Abstract
Comprehension of the pathobiology of any disease is crucial to identifying the cellular and molecular mechanisms through which that disease arises and evolves. This may lead to the discovery of new drugs that, acting on specific targets, may be effective in slowing or stopping the disease. Idiopathic pulmonary fibrosis (IPF) is a complex disease involving exogenous risk factors, genetic predisposition, and aging. Nevertheless, substantial information about the cellular and molecular pathways involved in IPF is, at least in part, clear. By virtue of this, two different drugs—pirfenidone and nintedanib—have been developed and approved for the treatment of IPF, and a number of preclinical and clinical studies are currently under way exploring new potential drugs.
Keywords
Idiopathic pulmonary fibrosis, IPF, Nintedanib, Pathobiology, Pirfenidone, Signal transduction pathways
Key Points
- •
By virtue of basic research and new clinical observations, the amount of information and data on the pathobiology of IPF is growing day by day, offering new and more ample opportunities to either develop new therapies or reposition known drugs for IPF.
- •
A number of preclinical and clinical studies are currently under way with the aim of finding new and more potent drugs or effective cell-based treatments for IPF and possibly to personalize treatments, identifying subcategories of patients on the basis of genetic backgrounds and response to treatment.
- •
This will certainly be possible in a future not so distant, provided that the tree of knowledge for the pathobiology of IPF continues to grow florid and robust.
An understanding of the pathogenesis of any disease is crucial to identifying those cellular and molecular mechanisms through which a disease arises and evolves. This may lead to the discovery and development of new compounds and drugs that, acting on specific targets, may be effective in slowing or stopping the disease. Unfortunately, the pathogenesis of many complex diseases caused by the combination of genetic, environmental, and lifestyle factors is still not clear. Idiopathic pulmonary fibrosis (IPF) is no exception to this rule, and its pathogenesis represents the perfect example of a complex disease due to the combination of genetic predisposition and exogenous risk factors. Nevertheless, during the last few years, we have gathered useful information about some of the cellular and molecular pathways involved in the origin and progression of this dreadful disease. However, it must be admitted that some of the data collected derive either from diagnostic biopsies and explanted lung tissues or from in vitro and in vivo animal studies, and for this reason they may have some limitations.
Lung tissue from biopsy, and even more explanted tissue, mirrors disease in specific moments and very often when it is in an advanced stage, whereas in vitro and in vivo studies, although very suggestive and extremely useful, need to be confirmed in the context of the human disease. In spite of these objective limitations, there is scientific agreement in considering IPF the result of the interaction between the individual genetic background, the chronic action of different exogenous risk factors such as smoking, and the relentless action of aging. In susceptible and aged individuals, chronic damage caused by risk factors elicits an abnormal response of alveolar–bronchiolar cells that subsequently leads to the activation of fibroblasts and ultimately to tissue fibrosis. During the last 10 years some of these pathobiologic mechanisms, involved in the abnormal response to damage of epithelial cells and fibroblasts, have been disclosed, and today the overall picture of the pathogenesis of IPF seems less obscure. Based on these considerations this chapter will review some aspects of IPF pathobiology that have inspired research studies that emerged into clinical trials leading to the development of new drugs. The pathobiologic foundations of some ongoing trials will be also reviewed.
The Approach to Treatment in the Old Days
For many years lung fibrosis was considered the result of a chronic inflammatory process of the alveolar tissue that could evolve into fibrosis. Based on this belief and a long empiric experience (not supported by scientific evidence), the treatment of IPF was built for decades on high doses of steroids associated with immunosuppressants, such as azathioprine. This approach was also endorsed by the ATS/ERS statement published in 2000, although two Cochrane reviews underlined the lack of randomized controlled trials exploring the effect of steroids and immunosuppressants in IPF. Both reviews concluded that there was very little evidence supporting the role of these drugs for the treatment of IPF. This is not surprising considering that the presence of inflammatory and immune cells is not a dominant feature of IPF lung tissue. In addition, even if it is difficult to conceive, fibrosis may take place without being preceded by chronic inflammation. It has been demonstrated that it is possible to induce a fibrotic reaction in a lung culture system free of blood cells and soluble mediators. This model of “washed lung” suggested that the disruption of the normal epithelial–fibroblast interaction, induced by hyperoxia as the fibrogenic stimulus, may be sufficient, in the absence of the classical inflammatory cells, to induce epithelial damage and to promote fibroblast growth and collagen deposition, in other words, to create tissue fibrosis. This is possible because epithelial cells and fibroblasts, commonly considered structural cells, are not merely bystander cells forming the scaffold of the lung, but are instead able to produce and release mediators, to proliferate and differentiate into more active and aggressive phenotypes, and eventually to affect the behavior of the surrounding microenvironment. The turning point in defining the real nature of the pathogenesis of IPF was made by Selman in 2002; he described IPF as a noninflammatory fibroproliferative disorder triggered by an unknown stimulus responsible for alveolar epithelial cell (AEC) injury. According to this hypothesis, epithelial damage and the subsequent altered crosstalk between fibroblasts and epithelium caused the release of growth factors and cytokines involved in the recruitment and differentiation of mesenchymal cells into myofibroblasts.
