Fig. 4.1
Schematic drawing of the proposed molecular pathogenesis of IPF. AEC, alveolar epithelial cell; EMT, epithelial-mesenchymal transition; ECM, extracellular matrix
4.2 Epithelial Injury and Genetic Background
Studies of the genetic backgrounds of patients with familial pulmonary fibrosis have revealed the existence of germline mutations in several genes. Surfactant proteins SP-A, SP-B, SP-C, and SP-D are produced from AECII and play roles in preserving lung homeostasis [5]. SP-B and SP-C are extremely hydrophobic and essential for reducing surface tension, whereas SP-A and SP-D belong to the collectin family of proteins and are involved in the host defenses. Among these genes, the SP-C gene mutation was first reported in children with familial pulmonary fibrosis [6, 7]. A mutation in the SP-A2 gene was subsequently identified in cases of familial disease [8, 9]; these mutations in the SP genes cause the accumulation of misfolded proteins in AECII. Other mutations have since been reported in telomerase genes [10, 11]. These mutations are believed to be associated with a reduced enzyme activity of telomerase and inversely correlate with the length of the telomere. An analysis of terc −/− mice also showed a reduced number of AECII in the distal lung epithelium [12]. Furthermore, the ATP-binding cassette protein A3 (ABCA3) is highly expressed in AECII and thought to be involved in the transport of surfactant proteins. The mutation in the ABCA3 gene was originally reported to cause the fatal interstitial lung disorder, whereas a later report showed an association with pediatric interstitial lung disease [13, 14]. More recently, a common MUC5B promoter polymorphism was identified to be associated with familial interstitial pneumonia, although the role of MUC5B in the pathogenesis of IPF remains unclear [15].
The above gene mutations are thought to render AEC susceptible to various stimuli, such as genetic predispositions. However, the frequency of these gene mutations appears to be rare in sporadic cases of IPF [16].
4.3 Biological Alteration in Injured AEC in IPF
Gene mutations in SP-C or SP-A2 cause the production of misfolded proteins that are not secreted or accumulated in the endoplasmic reticulum (ER) of AECs [17, 18]. Subsequently, the response to ER stress is induced, thereby upregulating the expressions of XBP-1, GRP78/BiP, and HDJ-2/HSP40, followed by the activation of caspase3 and apoptosis. Increased ER stress is seen in epithelial cells in the lungs of patients with IPF [19, 20], and apoptosis is reported to be increased in AECs in IPF lungs [21]. ER stress is enhanced by numerous factors. For example, viral infections, such as that with the influenza virus and cytomegalovirus, stimulate ER stress in AECs [20, 22]. In addition, environmental exposure to toxins, including cigarette smoke, upregulates ER stress in AECs [23], and aging is related to enhanced susceptibility to ER stress induced by murine herpesvirus [24]. These findings suggest that ER stress and apoptosis in AECs may be critical in both sporadic and familial cases of IPF and that similar mechanisms leading to ER stress, such as SP genes, may function in sporadic cases.
How do AECs under ER stress or apoptosis promote pulmonary fibrosis? The answer is not fully understood. However, it was recently demonstrated that the expression of TGF-β is mediated by ER stress associated with the SP-A2 gene mutation and influenza infection in AECs [20, 25]. ER stress may also generate EMT of AECs [26]. Since AECs have various biological functions, further research is required to clarify the link with epithelial injury and pulmonary fibrosis.
4.4 Critical Roles of Growth Factors in the Progression of Pulmonary Fibrosis
As mentioned above, injury to AECs is believed to be the first event triggering the onset of pulmonary fibrosis. However, growth factors are produced by various cells in addition to AECs in the IPF lungs [27]. Alveolar macrophages are known to produce numerous soluble factors under conditions of fibrogenesis in the lungs. Furthermore, emerging cells called fibrocytes also have the ability to produce profibrotic growth factors.
Among profibrotic mediators, transforming growth factor (TGF)-β and PDGF are thought to play a critical role in the onset of fibrogenesis of the lungs due to the activation of lung fibroblasts. Despite the limited evidence, other growth factors, including fibroblast growth factor (FGF), vascular endothelial growth factor (VEGF) and insulin-like growth factor (IGF)-1, etc., are also believed to contribute to the pathogenesis of pulmonary fibrosis.
Representative growth factors with a profibrotic activity are summarized in Table 4.1.
