Fig. 13.1
A diagram demonstrating the collaboration and interaction of alloimmune-dependent and independent factors that influence the pathogenesis of transplant vasculopathy. Abbreviations: Ag antigen, CD cluster of differentiation, eNOS endothelial nitric oxide synthase, and SMC smooth muscle cell (Reused with permission from Schmauss and Weis [1])
Clinical Features
The process of heart transplant causes denervation of the transplanted graft. Due to cardiac denervation, CAV may present insidiously. Cardiac angina is typically absent due to lack of cardiac afferent nerves. Shortness of breath or atypical symptoms may accompany CAV, but CAV may be asymptomatic. CAV may present with left ventricular systolic dysfunction, but even with severe CAV, left ventricular systolic function may be preserved. Significant CAV is often accompanied by restrictive allograft physiology. Restrictive physiology is defined as symptomatic heart failure with either echocardiographic or hemodynamic abnormalities on right heart catheterization. Echocardiographic parameters consistent with restrictive allograft physiology in adults include E/A velocity ratio > 2, decreased isovolumic relaxation time <60 ms, shortened mitral valve deceleration time <150 msec. Restrictive hemodynamics on right heart catheterization include right atrial pressure > 12 mmHg, pulmonary capillary wedge pressure >25 mmHg, and cardiac index < 2 L/min/m2. A consensus statement was published in 2011 by the International Society for Heart and Lung Transplantation (ISHLT) that established a working formulation of standardized nomenclature for CAV (Table 13.1). By this form of classification, CAV can be separated into not significant (CAV0), mild (CAV1), moderate (CAV2), and severe (CAV3) disease [15].
Table 13.1
The International Society for Heart and Lung Transplantation nomenclature of cardiac allograft vasculopathy
Grade of CAV | Disease severity | Angiographic findings |
---|---|---|
CAV0 | No disease | No detectable angiographic lesion |
CAV1 | Mild | Angiographic LM <50%, or primary vessel with maximum lesion of <70%, or any branch stenosis <70% (including diffuse narrowing) without allograft dysfunction |
CAV2 | Intermediate | Angiographic LM <50%; a single primary vessel >70%, or isolated branch stenosis >70% in branches of two systems, without allograft dysfunction |
CAV3 | Severe | Angiographic LM >50%, or ≥2 primary vessels >70% stenosis, or isolated branch stenosis >70% in all three systems; or ISHLT CAV1 or CAV2 with allograft dysfunction (defined as LVEF <45%, usually in the presence of regional wall motion abnormalities) or evidence of significant restrictive physiology |
Epidemiology
The incidence of CAV increases temporally in a progressive manner. Per the latest registry report from the ISHLT [16], for adult heart transplant recipients, the prevalence of CAV is 7.8% at 1 year post-transplant, 30% at 5 years post-transplant, and 50% 10 years following heart transplant. CAV is the third leading cause of death for heart transplant recipients who are more than 3 years post-transplant. In one early study, mortality was over 50% at 2 years after diagnosis of CAV [17]. Graft failure, which may reflect undiagnosed CAV, is another main cause of mortality post-transplant. In the pediatric population, CAV rates are reduced compared to adult heart transplant recipients with the prevalence of CAV primarily dependent on recipient age at time of heart transplant. In infants and young children (ages 1–5), 31% of heart transplant recipients will have CAV within 11 years post-transplant. For children age 6–10, 43% of transplant recipients will have CAV within 11 years post-transplant. For children age 11–17, 45% of recipients will have CAV within 11 years post-transplant. In the pediatric population, survival was 42–48% in the 6 years after diagnosis of CAV [18].
Diagnosis
Due to the morbidity and mortality associated with CAV, diagnosis of this disease process is critical. The gold standard test for diagnosis of CAV continues to be the conventional coronary angiogram. Interpretation of lumen patency by conventional coronary angiography may give a false sense of security as the lumen of the coronary artery may not be compromised until intimal thickening encroaches and causes focal stenosis (Fig. 13.2). For this reason, many institutions utilize intravascular ultrasound (IVUS) in addition to conventional coronary angiography for assessment of CAV. CAV that presents early post-transplant portends a poor outcome [19, 20]. Although a number of IVUS based parameters have been analyzed post-transplant, change in maximal intimal thickness (MIT) of a matched cross-sectional area of a coronary artery from baseline (approximately 6 weeks post-transplant) to 1 year post-transplant was a reliable surrogate marker for subsequent mortality, nonfatal major adverse cardiac events (MACE), and development of angiographic CAV through 5 years post heart transplant. Patients with MIT increase of 0.5 mm or greater in any matched site of a coronary artery had significantly higher incidence of death or graft loss, nonfatal MACE, and higher incidence of new angiographic CAV [21, 22].