In spite of the clear evidence showing a limited role for inflammation in the pathogenesis of IPF, the treatment of this disease remained based on steroids and immunosuppressants. This was likely due to at least three reasons: (1) In the recent past the diagnosis of IPF was not as accurate as today, and in some patients, with other forms of lung fibrosis such as nonspecific interstitial pneumonia (NSIP), the “traditional treatment” with steroids and immunosuppressants could be effective. (2) There is a complete lack of any other alternative treatment with the exception of lung transplantation. (3) Controlled clinical trials demonstrating whether or not this treatment was effective are lacking. This final gap was eventually filled in 2012 with the PANTHER study, a clinical trial designed to assess the efficacy and safety of triple therapy (prednisone, azathioprine, and N-acetylcysteine [NAC]) in IPF patients. Quite surprisingly, this trial was stopped before its conclusion because of an excessive number of deaths, hospitalizations, and adverse events in the treated group, and the absence of any efficacy. The PANTHER study put an end to the “traditional therapy” with steroids and immunosuppressants for the treatment of IPF.
In the meantime, additional experimental data had confirmed the marginal role of inflammation in IPF showing instead the key role of AECs and fibroblasts. According to more recent studies, the inception of tissue fibrosis is due to the exposure of AECs to chronic microinjuries, such as those caused by smoking or environmental and/or professional exposures that may damage the epithelium, causing, in susceptible individuals, the activation of incorrect repair mechanisms and stress response pathways that eventually promote fibroblast recruitment and proliferation. During normal tissue repair processes, when wound healing is over, the activity of fibroblasts ends, and they undergo apoptosis. However, in some circumstances, and IPF is one of those, fibroblast functions are altered resulting in their permanence into the tissue, ongoing deposition of extracellular matrix (ECM) proteins, parenchymal distortion, and ultimately fibrosis. Starting from this pathogenic evidence, new preclinical studies and clinical trials have been designed, shifting their focus from antiinflammatory molecules to targeting components of the wound healing cascade and fibrogenesis.
The Advent of Pirfenidone
Pirfenidone (5-methyl-1-phenyl-2-[1H]-pyridone) is an orally available synthetic pyridone compound that has been shown to inhibit the progression of fibrosis in animal models and in in vitro systems. Pirfenidone has been used for the treatment of different models of fibrotic diseases, including liver, renal, and cardiac fibrosis, all situations characterized by the abnormal deposition of collagen. The mechanism of action is still not fully understood, but preclinical studies suggest that pirfenidone combines antiinflammatory, antioxidant, and above all antifibrotic properties. Pirfenidone exerts its antiinflammatory action mainly through the regulation of tumor necrosis factor alpha and beta (TNF-α and TNF-β) pathways, whereas its antioxidant activity is exerted through the reduction of lipid peroxidation and partly because of its scavenger activity for toxic hydroxyl radicals. However, pirfenidone is best known for its antifibrotic properties.
Fibroblasts are normally quiescent cells unless tissue injury occurs. In this situation they undergo a phenotypic transition into myofibroblasts acquiring contractile and secretory properties and synthesizing collagen and ECM proteins. This is an important step in the pathogenesis of IPF, and many studies have proposed TGF-β as the key fibrogenic cytokine responsible for fibroblast differentiation. The major signaling pathway of TGF-β is through its transmembrane receptor serine/threonine kinases that in turn activate the cytoplasmic Smad proteins, and more specifically Smad3, inducing alpha smooth muscle actin (SMA) gene expression and subsequently fibroblast differentiation. It has been also demonstrated that Smad-independent molecular pathways such as phosphatidylinositol-3-kinase/protein kinase B (PI3K/Akt) might be involved in myofibroblast differentiation induced by TGF-β.
