, was first evaluated in the Guard During Ischemia Against Necrosis (GUARDIAN) trial, a catchall study of patients with non-ST-segment elevation acute coronary syndromes or planned elective revascularization with either percutaneous coronary intervention or CABG. An efficacy signal was noted for the CABG subset, and the drug was subjected to a larger trial dedicated to CABG. The primary end point in the Sodium-Proton Exchange Inhibition to Prevent Coronary Events in Acute Cardiac Conditions (EXPEDITION) trial (death or MI) was lower in the cariporide arm compared to placebo (16.6% vs 20.3%, p = 0.0002), the first time a phase III clinical study of myocardial protection had met its primary end point. However, whereas the composite end point was driven by a significant reduction in perioperative MI (18.9% vs 14.4%, p <0.0001), there was a paradoxical increase in overall mortality (2.2% vs 1.5%, p = 0.02), which appeared to be driven by an increase in cerebrovascular events. Indeed, there was a significant increase in stroke and altered mental status associated with the drug.
Perhaps the most thoroughly studied drug in surgical IR is acadesine, a purine analogue that increases tissue adenosine levels in energy-deprived tissues. A potent cardioprotective role has been ascribed to the stimulation of adenosine receptors in IR via modulation of mPTP opening. Acadesine, given as an additive in cardioplegia solution, has been investigated in a number of smaller studies, as well in as the approximately 2,700-patient phase III Acadesine 1024 Trial. Although the largest study failed to show a statistically significant difference in the primary outcome of periprocedural MI (3.4% vs 4.0%, p = 0.24, in favor of acadesine), a subsequent meta-analysis of all available data on acadesine suggested a 27% reduction in MI (3.6% vs 4.9%, p = 0.02) and a 26% decrease in the combined outcome of stroke, MI, or cardiac death (7.6% vs 4.6%, p = 0.04). Acadesine is currently being studied in the Reduction in Cardiovascular Events by Acadesine in Subjects Undergoing CABG (RED-CABG) trial, which plans to enroll 7,500 high-risk subjects.
Acknowledgment
We thank Lynne Isbell, PhD, at Discovery Chicago, for editorial support.
This work was supported by grants HL-075173 , HL-080144 , and HL-090842 from the National Institutes of Health , Bethesda, Maryland; grant 0640084N from the American Heart Association , Dallas, Texas; grant 7-08-MN-21-ADA from the American Diabetes Association , Alexandria, Virginia; and grant 0970518N from the AHA-Jon Holden DeHaan Foundation , Naples, Florida. Editorial support was funded by Schering-Plough Corporation , Kenilworth, New Jersey.
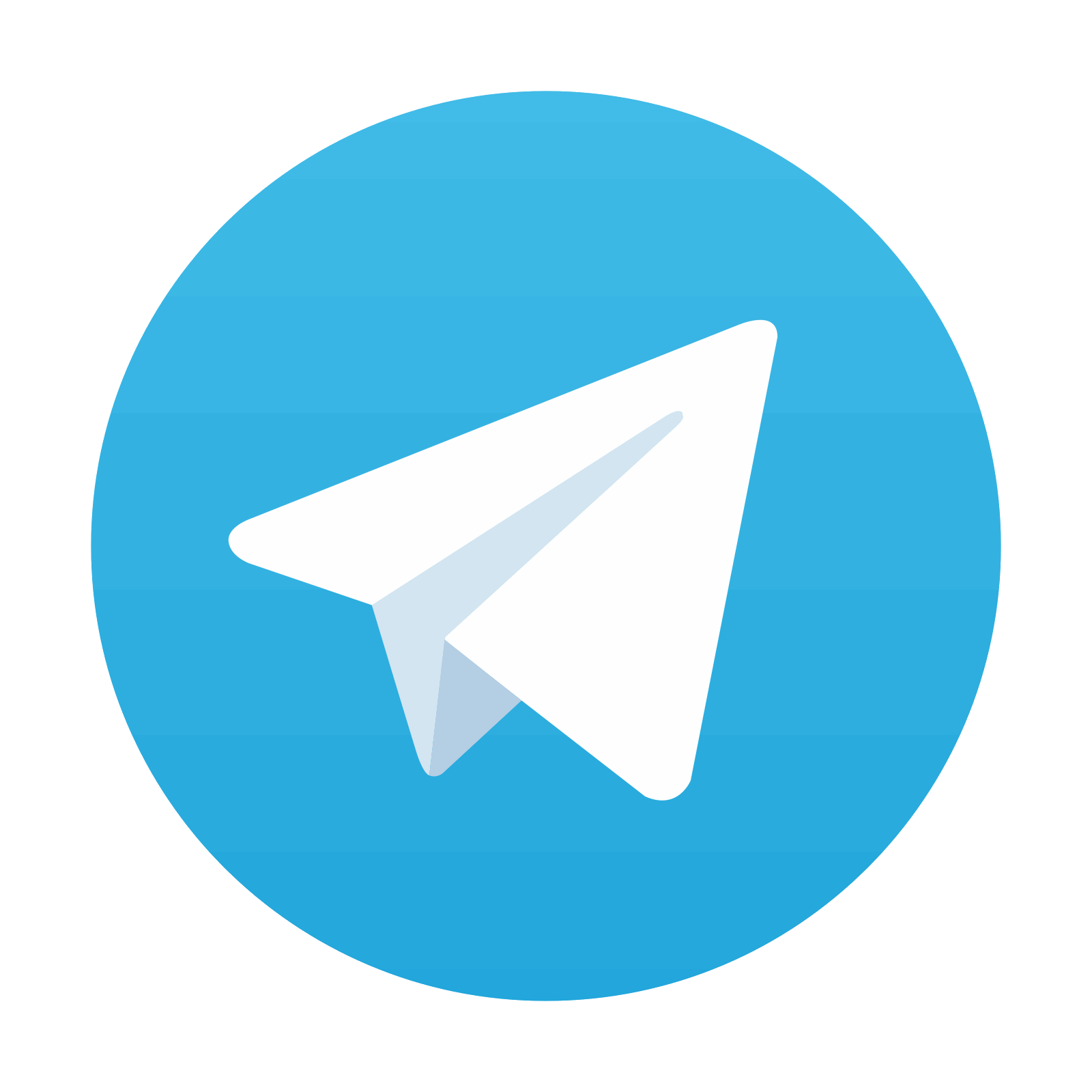
Stay updated, free articles. Join our Telegram channel

Full access? Get Clinical Tree
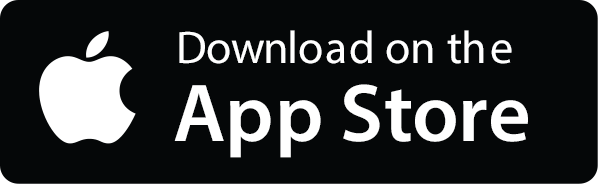
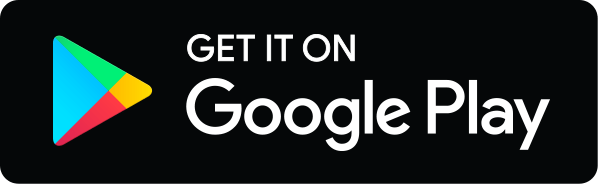