Fig. 13.2
The top half demonstrates the progression of cardiac allograft vasculopathy as demonstrated by right coronary artery angiogram at year 3 post-transplant compared to year 1. Note the multiple, diffuse stenoses (red arrows). The bottom half demonstrates the progression of intimal thickness (red arrow) as demonstrated by intravascular ultrasound at 52 weeks (1 year) post-transplant compared to baseline (week 4 post-transplant). There was a difference of greater than 0.5 mm change between baseline and 1 year. Such a finding is highly prognostic for poor long-term outcomes
Procedures such as conventional coronary angiography and IVUS pose risks that accompany invasive tests. These risks include bleeding, infection, contrast induced nephropathy, peripheral vascular disease, risk of myocardial infarction, dissection or damage of the coronary artery, stroke, and potentially death from the invasive test(s). Due to patient discomfort and the inherent risks of invasive testing, non-invasive tests can be used to assess for CAV. Non-invasive tests for CAV include exercise or pharmacologic-based stress tests, positive emission tomography (PET) testing with coronary flow reserve (CFR), and coronary computed tomographic angiography (CCTA). A normal dobutamine stress echocardiogram predicted an uneventful clinical course [23]. Patients undergoing regadenoson perfusion scans with fixed or reversible perfusion defects had significantly higher risk for death, left ventricular dysfunction, and increased rates of percutaneous intervention within 1 year of abnormal stress test compared to patients with normal perfusion on regadenoson perfusion scan [24]. Patients undergoing PET testing with CFR measurements that had CFR <2 showed higher incidence of left ventricular dysfunction and left ventricular enlargement with stress [25]. Abnormal CFR likely represents microvascular dysfunction not necessarily reflective of epicardial CAV. Although there are limitations of CCTA, it may be a reasonable alternative in patients who have had complications from conventional coronary angiography or for assessment of variant coronary anatomy. As denervation generally leads to higher resting heart rate post-transplant (due to lack of parasympathetic nervous system tone), CCTA can still yield technically adequate results in the post-heart transplant population [26, 27].
Management
Medical
Prevention and treatment options for CAV include medical therapies, modulation of the immune system, mechanical therapies including percutaneous intervention, and redo-heart transplant. The 3-hydroxy-3 methylglutaryl coenzyme A reductase inhibitor, pravastatin, was shown in a prospective clinical trial to reduce the incidence of CAV, reduce cholesterol levels, reduce cardiac rejection with hemodynamic compromise and increase survival at 1 year post-transplant [28]. This study was supported by another prospective trial of simvastatin, a similar drug, which demonstrated superior 8-year survival and freedom from CAV compared to a control group [29]. In another trial, use of vitamins C and E showed no progression of CAV (compared to placebo treated control patients) [30]. Given risk of development of CAV and likely contribution of traditional risk factors to development of CAV, low dose aspirin is generally advised post-transplant. The use of induction agents at time of heart transplant, including T cell depleting agents and interleukin (IL)-2 receptor antagonists, may lead to reduced rates of CAV. Anti-thymocyte globulin (ATG), a commonly used T cell depleting agent, has shown delayed onset of CAV [31] and decreased CAV progression by IVUS parameters between baseline and 1-year post-transplant [32]. Different approaches to maintenance immunosuppression have shown differences in the development of CAV. The purine inhibitor mycophenolate mofetil (MMF) in combination with the calcineurin inhibitor (CNI) cyclosporine showed decreased incidence of CAV [33]. Clinical trials using the proliferation signal inhibitors (PSI) sirolimus or everolimus in conjunction with CNIs have shown reduced rates of CAV compared to maintenance immunosuppressive regimens with CNI in combination with purine antagonist. Everolimus in combination with cyclosporine showed lower rates of CAV compared to cyclosporine with azathioprine [34]. Sirolimus in combination with cyclosporine showed lower rates of CAV compared to cyclosporine in combination with azathioprine [35]. High-dose everolimus in combination with cyclosporine showed harm in one trial, but low-dose everolimus with cyclosporine showed similar mortality compared to cyclosporine with MMF [36]. Everolimus showed efficacy over MMF for CAV in subpopulations including women, diabetics, patients over age 60, and patients with higher cholesterol levels [37]. A more recent study examined low-dose everolimus with reduced-dose cyclosporine versus standard-dose cyclosporine with MMF. Low-dose CNI was withdrawn and PSI dose increased to target levels 7–11 weeks post-transplant. This study also demonstrated lower CAV burden in the PSI arm [38].