A number of in vitro and in vivo animal studies have demonstrated that pirfenidone inhibits collagen synthesis and ECM deposition and reduces fibroblast proliferation and, most important, their differentiation into myofibroblasts. Other studies have shown that pirfenidone also decreases TGF-β–induced α-SMA and procollagen (Col) I at mRNA and protein levels. These “antifibrotic” effects are likely related to the inhibition exerted by pirfenidone on the phosphorylation induced by TGF-β of Smad3, p38, and Akt, key factors for the regulation of this pathway. Other studies performed on the bleomycin model of lung fibrosis have described a diminished presence of profibrotic factors such as TGF-β in the bronchoalveolar lavage fluid (BALF) of pirfenidone-treated animals and a concurrent reduction of the accumulation of hydroxyproline, Col I, and Col III in BAL and lung tissue.
Even if the exact mechanisms through which pirfenidone acts were only partially known, the identification of its antioxidant and antiinflammatory properties along with a surprising antifibrotic activity redefined the clinical interest for this pleiotropic drug. In spite of that, it took a few years to have the first double-blind randomized clinical trial testing the efficacy of pirfenidone in IPF patients. This was a Japanese Phase II study that was stopped after only 9 months because it was considered unethical to continue the study considering the increased number of acute exacerbations in the placebo group compared with the group treated with pirfenidone. The primary endpoint of the study was not achieved—there was no difference in the saturation of peripheral oxygen under exertion between the placebo and the pirfenidone arm, but a significant reduction in the decline of vital capacity was described in the pirfenidone group. These encouraging results led to another study in Japan, whose primary endpoint was the change in vital capacity from baseline at 52 weeks. This time the study met its primary endpoint showing that the loss in vital capacity was significantly higher in the placebo group. It was also proved that pirfenidone increased the progression-free survival (PFS) defined as the time until death and 10% or more decline in vital capacity from baseline.
In the meantime two multinational randomized double-blind placebo-controlled Phase III trials (004 and 006) named CAPACITY started in Europe, Australia, and North America to test the efficacy of pirfenidone in patients affected by mild to moderate IPF. The primary endpoint, common to both studies, was defined as the change in forced vital capacity (FVC) percentage from baseline to 72 weeks. In study 004, pirfenidone significantly reduced FVC decline at week 72 but did not reduce decline in 6-min walk test (6MWT) distance, while study 006 failed to meet its primary endpoint but reduced the decline in 6MWT distance. Based on the positive results of the one CAPACITY and Japanese studies, pirfenidone was approved in 2011 by the European Medicines Agency (EMA) for the treatment of mild to moderate IPF. The Food and Drug Administration (FDA) required an additional trial to approve the use of pirfenidone in the United States; this was called the ASCEND trial. In ASCEND, pirfenidone significantly reduced the proportion of patients who experienced a decline of 10% or more in FVC and the relative risk of death or disease progression. All patients randomized to pirfenidone 2403 mg/day or placebo in the CAPACITY and ASCEND trials were included in a pooled analysis aimed to assess safety and efficacy of pirfenidone at 1 year of treatment. This study suggested that pirfenidone reduces the risk of death by 48% and improves PFS by 38% in IPF patients compared to placebo.
As already underlined in this chapter, the understanding of the pathogenic mechanisms of a disease can be important to developing new drugs. Similarly, the comprehension of the mechanisms of action of a drug is useful to better understand its potential therapeutic effect as well as to predict and possibly avoid side effects. For this reason, preclinical and clinical studies thoroughly evaluated the safety profile of pirfenidone.
To further evaluate the long-term safety and tolerability of the drug, patients who participated in five different clinical trials were analyzed: three Phase III multinational trials (CAPACITY 004, 006, and ASCEND) and the two ongoing open label studies RECAP (002, 012). This study collected data from a large and well-defined cohort of IPF patients who were treated with pirfenidone and followed for up to 9.9 years. The major adverse events were gastrointestinal (GI) events [nausea (37.6%), diarrhea (28.1%), dyspepsia (18.4%), and vomiting (15.9%)] and rash (25.0%). Elevations in blood levels of aminotransferase greater than three times the upper limit of normal occurred in 3.1% of patients. All of these events were mild to moderate in severity, transient with dose modification, and without clinical long-term consequences.