Table 4.1
Major growth factors related to IPF and their biological effects
Growth factor | Producing cells | Biological activities | |||||||
---|---|---|---|---|---|---|---|---|---|
Endothelial cells | Epithelial cells | Fibroblasts | Fibrocyte | Myofibroblasts | Proliferation | Migration | Collagen production | Fibrosis | |
TGF-β | + | + | + | + | + | → | ↑ | ↑↑ | ↑ |
PDGF | + | + | + | + | + | ↑↑ | ↑↑ | ↑ | ↑ |
FGF2 | – | – | + | + | + | ↑ | ↑ | ↑ | ↑? |
4.4.1 PDGF and PDGF Receptors
PDGF is a homo- or heterodimeric molecule with a molecular weight of 30 kDa [28]. PDGF genes consist of four different genes, including PDGF-A, PDGF-B, PDGF-C, and PDGF-D, which are located on chromosomes 7, 22, 4, and 11, respectively [29, 30]. There are two types of PDGF receptors (PDGFRs), α and β, which have a molecular weight of 170–180 kDa and are composed of homo- or heterodimers. Possible PDGF-PDGFR interactions are multiple and complex according to an in vitro study [7]. However, an in vivo study showed a limited pattern of binding of PDGF-AA or –CC to PDGFR-α and PDGF-BB to PDGFR-β [30].
PDGFs are expressed in many types of cells, including fibroblasts, vascular endothelial cells, macrophages, and platelets/megakaryocytes [30]. It is also known that the expression of PDGFs is upregulated by various inflammatory cytokines and growth factors, including both TGF-β and PDGF. Moreover, PDGFRs are expressed in various cells, although the classical targets of PDGF are fibroblasts and smooth muscle cells, and the expression of PDGFRs is induced by various stimuli. In contrast to PDGFs, the expression of PDGFR in some cells is limited to PDGFR-α or PDGFR-β, not both.
PDGF is known to be a major mitogen for mesenchymal cells. In fact, PDGF is the strongest stimulus of the proliferation of fibroblasts [28, 30], and the depletion of the PDGF-A gene in mice has been shown to be homozygous and lethal, with two different restriction points, one located prenatally and one located postnatally [31]. Postnatally surviving PDGF-A-deficient mice develop lung emphysema secondary due to the loss of alveolar myofibroblasts containing PDGFR-α. On the other hand, PDGFR-a null mice show cranial malformations and deficiency of myotome formation [32]. Mice deficient for PDGF-B or PDGFR-β exhibit renal, cardiovascular, and hematological, but not pulmonary, abnormalities [33, 34]. In summary, PDGF-A/PDGFR-α pathway plays a role in the secondary septation process, since PDGFR-α-expressing cells located in the alveolar entry ring have characteristics of myofibroblasts [35].
PDGF is known to be a growth factor that plays a role in the pathogenesis of pulmonary fibrosis [28, 30]. In animal models, the induction of pulmonary fibrosis with bleomycin (BLM) has been used to assess the molecular pathogenesis. For example, Maeda et al. reported that the expression of the PDGF-A gene is increased in mice showing BLM-induced pulmonary fibrosis using semiquantitative reverse transcriptase polymerase chain reaction (RT-PCR) [36]. Walsh et al. also examined the bronchoalveolar lavage fluid of rats treated with BLM and found 38- to 40-kDa and 29-kDa peptides detected with anti-PDGF-BB and anti-PDGF-AA antibodies, respectively, demonstrating the growth-promoting activity of lung fibroblasts [37]. In that study, the growth-promoting activity was neutralized 64 % by the anti-PDGF-BB antibody and 15 % by the anti-PDGF-AA antibody. In contrast, Zhuo et al. showed that the PDGF-C, but not the PDGF-A, PDGF-B, or PDGF-D, gene is induced in the lungs of mice treated with BLM according to a Northern blot analysis [38]. Moreover, Shimizu et al. reported that PDGF-A and PDGF-B are induced and elevated in the BLM-treated lungs of mice at both the mRNA and protein levels [39]. Additionally, the adoptive transfer of an adenovirus expressing the PDGF-B gene into the lungs induces severe fibrosis in mice [40]. Based on these reports, the expression of PDGF isoforms is enhanced in the setting of fibrogenesis of the lungs, although the details require a further analysis.
