© Springer International Publishing Switzerland 2017
Mert Şentürk and Mukadder Orhan Sungur (eds.)Postoperative Care in Thoracic Surgery10.1007/978-3-319-19908-5_1717. Pain Management Following Thoracic Surgery
(1)
Department of Anesthesiology and Intensive Care Medicine, Istanbul University, Istanbul Faculty of Medicine, Istanbul, Turkey
17.1 Introduction
There are numerous articles with the keywords “pain after thoracotomy” or “postthoracotomy analgesia” emphasizing the fact that this is one of most attractive and challenging topics of anesthesiology even after decades and thousands of studies.
Several reasons contribute to this “ongoing challenge”:
- 1.
Thoracotomies (with posterolateral and posterior incisions) are one of the most painful operations. Physiopathology of postthoracotomy pain is very complicated and still not totally explained.
- 2.
The main target organ of the complications of postoperative pain and the operation is the same: the lung. Therefore, there is a strong relationship between appropriate postoperative pain therapy and pulmonary complications like atelectasis and pneumonia [1].
- 3.
Pain following thoracic surgery is triggered by breathing cycle constantly and exacerbated by movements such as coughing or deep breathing which can also determine efficacy of analgesic therapy [2]. Although a patient can sleep without pain (i.e., “low VAS-rest”), optimal pain therapy targets analgesia of a patient who can cough effectively (i.e., “low VAS-cough”). However, achieving such a target with high doses of opioids can also worsen the postoperative respiratory functions.
- 4.
17.2 Physiology of Pain
Before discussing management of pain, a thorough understanding of pain physiology and surgical trauma is a must. Acute pain after thoracotomy can be due to direct or indirect trauma. Surgical dissection of tissues including skin, muscles, ribs, pulmonary parenchyma, pleura, and nerves (acute intercostal neuralgia) constitutes direct trauma. Stretching of ligaments (acute costochondritis, posterior costochondral ligament damage, costochondral dislocation), pressure exerted by rib retractors, and irritation or inflammation as a result of surgery can cause indirect trauma [5, 6]. Trauma can continue even after surgery due to drainage tubes, residual blood, sutures, or wires adjacent to neurovascular bundle [7].
Nociceptive stimuli following trauma are transmitted to the central nervous system via intercostal, thoracodorsal, long thoracic, vagus, or phrenic nerves.
Intercostal nerves usually carry somatic nociceptive stimuli to the dorsal horn of the spinal cord by both fast-conducting, myelinated A delta and slower, unmyelinated C-fibers [8].
Thoracodorsal and long thoracic nerves which arise from C5–C7 roots carry nociceptive stimuli due to injury of latissimus dorsi and serratus anterior muscles [9].
Surgical manipulation of the pleura/pericardium, diaphragm, or bronchi can also result in a visceral stimuli transmitted by vagus and phrenic nerves. Diaphragmatic irritation results in phrenic nerve stimulation that is usually referred to ipsilateral shoulder pain (also known as postthoracotomy shoulder pain, PTSP) [10]. Musculoskeletal components due to distraction of posterior thoracic ligaments and brachial plexus stretching [11] also contribute to PTSP development.
Chronic postthoracotomy pain syndrome (CPTP) is defined as “pain that recurs or persists along a thoracotomy scar at least 2 months following the surgical procedure” [4] and is thought to have neuropathic, myofascial, and visceral components. CPTP is strongly related with acute postoperative pain [12]. Other risk factors identified for CPTP besides acute neuropathic pain are female gender, radiation therapy, presence of preoperative pain, and extensive surgical trauma including pleurectomy.
Surgical technique plays an important role in both acute and chronic pain. Thoracotomy for open lung resection usually involves a posterolateral incision below the scapula tip (mostly at fifth intercostal space) and dissection of latissimus dorsi and serratus anterior muscles [13]. Limiting incision size, appropriate closing of the muscles, or avoiding splitting the latissimus dorsi can decrease surgical trauma. In recent years, surgery is modified to completely or partially preserve muscles (also called muscle-sparing thoracotomy), though their effectiveness in reducing postoperative pain is controversial [14–16]. Anterolateral incision in which surgical exposure may be limited causes less pain than posterolateral approach [17]. Acute pain due to classical techniques is more severe than thoracoscopic procedures (i.e., video-assisted thoracoscopic surgery or VATS). However, chronic pain can still be encountered in VATS (albeit at a lower incidence) [18, 19] possibly due to trocar insertion trauma [20].