Many studies have been conducted to explain the molecular mechanism responsible for GI events. Animal studies showed that pirfenidone reduces the rate of gastric emptying and small intestinal transit, and this effect can be relieved by the administration of prokinetic drugs. It was demonstrated that the concomitant administration of mosapride and splitting pirfenidone doses during meals improve the decrease in gastric emptying rate caused by pirfenidone.
Rubino et al. studied the pharmacokinetic features of pirfenidone in a group of healthy volunteers and described the effects of the simultaneous assumption with food. The combination of pirfenidone with food caused a reduction and delay in the absorption of the drug and a reduced plasma concentration of both drug and metabolites. These results have an important clinical repercussion because a correlation between plasma concentration and the risk of adverse events has been demonstrated, suggesting that food may reduce the incidence of GI events.
On the basis of these previous studies, it is suggested that patients take the drug during or at the end of the meal, start with a longer initial dosing titration, and take a prokinetic drug if necessary to reduce the incidence of GI events. If GI events occur, it is suggested to reduce the dose and, if the adverse event persists, temporarily discontinue treatment until the symptoms become tolerable.
Photosensitivity is the second most common adverse event that may be caused by pirfenidone. A study performed on guinea pigs demonstrated that pirfenidone is photoreactive, which means that it can absorb ultraviolet B and A (UVB, UVA) radiation, causing the generation of reactive oxygen species (ROS) and lipid peroxidations that may be responsible for sunburn. After oral administration, pirfenidone distribution to the UV-exposed tissue (skin) is even higher than in lung tissue. Based on these findings, it is suggested that patients avoid sun exposure, protect skin with sunscreen active against both UVA and UVB radiation, and to wear protective clothes. Photosensitivity reaction is also related to pirfenidone dose, so that dosage adjustment or temporary discontinuation is indicated if the side effect does not resolve spontaneously. Pharmacologic treatment with oral and/or topical steroids and antihistamines is suggested in cases of persistence of the skin reaction.
On one hand, it is helpful to reduce the dose of pirfenidone to reduce the risk of adverse events, but on the other it is necessary to maintain the correct serum concentration of the drug to maximize its effectiveness. It is important to adjust the dose of pirfenidone carefully in each patient to balance tolerability with expected efficacy.
It has been estimated that 70–80% of pirfenidone is metabolized by cytochrome CYP1A2 and by CYP2C9, 2C19, 2D6, and 2E1. Thus the concomitant use of inhibitors of CYP1A2, such as grapefruit and fluvoxamine, should be avoided during the treatment with pirfenidone because they could increase the exposure to the drug and the risk of side effects. Conversely, strong inducers of CYP1A2, such as cigarette smoke, should be avoided because they may accelerate drug clearance reducing its effectiveness.
The oral administration of pirfenidone is followed by a rapid degradation of the molecule in two metabolites, 5-hydroxypirfenidone and 5-carboxypirfenidone, and their elimination in the urine. These two metabolites still have antifibrotic properties, as demonstrated by Togami et al., and their distribution in lung tissue is low if compared with the liver and kidneys because of a low affinity between metabolites and lung tissue. The direct consequence of this observation is the intriguing idea that pirfenidone and its metabolites could reach higher concentrations in the lung when administered by inhalation instead of the traditional oral route.
The Story of Nintedanib
Based on its prognosis, IPF has been generically compared to malignant disease. Similar to cancer, IPF is associated with risk factors such as smoking and/or environmental or professional exposure, and the presence of a specific genetic background is considered fundamental for the occurrence of the disease. In addition, IPF and cancer share a number of pathogenic pathways such as genetic and epigenetic alterations, abnormal expression of miRNAs, cellular and molecular aberrance including an altered response to regulatory signals, delayed apoptosis, reduced cell-to-cell communication, and above all the activation of specific signal transduction pathways. These signaling pathways are fundamental to translate any extracellular signal in an equivalent cell response and action. In this process, an extracellular signaling molecule stimulates a specific receptor inside the cell or on its surface, producing a series of intracellular events that lead, depending on the cellular type, to gene activation, metabolism alterations, or in general, changes in cell behavior.