On the other hand, an enhanced expression of PDGF in epithelial cells and alveolar macrophages in the lungs of patients with IPF has been reported [41, 42]. However, the mechanisms involved in the enhanced expression and actions of PDGF in the fibrotic lung are poorly understood. Recently, Gochuico et al. examined growth factors in the alveolar lining fluid of patients with rheumatoid arthritis complicated with pulmonary fibrosis and reported that PDGF-AB and PDGF-BB, but not TGF-β or PDGF-AA, are associated with the progressive stage of pulmonary fibrosis [43], indicating the importance of PDGF-B in the onset of fibrogenesis of the lungs.
The evidence described above suggests that targeting the PDGF/PDGFR signaling pathway may have therapeutic effects against pulmonary fibrosis. This hypothesis has been investigated using animal models of pulmonary fibrosis with specific inhibitors of PDGFR [44]. Rice et al. first reported that AG1296, a tyrosine kinase inhibitor (TKI) for PDGFR, prevents the development of pulmonary fibrosis induced by vanadium pentoxide (V2O5) in rats [45]. In addition, imatinib mesylate (Gleevec in the United States, Glivec in Europe) has been applied, as imatinib inhibits PDGFR in addition to bcr-abl and c-kit [46]. The antifibrotic effects of imatinib in various pulmonary fibrosis models have been extensively examined, and it has been reported that imatinib strongly inhibits fibrogenesis in the lungs [47–49]. In addition, Yoshida et al. reported that the in vivo gene transfer of an extracellular domain of PDGFR-β reduces the onset of BLM-induced pulmonary fibrosis [50]. Recently, nilotinib, another compound with a similar profile to that of imatinib, was also reported to show a higher antifibrotic activity than imatinib [51]. These observations suggest that the PDGF/PDGFR axis is a potential therapeutic target for pulmonary fibrosis.
4.4.2 FGF and FGF Receptors
FGF and FGF receptor (FGFR) are thought to be involved in the onset of fibrogenesis in the lungs. The FGF/FGFR family is composed of 18 FGF ligands and four FGFRs [52, 53]. Alternative splicing of the domain III of FGFR1–3 yields two different isoforms, IIIb in epithelial tissue and IIIc in mesenchymal tissues. Heparan sulfate glycosaminoglycan (HSGAG) binds to both FGF and FGFR in order to stabilize the binding of FGF to FGFR by facilitating dimerization. The specificity of different FGFs for different receptor isoforms has also been reported [54].
The physiological functions of FGF are diverse and complex, and studies have evaluated the roles of FGF proteins in vitro and in genetically modified mice in vivo [52–54]. Since FGFs originally possess mitogenic, chemotactic, and angiogenic activities, the biology of FGF has been examined in cancer research. In addition, knockout mice have been used to demonstrate the role of FGFs in the embryonic development of various organs. However, the physiological roles of most FGFs/FGFRs remain unclear. Both Fgf1 −/− and Fgf2 −/− mice are viable and fertile, with the exception of some abnormalities in the vascular system, although exogenous FGF-2 induces the proliferation of endothelial cells, smooth muscle cells, and fibroblasts.
The profibrogenic activities of FGFs have been observed in FGF-2 (basic FGF). For example, Hetzel et al. reported the proliferative activity of FGF-2 for lung fibroblasts [55], and fibroblasts derived from IPF show a reduced response to FGF-2, IGF-1, and EGF, but not PDGFs, as compared with normal fibroblasts. Meanwhile, Kanazawa et al. reported the activity of these compounds in promoting the migration of skin fibroblasts [56]. However, FGF-2 does not stimulate the production of fibronectin by lung fibroblasts. In IPF patients, there are scant data regarding the localization of FGF-2 in the lungs. However, the expression of FGF-2 mRNA has been reported to be upregulated in BLM-treated lungs in mice [57]. Although immunohistochemical staining has been reported to show the expression of FGF-2 in inflammatory cells, the role of the FGF-2/FGFR axis in mouse models of lung fibrosis remains unclear. Ju et al. reported that the administration of soluble FGFR2c ectodomain significantly reduces the extent of lung fibrosis in TGF-β-induced lung fibrosis mice [58]. However, Guzy et al. recently reported that Fgf2−/− mice show increased mortality due to epithelial injury induced by BLM without any effects on lung fibrosis [57]. At present, it is not possible to draw firm conclusions regarding the role of FGF-2 in the pathogenesis of pulmonary fibrosis in mice or in humans.
In contrast to FGF-2, FGF-1 has been reported to be an antifibrotic factor. FGF-1 reverts the profibrogenic effects of TGF-β such as α-SMA induction [59]. In addition, FGF-1 reduces the expression of collagen-I and induces the apoptosis of lung fibroblasts [60, 61].