17.3 Consequences of Untreated Pain
Main clinical consequence of inadequate pain relief in thoracotomy patients is altered pulmonary mechanics. Postoperative pulmonary dysfunction in thoracotomy patients is a common result of a combination of multiple factors such as preexisting lung disease, loss of parenchyma due to surgery, positioning, single-lung ventilation, and pain [21]. Surgery is known to decrease vital capacity as far as 15 % in lobectomy and 35–40 % in pneumonectomy [22]. Furthermore, general anesthesia itself may cause a functional residual capacity (FRC) reduction up to 20 % [23], and this is increased in lateral position [24]. Lastly, the patient needs to breathe to avoid postoperative deeply pulmonary complications. Thoracotomy patients often avoid deep breathing as it causes further stretching of incision, and instead “splinting,” i.e., expiratory muscle contraction, is observed. Thus, uncontrolled postoperative pain results in decreased lung compliance, reduced functional residual capacity, ventilation/perfusion mismatch, splinting, atelectasis, hypoventilation, hypoxia, and hypercarbia [25].
Acute pain can also cause an increase in sympathetic flow, heart rate, preload, and postload resulting in increased myocardial oxygen consumption which may be deleterious in a patient with ischemic heart disease. For endocrine consequences, pain is associated with catecholamine, ACTH, aldosterone, cortisol, ADH, angiotensin, and glucagon increase which produces a catabolic state with hyperglycemia and free water retention. Other repercussions of untreated acute pain can be listed as altered coagulation, fibrinolysis, cytokine production, and gastrointestinal motility and CPTP.
17.4 Treatment of Pain
A wide variety of techniques and analgesics can each target different points in acute pain transmission. In the past, most authors agreed that optimal strategy should be preemptive and multimodal, and hence a preoperatively placed thoracic epidural catheter should be a standard in all patients undergoing major open thoracic surgical procedures as stated by Gottschalk et al. [20]. In less than 10 years, all three standards (preemptive, multimodal, and thoracic epidural) in this review, though still relevant, became somehow controversial.
17.5 Multimodal Analgesia
Postthoracotomy pain has complex mechanisms involving both incisional (nociceptive) pain due to the damage of myofascial structures and neuropathic component in transition from acute to chronic pain. To expect one method of analgesia to be omnipotent is to set oneself for failure. For example, as explained above, PTSP accompanying thoracic procedures is caused by afferent impulses conducted with phrenic nerves and can be treated with a phrenic nerve blockade [26], but not with thoracic epidural or paravertebral analgesia. To cover all the components and the whole pathway of thoracotomy pain, using systemic application of opioids would require very high doses with side effects and/or remain inadequate. Therefore, systemic use of opioids is not a first-choice treatment and should be considered at most as a “rescue” analgesic of other methods.
Combining strategies acting on different sites of central and peripheral nervous system is attractive as side effects of high-dose opioids can be avoided with better analgesia. A commonly used strategy is to combine local anesthetics (regional analgesia or infiltration), opioids, and non-opioid analgesics with regional anesthesia as mainstay of pain relief. This strategy theoretically may also aid in decreasing humoral inflammatory factors in circulation and hence decrease central sensitization. Role of multimodal analgesia in prevention of transition from acute to chronic pain is yet unknown [5].
17.6 Preemptive Analgesia
“Preemptive analgesia” concept, i.e., providing analgesia to prevent the establishment of central sensitization caused by incisional injury – before the noxious stimulus – had been initially advocated by experimental studies. However, the promising results of experimental studies could not be confirmed by following clinical studies [27]. Probably, this controversy was due to an incorrect definition of the concept. As a matter of fact, it is not rational to expect that only a preoperative peri-incisional injection of lidocaine would cause a change in postthoracotomy pain [28]. In a new definition, “preventive analgesia” should prevent the establishment of central sensitization caused not only by incisional but also by inflammatory injuries, covering the whole preoperative and early postoperative periods [29]. This should lead to an equal effective analgesia with lower doses of analgesics. In a prospective randomized trial comparing the effects of preoperative- or postoperative-initiated thoracic epidural anesthesia (TEA) versus intravenous opioids, it has been found that the preoperative initiation of TEA was associated with a significant improvement in both acute and chronic postthoracotomy pain [3]. A meta-analysis on timing of analgesia for postthoracotomy pain also showed that preoperative thoracic epidural analgesia is associated with a better control of acute pain [30].
