The diaphragm is generally referred to as the main “respiratory muscle”—which is to some extent a misnomer. A better description would be that the diaphragm is the primary muscle of ventilation. Its critical physiological role is to serve as the main muscle which moves air into the lungs, where this air, of course, oxygenates the blood. Since delivery of oxygenated blood to all parts of the body is critical to life, and since intact cardiac and respiratory systems are the essential elements to assure that this oxygenated blood is manufactured and delivered, failure of any of the components that makes up either one of these systems results in death or severe disability. One could thus make a very strong argument that along with the heart and lungs, the diaphragm is one of the three most important organs in the body.
Just as the heart is the blood pump, the diaphragm is the air pump. Brief consideration will convince the reader that the heart and diaphragm are the only two muscles in the body that are continuously active throughout the life of an individual. If either one of these muscles fails, the individual fails. As the only skeletal muscle that is continuously active (the heart is made up of cardiac muscle), the diaphragm presents a number of interesting physiological adaptations. Diaphragm muscle fibers demonstrate unique characteristics that permit it to function adequately given this unusual role of constant activity. Further, its special role seems to influence the diaphragm muscle’s response to various disease states (e.g., emphysema) and medical interventions (e.g., mechanical ventilation [MV]), such that it often does not respond in the same manner as skeletal muscles in the limbs do under similar circumstances.
Beyond its physiological role, the diaphragm also serves an important anatomic role—separating the mobile abdominal contents from the compressible lung. Defects in this anatomic barrier result in herniation of abdominal contents into the chest, which can lead to both malfunction/failure of the abdominal organs, and dysfunction of the lung. This anatomic role of the diaphragm, like its physiological role in ventilation, serves at bottom to preserve a fully functioning respiratory system such that oxygenated blood can efficiently reach the heart.
The diaphragm is made up of two muscular parts and a central tendon. The muscular portions are the costal diaphragm and the crural diaphragm. The costal diaphragm is its main functional component. It is formed by muscle fibers which originate on the lower six ribs and the xiphoid process and attach on the central tendon (Fig. 148-1). The crural diaphragm consists of bundles of muscle fibers that arise from the first three lumbar vertebral bodies and the medial and lateral arcuate ligaments on each side. Although some mistakenly think of the costal diaphragm as being made up of two separate muscles – right and left hemidiaphragms each forming their own separate dome – in fact the diaphragm is a single, continuous organ that spans the midline as one broad dome.
The importance of the diaphragm’s role in separating the abdominal from the intrathoracic contents is highlighted by the apertures in the diaphragm, through which a number of structures that span each of these cavities must pass. These apertures for the vena cava, the esophagus, and the aorta also allow passage of smaller structures. The vagi and sympathetic trunks pass through the esophageal aperture; the thoracic duct and azygos vein pass through the aortic aperture. Although the apertures are sealed around each of these structures by soft tissue including the mesothelial layer that lines the abdominal surface of the diaphragm and the pleura that lines its thoracic surface, the apertures are nevertheless sites that can allow herniation of visceral contents into the chest. By far the most common acquired diaphragmatic hernias, as discussed in Chapter 46, are hiatal hernias through the esophageal aperture.
Congenital diaphragmatic hernias occur at sites that are compromised by failure of the normal embryological development of the diaphragm. In the embryo, the diaphragm is formed by the fusion of the septum transversum, the pleuriperitoneal membrane, the dorsal mesentery, and the lateral body wall mesoderm (Fig. 148-2). There is also a contribution of myoblasts from the third through fifth cervical somites which migrate to contribute to the formation of muscle fibers. The various congenital diaphragmatic defects can be attributed to failure of one of these processes. For example, posterolateral Bochdalek hernias result from failure of the fusion of the pleuriperitoneal membrane. Morgagni hernias, which occur in the anterior, medial diaphragm posterior to the xiphoid, result from failure of the myoblasts from the cervical somites to appear. The congenital hernias are discussed in Chapter 51.