Immunomodulation with photopheresis may reduce rates of CAV due to reduction of rejection episodes. Photopheresis involves removal of approximately 5% of peripheral blood lymphocytes. These cells are treated with ultraviolet-A light and methoxsalen. Treated cells are reinfused into the patient [39]. This treatment is thought to cause apoptosis of T cells and creation of regulatory T cells that, in theory, reduce the inflammatory state. Benefit from photopheresis was demonstrated when used empirically post-transplant [40] and for treatment of post-heart transplant rejection with hemodynamic compromise or recurrent heart transplant rejection [41]. Patients treated empirically with photopheresis for the first 6 months post-transplant had reduced rates of acute rejection without increased risk of infection. Using photopheresis in the treatment of patients with rejection with hemodynamic compromise or recurrent rejection decreased the risk of subsequent significant rejection episodes. Reduction of rejection and the inflammatory state may lead to decreased rates of CAV, although this has not formally been studied in a clinical trial format.
Surgical
CAV is generally a pan-arteritis, but it can present with focal stenosis. Percutaneous intervention and stent placement is generally a temporizing measure for CAV. One study showed equivalent outcomes following placement of sirolimus drug eluting stents (DES) compared to bare metal stents for significant CAV [42]. Data with newer everolimus DES suggest durability of stented segments with low rates of target lesion revascularization [43]. Prior attempts at revascularization by coronary artery bypass grafting (CABG) surgery resulted in high post-surgical mortality and low rates of survival 1 year after CABG [44, 45]. CAV is the main cause of need for redo-heart transplant. Annually, 2–4% of heart transplant recipients are redo heart transplant recipients. Unfortunately, survival after redo heart transplant is reduced compared to index heart transplant. For adult recipients, 1 year survival after redo heart transplant is approximately 70% and 10 year survival is 38% [16].
Outpatient Management
CAV diagnosis and treatment is important to the long term management of patients post heart transplant. There are a number of additional factors important to long-term management. Generally, maintenance immunosuppression is most intense in the first month after transplant. The risk for rejection and infection is highest in this time point and outpatient follow-up is most intense in this time period. Patients generally require involvement of one or two caregivers to assist with medication administration, medication adherence, and transportation to frequent clinic visits. Target trough levels of immunosuppressant medications are lowered in time. Decrement in cardiac function may be asymptomatic and therefore cardiac function and lab work, including renal function, are frequently assessed during follow-up. Many programs use a combination of two immunosuppressant medications and prednisone. If a patient avoids rejection, maintains normal LV systolic function, and does not develop DSA, she or he can potentially wean off prednisone in time. After surgery, a patient’s functional status oftentimes improves with cardiac rehabilitation.
Malignancy
The main limitations to long-term survival after heart transplant are CAV, graft failure, and malignancy. The use of chronic immunosuppression after transplant to prevent allograft rejection increases the risk of malignancy in the long term. Recipients of heart transplant may have a pre-existing history of malignancy that, after transplant and on immunosuppression, predisposes them to recurrence of their primary malignancy. For patients with history of low-risk tumors, potential heart transplant recipients need not be delayed in evaluation for heart transplant. For patients with pre-existing cancer with a high risk of recurrence, heart transplant should be delayed for an adequate time as opined by oncology to ensure patients remain free from cancer recurrence. High-risk cancers include melanoma, breast, and colorectal cancer. Although data is limited, there is possible transmission of malignant oncogenic cells from donor to recipient. Caution should be considered in donors with history of renal cell carcinoma with vascular invasion, melanoma, choriocarcinoma, and central nervous system tumors [46]. Malignancy may be discovered in organ donors after the process of organ transplant or may be known at the time of organ transplant. The rate of de novo malignancy is approximately twofold higher in transplant recipients compared to the general population [47]. Proposed mechanisms for increased rates of de novo malignancy include direct effects of immunosuppression, reduced immune surveillance, and expansion of atypical cells. In addition, oncogenic viruses may proliferate in the setting of immunosuppression and contribute to the development of malignancy. Viruses including Epstein-Barr virus (EBV), human herpes virus 8 (HHV-8), human papillomavirus (HPV), human T-cell lymphotropic virus 1 (HTLV-1), and Merkel cell polyomavirus (MCV) have association with particular malignancies. Survival after diagnosis of malignancy depends on many factors including size of tumor, local or distant spread of the tumor, aggressiveness of the tumor, and ability of the patient to tolerate treatments directed against the tumor.