17.7 Regional Analgesia
17.7.1 Thoracic Epidural Analgesia/Anesthesia
Thoracic epidural analgesia (TEA) has been traditionally regarded to be the gold standard in the treatment of postthoracotomy pain [31, 32]. TEA provides better analgesia, better quality of life [33, 34], and better preservation of FRC [34] compared to parenteral opioids. TEA is associated with a significant decrease in postoperative pulmonary complications such as pneumonia and atelectasis [35, 36]. There is also evidence that TEA may prevent chronic postthoracotomy pain [3] and provide beneficial anti-ischemic [37] and anti-arrhythmic effects [38].
One should also be aware of possible complications of epidural analgesia such as failure to place the catheter, hypotension due to bilateral sympathetic blockade, urinary retention, nausea, and rarely nerve damage, hematoma, infection, and accidental intrathecal or intravascular spread with resulting local anesthetic toxicity [39]. Therefore successful implementation of TEA depends on provider’s attitude and conduct as with any anesthetic procedure. The concept is linked to several questions concerning:
17.7.1.1 How to Do It?
As thoracic epidural catheterization requires a manipulation above the conus medullaris (usually at T3–T9 level), there is possibility of medulla spinalis injury. This procedure, combined with the fact that thoracic epidural catheter is considered to be technically more difficult than the lumbar one, should be performed “awake” (or “lightly sedated”) to warn the anesthetist of any possible neurological injury. Placement of thoracic epidural catheters in anesthetized patients has resulted in serious neurological damage [40]. Another advantage of placing epidural catheters prior to anesthesia would be testing of sensory block extension.
Epidural catheter is often advised to place between T3–T6 levels via a paramedian approach. There are two main reasons for such a recommendation: one is the extreme upward angulation of the processus spinosus at the mid-thoracic region, and the other is possible ligamentum flavum midline gaps in cervical and thoracic regions. With the same rationale, it is also recommended to use “hanging drop” technique instead of “loss of resistance.” However, the authors’ experience is that the more common approach of “loss of resistance” via the median approach is still appropriate (and easy to perform) especially in low thoracic levels. Furthermore needle for skin local anesthetic infiltration can also be used as a “relatively noninvasive” guide to locate optimum insertion angle.
The line connecting the inferior angles of the scapula is the landmark of T8; however this landmark should also be checked by counting up from the iliac crest specifically in obese patients [41]. Of note, the catheter and solution delivered should target dermatomes where nociceptive input originates to provide better analgesia with minimal side effects (right place, right drug, and right dose). Considering that the catheter would be inserted some 2–4 cm within the epidural space, the optimal level for best epidural drug spread can be adjusted. After high-thoracic epidurals, spread occurs markedly more caudal than cranial. Conversely, low-thoracic epidurals have more cranial spread, while at the mid-thoracic level, there is a homogenous spread in both directions [42].
17.7.1.2 Should It Be Mid-thoracic, or Is Low Thoracic or Lumbar Also Possible?
The “congruence” of the catheter and the incision appears to be crucial. Placing a catheter that does not coincidence with incision can result in lower pain relief [43] and possible early removal of the epidural catheter because of ineffective analgesia. Moreover, the advantages associated with the attenuation of the stress response because of sympathetic blockade have been shown to be effective only with extensive blockade [44]. On the other hand, many anesthetists (especially the ones working in low-volume centers for thoracotomies and hence less experience with TEA) tend to prefer lumbar epidural analgesia (LEA). This approach can be advocated with a less possibility of neurologic injury and may be performed also after anesthesia induction. However the success of such approach depends on the use of opioids, specifically hydrophilic opioid morphine which tends to spread to thoracic regions [45, 46]. Similarly, single-shot intrathecal morphine may provide adequate pain relief for 12–24 h [47] with doses ranging from 15 to 20 μg/kg [48].