It is currently difficult to determine the overall roles of FGF/FGFR in the pathogenesis of IPF. Further studies with an inhibitor specific for FGFR in pulmonary fibrosis models may help to clarify the roles of FGF/FGFR, although each isoform of FGF/FGFR cannot be analyzed minutely.
4.4.3 VEGF and VEGF Receptors
VEGF and VEGF receptors play a central role in both physiological and pathological angiogenesis [62]. The VEGF family is composed of seven members, VEGF-A, VEGF-B, VEGF-C, VEGF-D, VEGF-E, PIGF, and svVEGF, while VEGF receptors include three members, VEGFR1, VEGFR2, and VEGFR3. Within the VEGF family, VEGF-A apparently serves as a key player in angiogenesis. Ebina et al. reported that the number of CD34-positive vessels is increased in the early phase of IPF of the lungs [63]. The role of angiogenesis in the development of lung fibrogenesis has not yet been determined, although Antoniou showed elevated VEGF levels in the BAL in patients with IPF [64]. There is limited evidence concerning the relationship between VEGF and lung fibrosis. Farkas et al. reported the direct effects of VEGF-A on lung fibroblasts and found that VEGF-A enhances the collagen-I expression induced by TGF-β [65]. In addition, Hamada et al. demonstrated that gene therapy with the soluble flt-1 gene reduces pulmonary fibrosis in a BLM-induced model in mice [66]. On the other hand, Ou et al. reported that the VEGFR-2 antagonist SU5416 attenuates BLM-induced lung fibrosis in mice [67]. These reports suggest the possibility of the profibrotic effects of VEGF, although the effects are unknown with respect to the role of each isoform of VEGF/VEGFR.
4.4.4 TGF-β and TGF-β Receptors
The signaling pathway via the TGF-β/TGF-β receptor complex is complicated [68, 69]. Human TGF-β ligand has three isoforms TGF-β1, TGF-β2, and TGF-β3, among which TGF-β1 has been reported to be a predominant isoform in cases of pulmonary fibrosis [70]. The TGF-β ligand binds heterodimeric serine/threonine kinase receptors of the TGF-β receptor I (TβRI) (ALK5) and TβRII. The binding of TGF-β to these receptors is regulated by several molecules, including latent TGF-β binding protein (LTBP) and integrins αVβ6. The signaling pathway in the cytoplasm is also complex. The classical Smad pathway and non-Smad pathway differentially regulate the profibrotic effects of TGF-β. The biological activities of TGF-β are extremely pleiotropic and dependent on the type of cell and pathological condition. Representative activities are known to inhibit cell proliferation, regulate the extracellular matrix, suppress the immune response, and induce the epithelial-mesenchymal transition (EMT) [69]. Therefore, TGF-β is believed to play a central role in the pathogenesis of lung fibrosis.
The expression of TGF-β has been reported to be upregulated in the IPF lungs, particularly in AECs [71]. TGF-β stimulates the migration and production of collagen in lung fibroblasts [68, 69]. In addition, TGF-β inhibits the apoptosis of myofibroblasts. The involvement of TGF-β in lung fibrosis was recently demonstrated using BLM models of pulmonary fibrosis [72–74]. However, Andorianifahanana et al. showed that profibrotic TGF-β responses require the cooperative actions of PDGF and ErbB receptor tyrosine kinases [75]. Of course, the TGF-β function is partly mediated by the productions of CTGF and FGF-2 [76, 77]. Based on these reports, the profibrotic effects of TGF-β are mediated by several other growth factors, including PDGF, EGF, CTGF, and FGF-2, indicating that the role of TGF-β in lung fibrogenesis may have been overestimated. The other concern in targeting therapy for TGF-β is the paradoxical induction of persistent inflammation by blocking TGF-β signals [78, 79]. Therefore, further evidence is required to completely understand and control TGF-β signals.
4.4.5 Origin of Lung Fibroblasts and Their Contribution to Pulmonary Fibrosis
Lung fibroblasts are thought to be differentiated from the mesoderm during embryonic development and exist in the interstitium of the lungs as resident fibroblasts [80]. However, recent findings have demonstrated other origins of lung fibroblasts [81]. In 2004, Hashimoto et al. clearly showed the existence of bone marrow-derived fibroblasts in the lungs treated with BLM in mice [82]. The typical phenotype of these cells was both CD45 and collagen positive, suggesting that the characteristics of these cells are compatible with those of so-called fibrocytes [83]. The presence of fibrocytes in the BLM model was also reported by Phillips et al., who confirmed that the trafficking of fibrocytes is dependent on the CXCL12/CXCR4 axis [84]. In the setting of IPF, patients with progressive fibrosis or acute exacerbation show increased numbers of fibrocytes in the peripheral blood [85]. However, the role of fibrocytes is currently moving to a supportive role for resident fibroblasts via the production of growth factors as a result of the direct contribution of collagen production in mice [86–89]. The significance of fibrocytes in IPF should be further examined.