A large variety of signal transduction pathways are activated both in cancer and in IPF, and many studies have demonstrated their involvement in the pathogenesis of these two diseases. It has been largely demonstrated that alterations of the Wnt signaling transduction pathway have clinical relevance in the pathogenesis of cancer. Indeed, the Wnt/β-catenin signaling pathway regulates the expression of molecules involved in tissue invasion such as matrilysin, laminin, and cyclin-D1, and it is also involved in a biologically relevant crosstalk with TGF-β. It is interesting to note that the Wnt/β-catenin pathway is also strongly activated in IPF lung tissue as evidenced by extensive nuclear accumulation of β-catenin at different involved sites such as bronchiolar proliferative lesions, damaged alveolar structures, and fibroblast foci. The functional significance of β-catenin has been well demonstrated by the description of an intense immunoreactivity for β-catenin and by the subsequent expression of high levels of cyclin-D1 and matrilysin. Caraci et al. have demonstrated that the Wnt/β-catenin pathway may also be activated by TGF-β through the induction of α-SMA expression via extracellular-regulated kinases (ERK)1/2 activation, glycogen synthase kinase-3beta (GSK-3beta) inhibition, and nuclear β-catenin translocation that leads to fibroblast activation and collagen production in human lung fibroblasts.
Several studies are currently exploring the anticancer effect of specific inhibitors of the Wnt pathway such as ICG-001. It is interesting that the same compound has already been used with success in an animal model of lung fibrosis. Another key process not only described in carcinogenesis but also likely involved in causing IPF is the altered regulation of apoptosis. In this case the PI3K/Akt signaling pathway plays an important role. This pathway is involved in the regulation of cell growth, proliferation, and survival. Phosphoinositol-3-kinase (PI3K) stimulates the synthesis of phosphatidylinositol-3,4,5-triphosphate, which causes the activation of Akt-modulated cellular processes such as protection from apoptosis. Based on its primary structure and in vitro lipid substrate specificity, it is possible to recognize three different classes of PI3K: classes I, II, and III. Recently, Conte et al. assessed the expression of class I PI3K p110 isoform in IPF lung tissue as well as in tissue-derived fibroblast cell lines and evaluated the effect of the selective inhibition of p110 isoforms on the proliferation and fibrogenic activity of the IPF fibroblast. The expression of PI3K p110, alpha, beta, and delta isoforms does not differ between normal and IPF tissue–derived fibroblasts, whereas immune reactivity for p110 gamma was much stronger in both IPF lung homogenates and ex vivo fibroblast cell lines. Furthermore, both p110 gamma pharmacologic inhibition and gene silencing significantly inhibited the proliferation rate as well as α-SMA expression in IPF fibroblasts. These data strongly suggest that this isoform of PI3K may have a role in the pathogenesis of IPF, representing a novel specific pharmacologic target.
In this regard, a recent study has demonstrated that oral administration of a p110 gamma inhibitor prevents bleomycin-induced pulmonary fibrosis in rats. More recently researchers have focused their attention on another signal transduction pathway activated in IPF and frequently altered in many cancers: the JAK–STAT signaling pathway. This system is a major signaling alternative to the second messenger system such as cyclic AMP, cyclic GMP, inositol triphosphate, diacylglycerol, and calcium. It transmits chemical signals from outside the cell, through the cell membrane toward the cytoplasm, and then into the nucleus. Here, it affects the activity of gene promoter regions, causing DNA transcription and cell activation. One of the regulatory mechanisms of the JAK-STAT signaling pathway is represented by the SOCS family (suppressor of cytokine signaling proteins). Bao et al. have demonstrated that in IPF patients there is a lower expression of SOCS1 and this finding has been related to more severe manifestations of the disease and to a worse prognosis.
One of the most studied signaling pathways, strongly activated not only in many cancers, but also in IPF, is the tyrosine kinase pathway. Tyrosine kinase is an enzyme that acts as a sort of “on” or “off” switch that regulates many cellular functions. Mutations can turn tyrosine kinases in a nonstop functional state that, under specific circumstances, may lead to the initiation or progression of cancer. More recently this pathway has also been studied in the context of the wound healing process and fibrosis. Indeed, tyrosine kinases catalyze the phosphorylation of tyrosine residues in proteins such as platelet-derived growth factor (PDGF), fibroblast growth factor (FGF), or vascular endothelial growth factor (VEGF), regulating a wide array of cellular functions, including cell growth, differentiation, adhesion, motility, and regulation of cell death.