17.7.1.3 What and How Much to Inject?
A combination of local anesthetic and opioids has apparent benefits over the solo use of both drugs. The combination makes it possible to decrease the doses of both drugs, leading to a decreased frequency and intensity of unwarranted effects (e.g., less pruritus because of opioids and less motor blockade because of local anesthetics). Furthermore local anesthetics have been shown to facilitate the entry of opioid from the epidural space into the cerebrospinal fluid [49]. The only possible drawback of adding opioid is the possibility of a late onset of respiratory depression, but this can be minimized by using appropriate doses of appropriate opioids.
Regarding local anesthetics, bupivacaine, levobupivacaine, and ropivacaine are popular choices in differing concentrations. Application of the same amount (=dose) of the epidural cocktail in different concentrations/volumes mostly depends on “individual” choices. Although a case can be made to avoid “intense” analgesia (even “anesthesia”) in a limited region for high concentration in low-volume solutions in the postoperative period, this has not shown clinically [50]. Regarding the choice of the opioid, epidural lipophilic opioids such as fentanyl or sufentanil prefer to stay in epidural fat and have low spinal bioavailability resulting in rather “narrow” but rapid-onset analgesia, whereas hydrophilic opioids such as morphine can reach cerebrospinal fluid in higher ratio and can achieve a “wider” analgesia despite increased nausea/vomiting and late onset of action. The optimal concentration of epidural fentanyl for bupivacaine 0.1 % was found to be 5 μg/ml [51]. Our center also uses patient-controlled epidural analgesia with bupivacaine 0.1 % and morphine 0.05–0.1 mg/mL solution successfully [52].
Several adjuvants have been studied regarding their effectiveness in TEA. Among them, magnesium [53], ketamine [54], clonidine [55], dexmedetomidine [56], and neostigmine [57] appear to be promising. They all are reported to be associated with a reduction in postoperative analgesic requirement; the effects on chronic pain are rather controversial. Unfortunately, almost all of these drugs are to be used “off-label” via the epidural route; this is maybe the most important reason why their use is limited to scientific trials.
17.7.1.4 When to Start?
As explained above, preoperative insertion and dosing of epidural catheter and onset of analgesia prior to incision while continuing analgesics in the postoperative 48–72 h can provide “preventive analgesia.” In our institution, a loading dose of 10 mL of aforementioned epidural solution is applied before the surgery, and a constant infusion rate of 7–10 mL/h is continued throughout the surgery. However, one should be aware of possibility of hypotension at the start of surgery and be ready to counter this either by decreasing the general anesthetic levels with the depth of anesthesia monitoring or if necessary low doses of vasopressors. Obviously, loading the patient with fluids should be avoided in these cases, as long as the reason of hypotension is not hypovolemia. Second concern to preoperative or intraoperative use of TEA is that it may lead to pulmonary vasodilation. It can be assumed that the hypoxic pulmonary vasoconstriction would be inhibited, with a consequent increase in pulmonary shunt and decrease in oxygenation. Although there are some studies supporting this assumption, the increase in shunt and decrease in oxygenation appear to be statistically insignificant and clinically irrelevant with both isoflurane and propofol [52]. During the postoperative period, PCEA protocol with no loading dose, basal infusion of 5 mL/h, 3 mL bolus dose, and 30-min lockout period is used in our institution. However, this regimen is also tailored in each patient individually.
17.7.2 Paravertebral Block
Paravertebral block (PVB) with its fast-growing popularity is now considered as a serious and in some cases an even more appropriate alternative of TEA [58]. Unnecessary bilateral sympathetic blockade resulting in hypotension and urinary retention encountered in TEA can be avoided with PVB, and possibility of neurological injury, hematoma, epidural abscess, accidental intrathecal injection, and systemic local anesthetic injection, although theoretically possible, is lower in PVB than TEA. Specific complications listed for PVB include ipsilateral Horner syndrome and pneumothorax. As with TEA, infection at injection site, severe coagulopathy, and deformities of the spine resulting in technical difficulty to perform the block are the main limitations to the use of PVB. Furthermore, PVB should not be performed in patients depending on intercostal muscles for ventilation or in patients with ipsilateral diaphragmatic paresis.