It is also important to note that the barrier function of the diaphragm fails to a lesser extent in other clinical syndromes that are perhaps less obvious than the diaphragmatic hernias. Some patients appear to have “pores” of varying sizes in the diaphragm which may allow intraabdominal fluid and other materials to pass into the chest. These syndromes were first categorized by Kirchner.1 The most obvious of the Porous Diaphragm Syndromes result from abnormal intraabdominal fluid passing into the chest. The fluid may consist of benign ascites (as in hepatic hydrothorax in cirrhotic patients), malignant ascites (as in Meigs syndrome with ovarian cancer), or peritoneal dialysis fluid (in patients receiving PD), as just a few of the more common examples. All of these tend to occur in the right hemithorax, perhaps because peritoneal fluid circulates such that it pools in the right subphrenic space, or because the presence of the liver on the right creates a sumping action upon the right hemidiaphragm. These problems can often be solved by: (1) at least temporarily minimizing as much as possible the abdominal fluid component by drainage or medical therapy, and then (2) creating a basilar pleurodesis with talc or mesh that will allow the adherent lung to occlude the pores. Depending upon the chronicity of the pleural effusion, a formal decortication may be required as part of this procedure, and if the pores are large, success may be improved by actual pore suture or stapling rather than relying upon a pleurodesis alone. The thoracic procedure is generally carried out by video-assisted thoracoscopic surgery (VATS). Examples of this type of approach are described in publications by Cerfolio and Bryant2 for hepatic hydrothorax and Mak et al.3 for effusions related to PD.
Catamenial pneumothorax refers to pneumothorax that occurs cyclically with menses. This is thought to result in some cases from implants of endometriosis on the lung (which may lead to direct leakage of air from the visceral pleural surface during the hemorrhagic phase within the implants), and in other cases from implants on the diaphragm which may in a similar fashion lead to diaphragmatic defects through which air may enter the chest from the peritoneal space. Implants in both of these locations have been identified during thoracoscopic exploration in a high percentage of patients with this syndrome. It is becoming clear that creating a particularly effective pleurodesis at the level of the diaphragm is an important component of successful management of these patients (as with other “porous diaphragm syndromes”) in addition to the more standard pleurodesis or pleurectomy covering the remainder of the chest.4 Resection of any blebs and visceral pleural implants that are seen are of course also performed during these procedures. Hormonal manipulation to prevent menses for several weeks after the time of the surgical procedure has also been suggested.
The diaphragm’s arterial supply derives largely from the right and left inferior phrenic arteries, the intercostal arteries, and the musculophrenic branches of the internal mammary arteries. There is also a minor contribution from the pericardiophrenic arteries which run with the phrenic nerves. Drainage is through the inferior phrenic veins, which drain into the inferior vena cava and through the azygos and hemiazygos systems. There is clearly substantial collateral circulation across the diaphragm, since it does not appear that diaphragm muscle infarcts or suffers necrosis when individual elements of the arterial supply become occluded or are surgically divided.
Diaphragmatic lymphatics form a specialized system which appears to be important in draining fluid from the peritoneal cavity, and perhaps the pleural cavity, and returning it to the vascular system. This fluid appears to enter subperitoneal lymphatic lacunae, which sit between muscle fibers of the diaphragm. From the lacunae, fluid traverses the diaphragm via intrinsic lymphatics to reach collecting lymphatics beneath the diaphragmatic pleura. Both the intrinsic and collecting lymphatics contain valves. The collecting lymphatics drain principally into retrosternal (parasternal) lymphatic trunks that carry lymph to the great veins after it filters through mediastinal lymph nodes.5 Subpleural lymphatic channels along the diaphragm have been demonstrated to drain into lymph vessels that ascend along the inferior pulmonary ligaments, along the esophagus, or between the pulmonary veins, before connecting to the intertracheobronchial lymph nodes and sometimes toas high as the upper mediastinal lymph nodes.6 Thus, lung cancers invading the diaphragm may drain by this alternate route to involve the same mediastinal lymph node chains that receive lymph from tumors within the pulmonary parenchyma.
It is thought that the contraction of the diaphragm creates a sumping action that facilitates absorption of fluid and lymph into all of these channels, making the diaphragm an important contributor to the total volume of lymph flow.