PDGF, a ligand of tyrosine kinase receptor, is a heterodimeric molecule expressed in different cells, including fibroblasts. Binding of PDGF to its receptor (PDGFr) leads to autophosphorylation of the receptor and activates intracellular pathways, such as Ras, Raf, and MEK, and extracellular ones such as ERK and PI3K. PDGF is a potent growth factor for fibroblasts in vitro, and some mediators such as TGF-β and basic FGF have PDGF-dependent profibrotic activities. PDGF is more highly expressed in epithelial cells and alveolar macrophages in the lungs of patients with IPF. Moreover, high levels of PDGF have been shown in irradiated mice, and the use of a PDGFr inhibitor has attenuated the development of pulmonary fibrosis in animal models induced by radiation.
In addition to PDGF, FGF is involved not only in carcinogenesis but also in fibrogenesis. FGF receptors (FGFrs) are present on epithelial cells and fibroblasts and mediate epithelial–mesenchymal transition and fibroblast transition into myofibroblasts. FGF and more specifically two members of this family of growth factors such as FGF1 and FGF2 are potent activators of fibroblast proliferation and collagen production. The binding of FGF to FGFr causes autophosphorylation and activation of downstream signaling via PI3K/Akt, ERK1/2, and Ras/Raf/MAPK pathways. Interestingly, TGF-β regulates the FGFr cascade, inducing the upregulation of FGFr-1 and the release of FGF2 in human lung fibroblasts. In animal models of pulmonary fibrosis FGFr-1 is strongly upregulated, whereas it is not expressed in control animals, suggesting its involvement in the fibrotic process. This is substantiated by the observation that inhibition of FGF signaling ameliorates bleomycin-induced pulmonary fibrosis. In addition, increased levels of FGF have been detected in lung cells of patients affected by IPF.
If the role of VEGF in cancer is well recognized, its involvement in IPF is less clear. VEGF is produced by alveolar and bronchial epithelial cells, airway smooth muscle cells, fibroblasts, and endothelial cells. VEGFr is characterized by the typical intracellular domain that undergoes autophosphorylation as a consequence of the binding VEGF-VEGFr, activating different signaling pathways (p38, PI3K, Ras) involved in cell proliferation and migration. In IPF patients, VEGF expression is low in fibroblasts and leukocytes in fibrotic lesions, but increased in endothelial cells and AECs. Parkas et al. reported direct effects of VEGF-A in lung fibroblasts, enhancing collagen I expression induced by β. More recently, high VEGF serum concentration in IPF patients was related to a worse 5-year survival rate. The precise role of VEGF-VEGFr signaling in IPF is controversial, and further studies are required to clarify its exact role. Nevertheless, VEGF and even more PDGF and FGF have a potent effect on fibroblast activation and proliferation, and their inhibition is expected to reduce fibrosis in IPF.
Based on the previous discussion, tyrosine kinases receptor inhibitors, widely used in non–small cell lung carcinoma and other cancers, have been tested for the treatment of IPF. Imatinib mesylate, a specific inhibitor of PDGFr, was evaluated for its potential antifibrotic effects in preclinical and clinical studies. It was able to inhibit fibroblast proliferation and collagen deposition in vitro and in vivo, but when it was evaluated in a clinical trial, no benefit in slowing disease progression was found. To achieve a more potent antifibrotic effect, other compounds able to exert an inhibition of multiple receptors were tested. BIBF 1000, an inhibitor of PDGFr, VEGFr, and FGFr, was first evaluated in a mice model of bleomycin-induced pulmonary fibrosis and in an ex vivo fibroblast differentiation assay. It was found to attenuate fibrosis by reducing the expression of profibrotic factors and by decreasing collagen deposition.
Along the same lines, BIBF1120 (nintedanib), a triple kinase inhibitor, with potent suppressing effects on VEGFr, PDGFr, and FGFr, was also evaluated. Nintedanib exerts its inhibitory effect on PDGF, FGF, and VEGF through the occupation of the intracellular ATP-binding pocket of the tyrosine kinases. This drug interferes with fibroblast proliferation as well as with the secretion and deposition of ECM. It has been shown in a mice model that nintedanib reduces TIMP1 and TIMP2, which in turn inhibit matrix metalloproteinases responsible for the degradation of collagen type I and II. It also reduces collagen deposition and inhibits the transformation of fibroblasts into myofibroblasts in human lung fibroblasts of IPF patients by TGF-β.