In terms of outcomes, although its effect on preventing chronic postthoracotomy pain is unknown, recent reviews have stated that PVB is a safe and superior alternative to TEA for dynamic analgesia [59] and has comparable efficacy to TEA in static analgesia [60]. PVB is also successful in preserving pulmonary function and preventing complications such as failure of technique, hypotension, need for vasopressors, urinary retention, nausea and vomiting, need for ventilator support, need for reoperation, arrhythmia, anastomosis leak, and sepsis [5, 11]. Of note, most studies reporting better or comparable analgesia with TEA have been placed by the surgeon under direct vision. Also one should keep in mind that its efficacy would be limited following single-shot injection. Furthermore, according to a systematic review and metaregression study by Kotze et al., the effectiveness of PVB is increased with continuous infusion techniques when compared to intermittent boli [61]. This study also reported that higher doses of bupivacaine provided better analgesia when compared to lower doses. When using high doses, albeit infrequently, local anesthetic toxicity is possible especially if the pleural integrity is not preserved as absorption of the local anesthetic is faster from the pleural space compared to the paravertebral space [62].
The anatomy of the thoracic paravertebral space plays an important role in the application of PVB. This is a wedge-shaped space bounded medially by the bodies of the vertebrae, intervertebral discs, and intervertebral foramina, anterolaterally by the parietal pleura and the innermost intercostal membrane, and posteriorly by the transverse processes of the thoracic vertebrae, heads of the ribs, and the superior costotransverse ligament.
In the classical technique, needle is inserted 25 mm lateral to the spinous processes of the targeted vertebrae and directed posteroanterior till pars intervertebralis, articular column, or transverse process is encountered. Once the bone is met, needle is advanced in an inferior (caudal) and lateral direction while testing for a change in resistance. Change in resistance indicates penetration of the costotransverse ligament and identification of paravertebral space. We will not explain the technique of PVB in details, but one important point is that there should be a feeling of “change in resistance” as the needle is advanced caudally beyond the costotransverse ligament and needle insertion depth should be predefined (i.e., no more than 10–15 mm) [63]. This means a “click” and a “loss of resistance” similar to the ones in TEA should be avoided, for they may indicate a pleural puncture. Another important point to remember is cranial advancement can increase the risk of pleural puncture, whereas medial angulation can cause epidural, intrathecal, or spinal injection.
Although technically easy, an unacceptable high rate of misplacement is shown with classical landmark technique radiologically [64]. The advance of ultrasound technology has enabled us to visualize the transverse process, costotransverse ligament, and paravertebral space and pleura. The use of ultrasound, albeit time costly, can reduce misplacement and/or complications either with assistance as distance to paravertebral or pleural space can be measured prior to block or with direct needle visualization and guidance in real time during the block [65, 66]. Furthermore, anterior displacement of parietal pleura with injection of local anesthetic can be observed.
Interestingly, in magnetic resonance imaging studies, a discrepancy can be observed between spread of local anesthetic and somatic analgesia (i.e., although the injected local anesthetic only encompassed four vertebral levels, sensory block levels were more extensive and showed high variability) which is explained by some via a possible secondary epidural spread [67].
17.7.3 Intercostal and Interpleural Block
For intercostal block, each intercostal nerve is targeted at inferior rib margin in intercostal space. In these blocks, local anesthetic spread is mostly distal, and proximal spread is relatively limited unlike PVB requiring multiple injections and high amount of local anesthetics with possible systemic toxicity side effects for effective analgesia. However, these blocks provide fast, easy, and valuable alternative in patients when TEA and PVB cannot be used. Although intercostal nerve blocks have been shown to be effective especially in multiple injections and continuous infusions in a meta-analysis [31], this has not been the case for single-shot block [68]. Yet, there is recent interest in these blocks with the use of long-acting bupivacaine liposome [69].
Interpleural analgesia targets the spread of local anesthetic from interpleural space in a retrograde manner to intercostal and paravertebral space. However, this technique is not recommended due to possibility of air entrapment (pneumothorax) during needle pass through the pleura, large doses of local anesthetic requirement (systemic toxicity), and loss of drug via chest tubes [31].