The motor supply to the diaphragm is via the phrenic nerves. These nerves run in very reproducible positions along the anterior scalene muscle and down along the mediastinum on each side. It is critical for thoracic surgeons to be intimately familiar with the anatomy of the nerves along their entire course in order to avoid injury to them (likely the most common cause of diaphragm paralysis) during all thoracic procedures. The costal diaphragm’s innervation derives from the third and fourth cervical segments, while the crural diaphragm’s innervation is from the fifth segment. Thus, spinal cord injury at C4 or higher leads to partial or complete diaphragm paralysis.
The right phrenic nerve passes through the central tendon along the vena cava through the caval aperture. It divides there into anterior, lateral, and posterior branches (Figs. 148-3 and 148-4). On the left, the nerve enters the diaphragm just anterior to the central tendon and sends off similar branches. The locations of these branches have implications for the placement of incisions in the diaphragm that will be least disruptive to innervation (see separate chapter on diaphragm incisions).
In normal individuals there is very little or no cross-innervation between the left and right sides of the diaphragm. The right phrenic nerve supplies only the right hemidiaphragm and the left nerve supplies only the left hemidiaphragm. As a result, injury to one nerve only causes major dysfunction to the ipsilateral diaphragm. In experimental animals, there seems to be some ability of the contralateral, healthy phrenic to eventually, partially reinnervate a denervated diaphragm, but it is unclear if this occurs to any clinically significant extent in humans.
As a result of its circumferential attachments to the ribs and spine, its attachment to a central tendon, and its sheet-like, domed configuration, when the muscle fibers of the diaphragm contract, the sheet-like muscle descends caudally. Wade measured the displacement of the diaphragm’s dome with inspiration at 9.5 cm on average.7 The diaphragm essentially acts as a piston, descending caudally with contraction. This diaphragmatic descent increases the intrathoracic volume, thereby reducing the intrapleural pressure. A lesser component (approximately 10%) of the reduction in intrapleural pressure with diaphragm contraction results from what is called the “insertional component” – or the diaphragm fibers’ insertion on the superior margin of the lower ribs – which during contraction cause the lower ribs to expand outward (the increase in intraabdominal pressure may also contribute to this action). The resulting reduced intrapleural pressure expands the lung, drawing air into the lungs via the airways. As the diaphragm relaxes, it moves passively in a cranial direction, allowing air to passively exit the lung as a result of the intrinsic elastic recoil of the lung tissue and chest wall.
When the diaphragm is dysfunctional or ventilatory requirements are increased by exercise, other inspiratory muscles – which do not contribute much during quiet breathing – may become active. These include mainly the scalene muscles, the parasternal intercostal muscles, the sternocleidomastoid muscles, the transversus abdominis muscle, and possibly the external intercostal muscles.
As in limb muscle, shifts in the distribution of the various muscle fiber types within the diaphragm (e.g., slow or fast fibers) occur in response to certain stressors. These shifts may be adaptive, or in some cases perhaps maladaptive (see below discussion regarding changes in fiber types in emphysema).
The sensation of dyspnea remains poorly understood, but appears to result from a very complicated set of neurophysiological events. Interestingly, it has been clearly shown that dyspnea is far more closely related to respiratory muscle function than it is to FEV1. To summarize a large body of research in a few sentences, one might say that one of the most important contributor to the sense of dyspnea in many patients is the work of breathing (WOB).