The other source of these cells is believed to be EMT-derived fibroblasts. In 2005, Willis et al. reported the existence of the EMT in AECs in vitro as well as in IPF lungs [90]. According to their data, AECs expressing both pro-SP-B and α-SMA are present in cases of IPF. Kim et al. also reported the existence of EMT-derived fibroblasts expressing both pro-SP-C and α-SMA in a BLM model [91], and Tanjore found EMT-derived cells (S100A4+SP-C+) in a BLM model in mice, although the myofibroblasts expressing α-SMA were not derived from an EMT origin [92]. Subsequently, Rock et al. demonstrated no contribution of the EMT in α-SMA cells or S100A4+ cells using a confocal microscopic analysis [93]. Although these results suggest the negligible contribution of the EMT in the pathogenesis of pulmonary fibrosis, we cannot rule out the possibility that the EMT is partially generated or that the incomplete differentiation of AECs still contributes by producing several mediators, albeit not ECM.
Pleural mesothelial cells (PMCs) are reported to transform into fibroblasts in response to TGF-β in vitro [94]. In recombinant mice for GFP driven by the Wilms tumor-1 promotor, PMCs trafficking into the lungs are found to express α-SMA [95]. Although PMC-derived myofibroblasts are detected in the subpleural area of the IPF lungs, the number of these cells is reduced, indicating that PMCs are not a major source of myofibroblasts in IPF [95]. Meanwhile, endothelial cells are a major cellular component of the lungs. Hashimoto et al. reported that endothelial cells differentiate into myofibroblasts in vitro as well as in mice [96]. Although further research is required in humans, the endothelial-mesenchymal transition (EndoMT) may contribute to the onset of fibrogenesis in the case of IPF. More recently, pericytes have become a center of attention as a novel origin of lung myofibroblasts. Pericytes are known to be specialized mesenchymal cells that share a common basement membrane with endothelial cells. Using Foxd1-mapping system in genetically modified mice, Hung et al. demonstrated that 45–68 % of myofibroblasts are derived from pericytes in the lungs of BLM-treated mice [97]. Further analyses of pericytes in the IPF lungs are expected in future studies.
4.4.6 Loss of Alveolar Epithelial Integrity and Pulmonary Fibrosis
The concept of epithelial cell integrity may have an effect on the pathogenesis of IPF. Tsujino et al. found that CD151−/− mice spontaneously develop age-related pulmonary fibrosis and display increased susceptibility to BLM-induced lung fibrosis [98]. CD151 is a tetraspanin protein expressed on the basolateral surface of AECs that exhibits binding with integrin α3β1 and maintains epithelial integrity to support the adhesion of AECs to the basement membrane. In the setting of IPF, the expression of CD151 is significantly downregulated in AECs. A similar phenomenon is observed in mice deficient for phosphatase and tensin homolog deleted from chromosome 10 (Pten). Pten is a multifunctional phosphatase that negatively regulates the PI3K/Akt pathway. Miyoshi et al. showed that the knockdown of Pten in AECs induces the dysfunction of tight junctions and exacerbates lung fibrosis induced by BLM [99]. Furthermore, the phosphorylation of Akt is enhanced in the lungs of Pten-deleted mice, and the blockade of Akt by the inhibitor reduces pulmonary fibrosis. Moreover, a decreased expression of Pten and enhanced phosphorylation of Akt are observed in the lungs of IPF animals, and the AECs in Pten-deficient mice show reduced expressions of surfactant proteins [100]. However, it remains undetermined whether the loss of epithelial cell integrity is a causative factor in IPF. In contrast to that observed in CD151-deficient mice, lung fibrosis in Pten-deficient mice is not dependent on TGF-β signals. Therefore, disorders in epithelial integrity may be crucial and fundamental for the progression of lung fibrosis in the case of IPF.