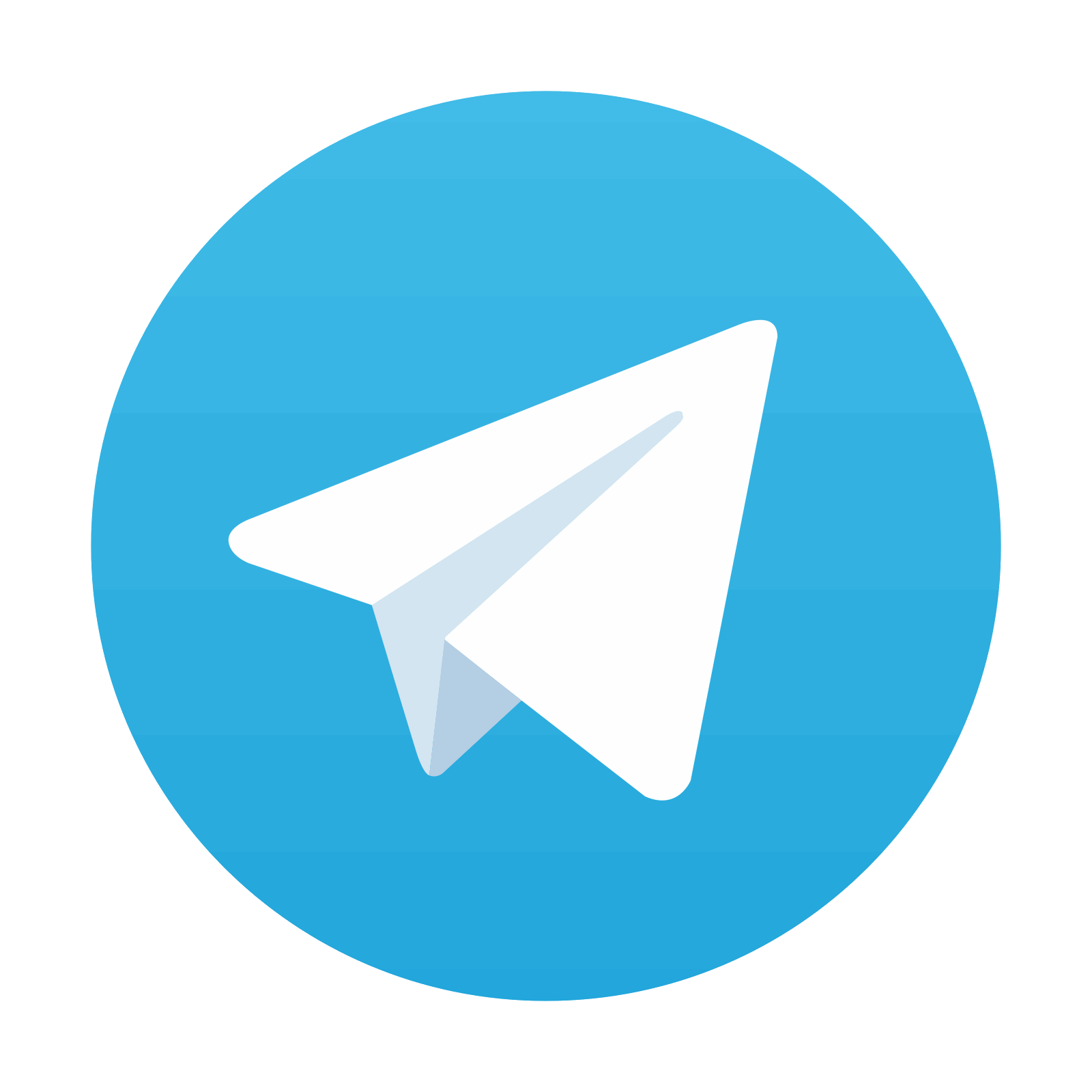
Stay updated, free articles. Join our Telegram channel

Full access? Get Clinical Tree
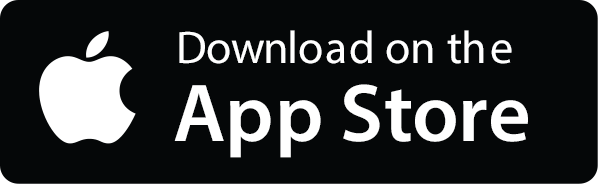
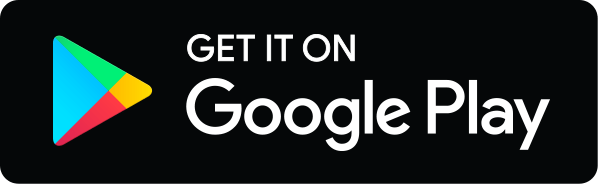