More recently, nintedanib was studied in clinical trials as a potential antifibrotic therapy in IPF, demonstrating that treatment with this drug may reduce the decline in lung function of IPF patients by about 50%. Based on these results, nintedanib has been approved as a new therapy in patients with IPF. This is relevant, because for the first time, based on the observation of the pathogenic similarities between IPF and cancer, a drug “borrowed” from another field, in this case oncology, has been “repositioned” for IPF. “Drug repositioning” is an interesting and convenient strategy that may save time and reduce costs in the development of a specific drug. The safety profile of the explored drug is already known so that the risk of adverse events is reduced. In addition, the mechanism of action of a repositioned drug is known and can be used in those diseases where a similar mechanism is involved. Today, computational strategies are available to explore the mechanisms of action of approved drugs and to match them with cellular and/or molecular targets involved in the pathogenesis of virtually any disease.
The first randomized, double-blind, placebo-controlled, Phase II trial conducted to evaluate the efficacy of nintedanib in IPF patients (TOMORROW study) demonstrated, in the group receiving BIBF1120, a reduction of 68.4% in the annual rate of decline in FVC and a lower incidence of acute exacerbations compared with placebo. Following the results of this clinical trial, two other randomized double-blind Phase III trials (INPULSIS-1, INPULSIS-2) were performed. A total of 1066 IPF patients were randomized in a 3:2 ratio to receive placebo or BIBF1120. This treatment reduced the rate of decline in FVC over the 52-week study period in both INPULSIS trials. The secondary endpoints were represented by the time to the first exacerbation and the change in total St. George’s Respiratory Questionnaire (SGRQ) score, but no consistent differences were found between the placebo and the nintedanib groups. Recently, pooled analyses of data from the three trials (TOMORROW, INPULSIS 1 and 2) were performed to obtain an overall estimate of the clinical efficacy of nintedanib in 1231 IPF patients. Nintedanib consistently slowed disease progression, reducing the annual rate decline in FVC compared with the placebo group and causing a modest change in SGRQ total score from the baseline. This analysis also demonstrated a positive trend toward a reduction in risk for all-cause and respiratory mortality.
Nintedanib, after its oral administration, is rapidly metabolized by hepatocytes into metabolite BIBF1202. This metabolite is eliminated through the liver and feces, while the excretion in the urine is minimal. The hepatic metabolism of nintedanib is CYP450 independent; therefore, the drug interactions are very limited if compared with pirfenidone. Particular care should be used in patients affected by coagulopathy at risk of bleeding because nintedanib, as an inhibitor of VEGF, can theoretically cause bleeding. The most frequent adverse events recorded during the studies were GI events, especially diarrhea, which occurred in 61.5% of patients in INPULSIS-1 and 63.2% in INPULSIS-2, and increased liver enzymes levels, which occurred in 4.9% of patients in INPULSIS-1 and 5.2% of patients in INPULSIS-2, respectively. In the pooled data analysis, diarrhea was the most frequent adverse event in the nintedanib group (61.5% of patients treated with nintedanib vs. 17.9% of patients treated with placebo). In general, adverse events are reversible with reducing the dose of nintedanib or stopping the treatment, without clinically significant consequences.
Diarrhea was described as the most frequent adverse event following nintedanib assumption. The pathophysiologic mechanism of drug-induced diarrhea is still not completely understood, but some studies conducted for oncologic tyrosine kinase inhibitors could be helpful to understand the molecular mechanisms through which diarrhea develops. It has been supposed that patients treated with tyrosine kinase inhibitors that are directed against EGFR experience diarrhea because of the excessive chloride secretion and deficient sodium absorption. In the normal colon, sodium absorption and chloride secretion are stimulated directly by intracellular messengers such as c-AMP and intracellular calcium. EGFR is a negative regulator of chloride secretion and is expressed in GI normal mucosa. EGFR inhibitors could increase chloride secretion, inducing a secretory diarrhea. Diarrhea following imatinib has also been related to c-Kit inhibition, highly expressed in the interstitial cells of Cajal, which have a pacemaker function in the intestine, so that c-Kit inhibition can cause an alteration in the intestinal motility resulting in diarrhea.
Nintedanib dose reduction rapidly lowers the incidence and severity of diarrhea. Sometimes the addition of loperamide is indicated as a useful treatment to decrease intestinal motility.