Cardiac transplant may require more intense immunosuppression because of the risk of death with graft loss. Animal studies suggest CNI may promote cancer through increased production of transforming growth factor (TGF) beta [48]. Common malignancies after heart transplant include post-transplant lymphoproliferative disease (PTLD), Kaposi’s sarcoma, skin cancer, lung cancer, and anogenital cancer. PTLD represents a heterogeneous group of lymphoproliferative disorders. EBV infection is associated with PTLD. EBV-seronegative recipients receiving transplants from EBV-seropositive donors are at elevated risk for development of PTLD. With EBV infection, B cells incorporate EBV DNA into the cellular genome, decreasing the rate of apoptosis and leading to cellular proliferation. EBV DNA load is suggestive in the right clinical context for PTLD. Imaging studies including fluorodeoxyglucose (FDG)-positron emission tomography can assess hyper metabolic tissue, but ultimately, diagnosis of PTLD is made on histopathology. Risk of PTLD is highest in the first year after transplant when immunosuppression is most intense. Common sites of PTLD in heart transplant recipients include lung, GI tract, liver, lymph nodes, and disseminated disease. In heart-lung transplant recipients, PTLD is primarily found in the lung. Symptoms are variable with PTLD. PTLD can present with fever, fatigue, malaise, recurrent infections that do not respond to antibiotic therapy, lymphadenopathy, or with significant organ dysfunction. PTLD is generally treated with significant reduction of immunosuppressive therapies. Reduction of EBV can be attempted with the antiviral agent acyclovir or gancyclovir. In high-risk patients, prophylaxis with anti-viral agents can be considered. For treatment of neoplastic B cells, a number of approaches are possible including use of chemotherapy, anti-B cell therapy with Rituximab, use of PSI (and withdrawal of one immunosuppressant agent), and tumor resection [49]. When reduction or withdrawal of immunosuppressant therapies is not effective, mortality from PTLD is high. Kaposi’s sarcoma is associated with HHV-8 and occurs in men at rates threefold higher than is seen in women. Lesions typically affect the legs and cause lymphedema. Skin cancers include squamous cell and basal cell carcinomas, melanoma, and Merkel cell carcinoma. Factors that mitigate risk of skin cancer development include ultraviolet radiation, fair skin, pre-transplant history of skin cancer or actinic keratosis, geographic location, and intensity, duration, and type of immunosuppressant therapy. Use of voriconazole for treatment of fungal infections has been associated with the development of aggressive squamous cell carcinomas [50]. Lung cancer, particularly in patients with prior significant tobacco exposure, is increased in heart transplant recipients. Anogenital cancer occurs in 2–3% of transplant recipients. Lesions may be multiple and extensive and may resemble genital warts. Screening for the presence of malignancy after heart transplant is critical. Dermatologic evaluation should be done to screen for skin cancer. There are no formal guidelines for cancer screening after heart transplant, but regular health maintenance screening would be appropriate.
As with CAV, use of PSIs instead of anti-metabolites may be favorable in the context of malignancy. Transition to PSIs may decrease the risk of development of subsequent malignancies after heart transplant [51]. Additional indications for use of PSIs in this context include history of heart transplant rejection and viral infection with CMV. Although PSIs can cause proteinuria kidney disease, it can be used instead of CNI in a renal sparing effort [52] (see Table 13.2 for a summary of indications). PSI use should be made on an individual basis. Potential risks of PSIs include increased risk of fungal infection, fluid retention, risk of venous thromboembolism, hypertriglyceridemia, oral ulcers, proteinuria renal disease, nausea, diarrhea, leukopenia, and pneumonitis.