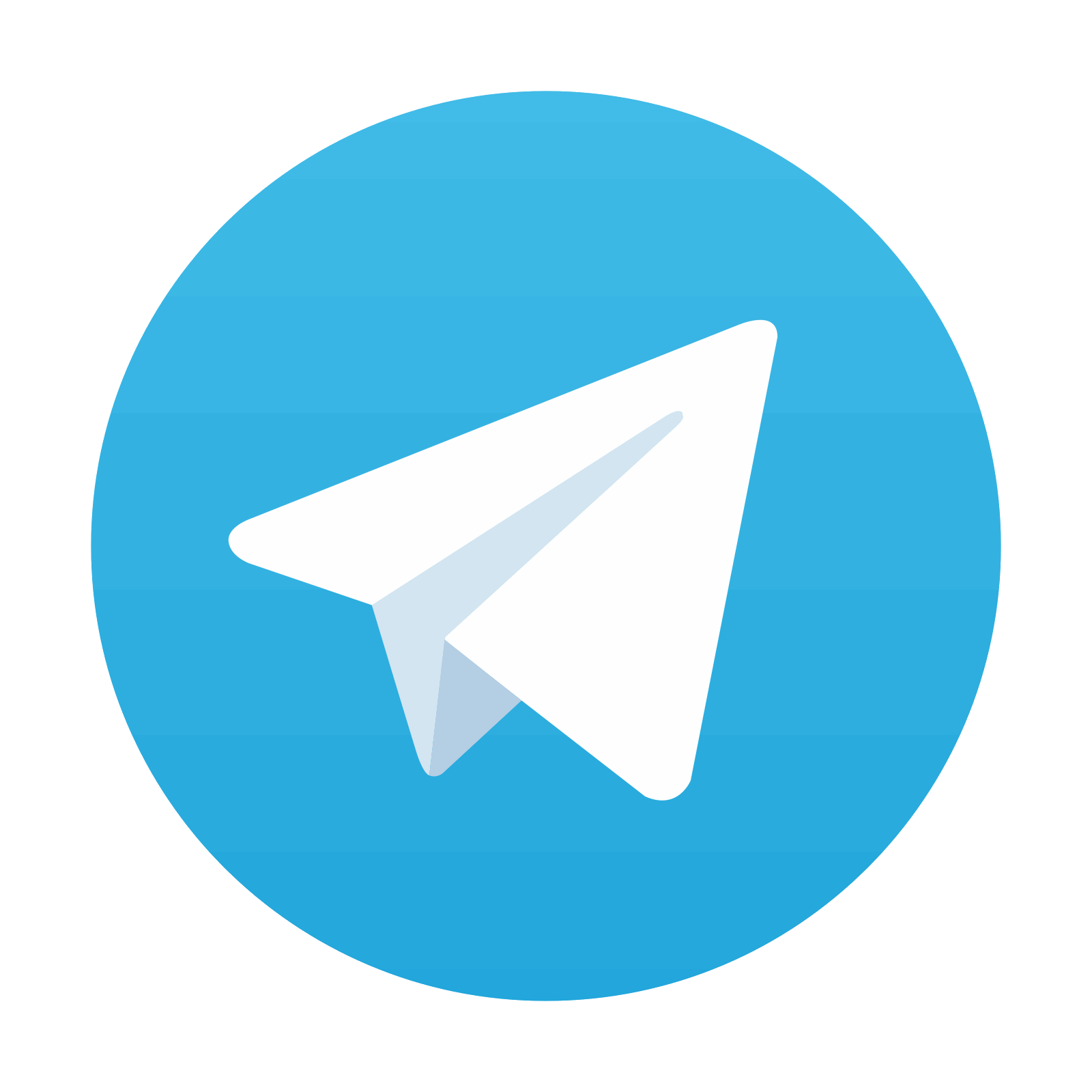
Stay updated, free articles. Join our Telegram channel

Full access? Get Clinical Tree
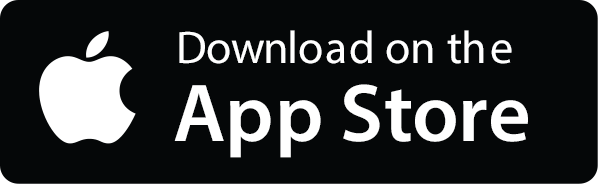
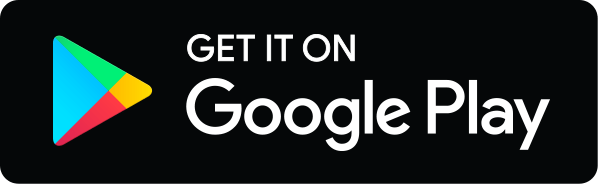