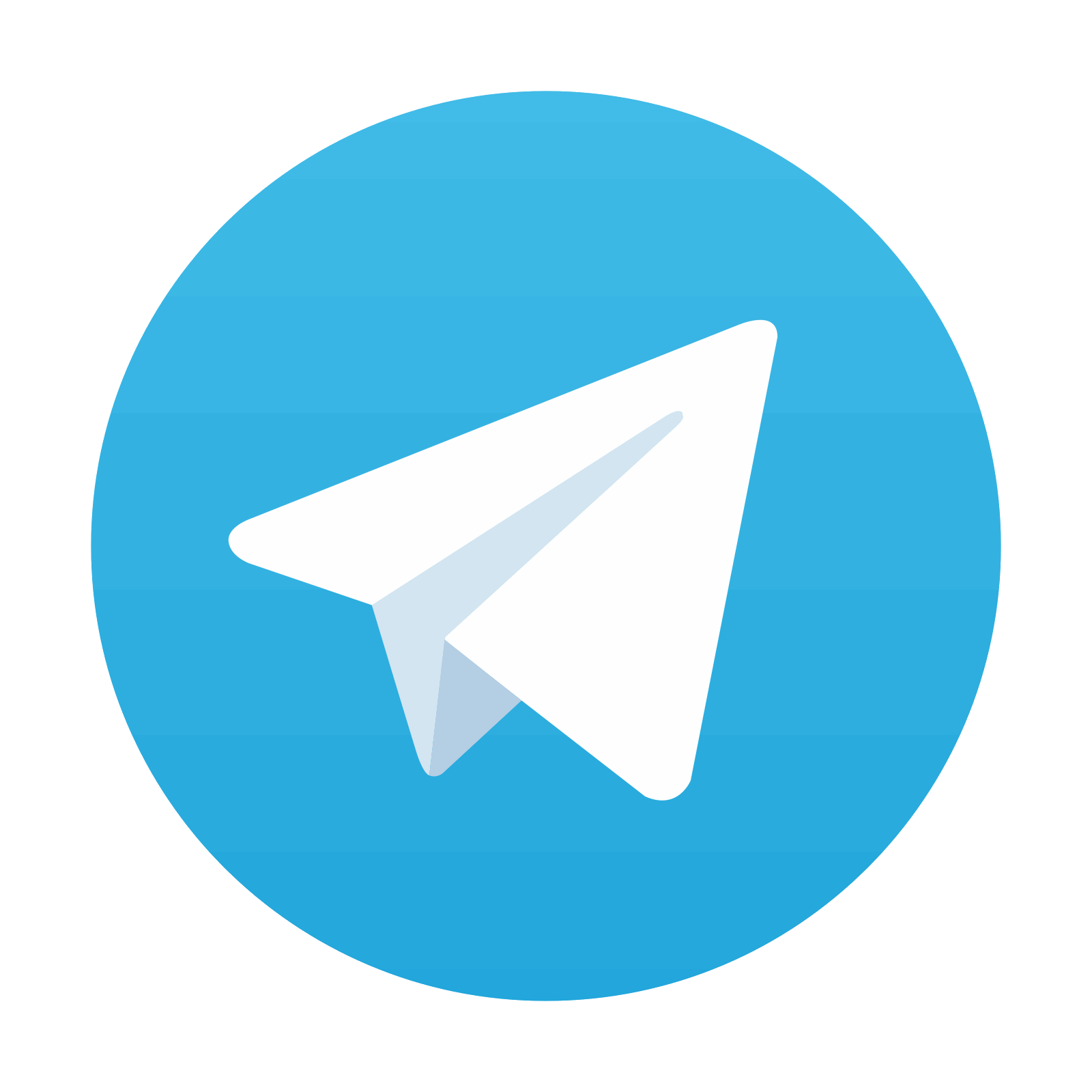
Stay updated, free articles. Join our Telegram channel

Full access? Get Clinical Tree
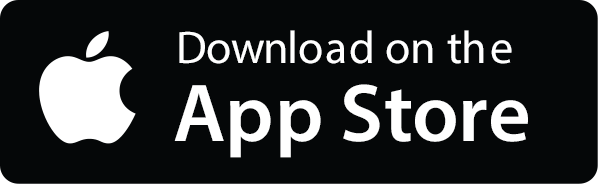
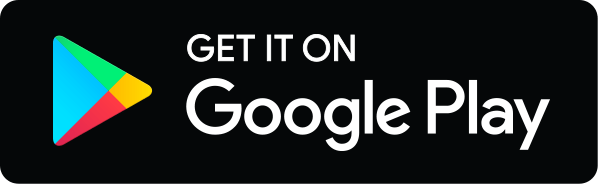