Table 13.2
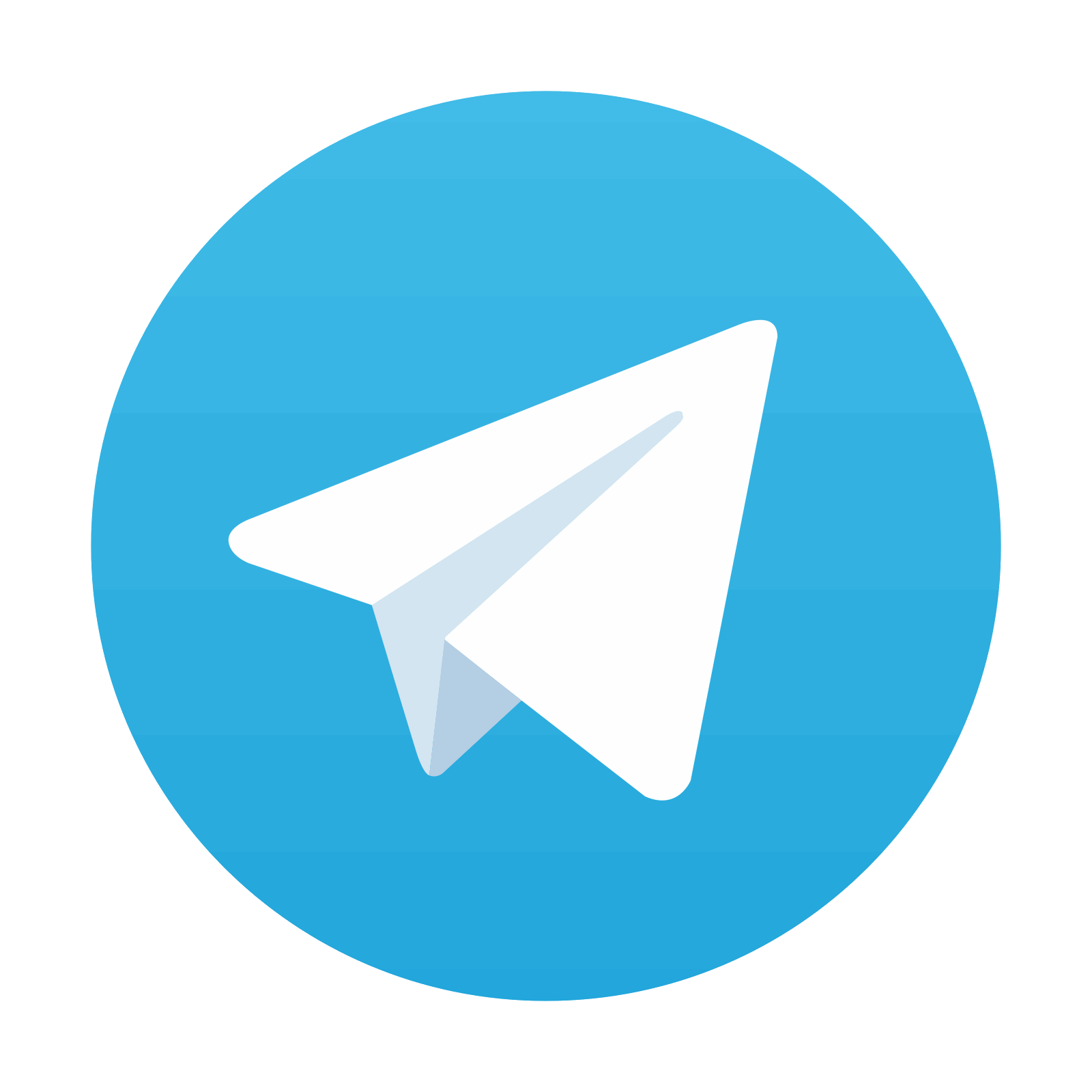
Indications for changing to proliferation signal inhibitors
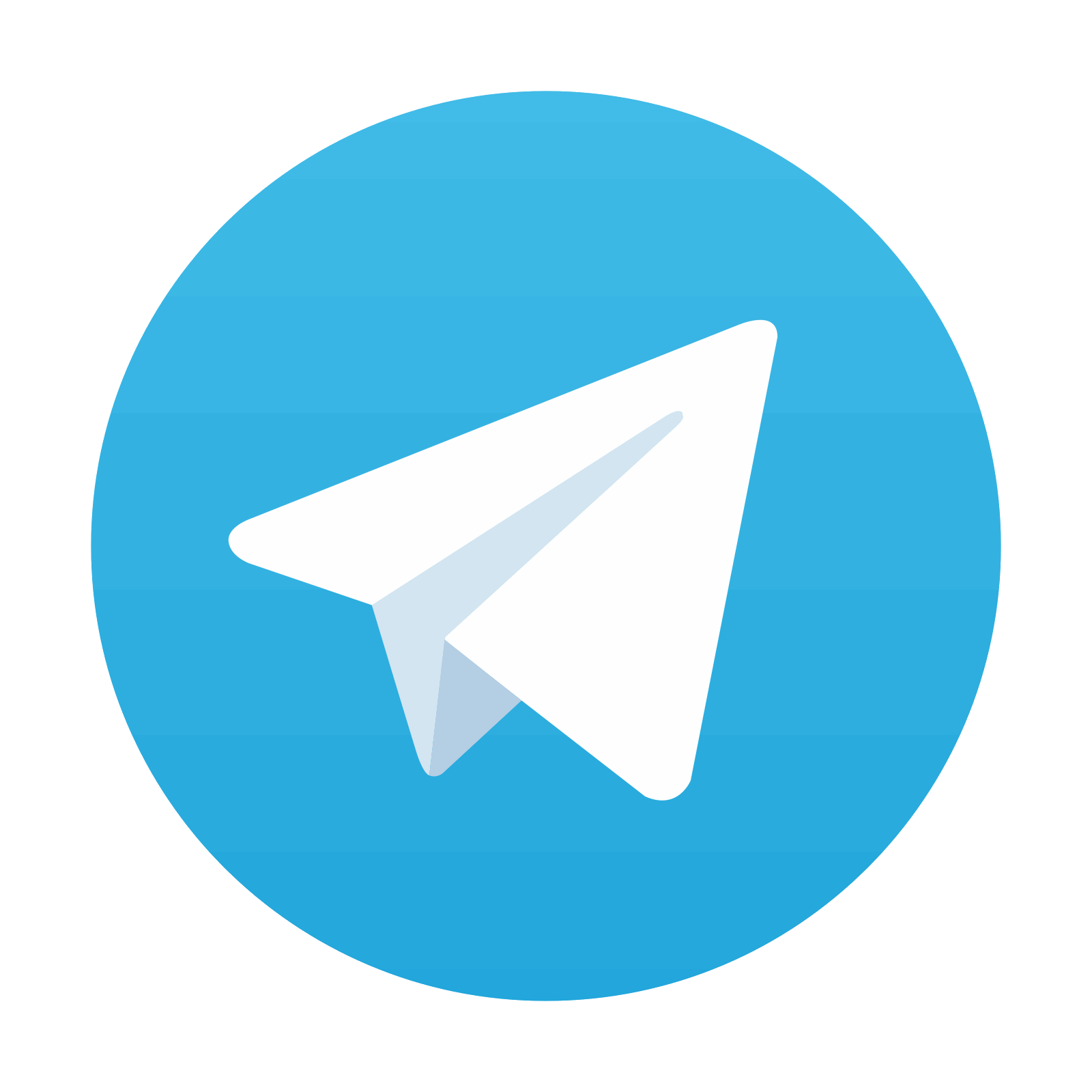
Stay updated, free articles. Join our Telegram channel

Full access? Get Clinical Tree
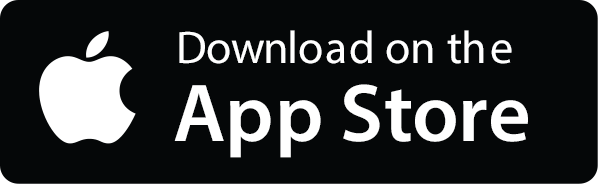
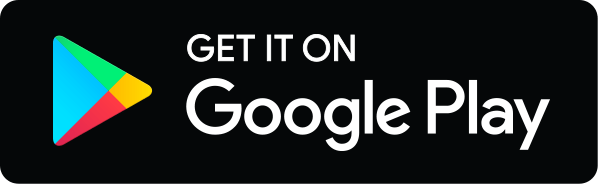