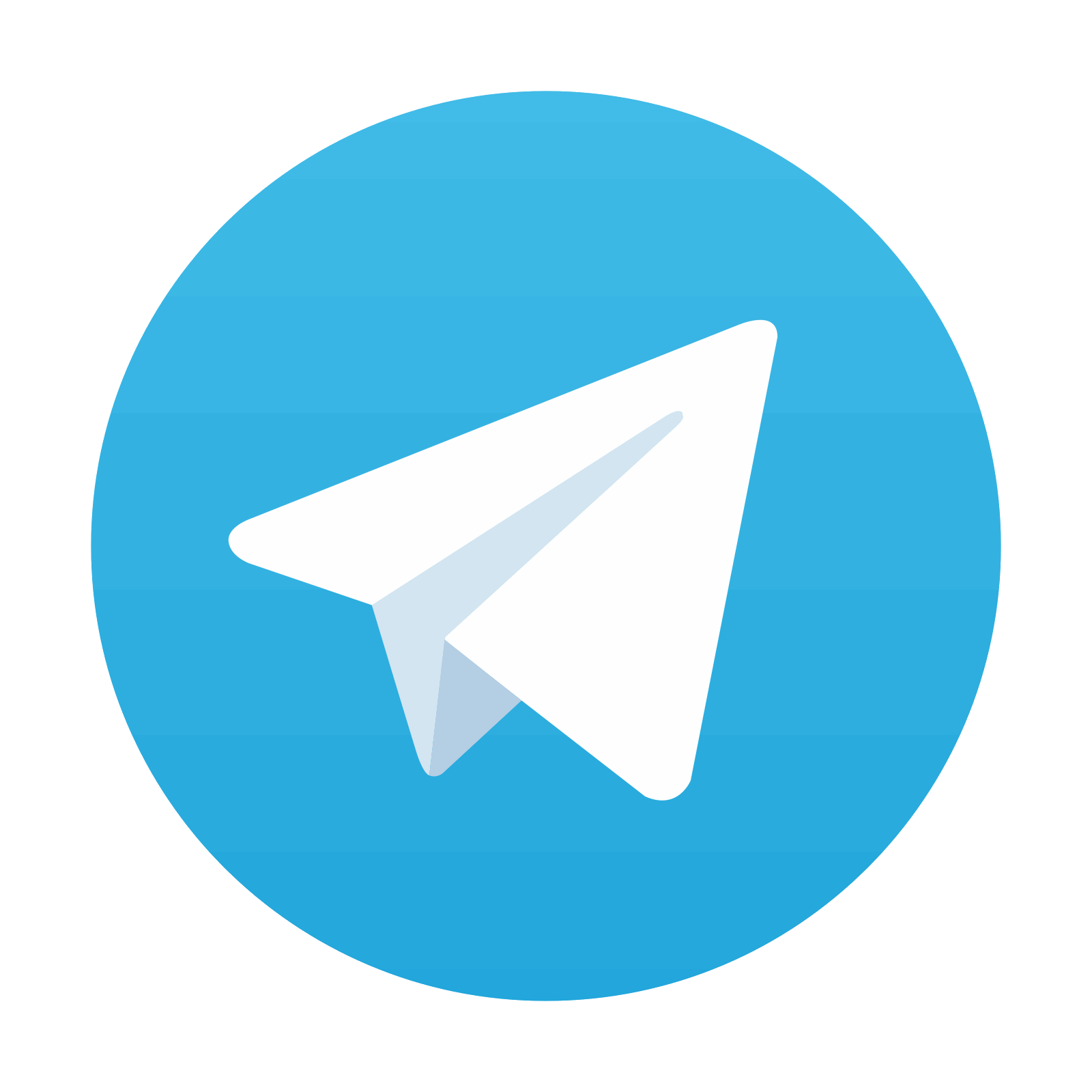
Stay updated, free articles. Join our Telegram channel

Full access? Get Clinical Tree
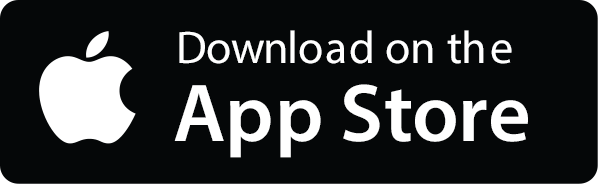
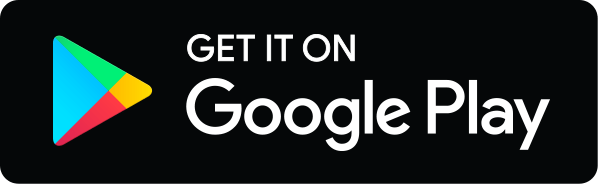