Fig. 24.1
Example of measuring coronary flow reserve . Coronary flow reserve is calculated as the ratio of hyperemic average peak flow velocity to baseline APV. (APV average peak velocity, CFR coronary flow reserve, DSVR diastolic systolic velocity ratio)
While epicardial segment of coronary system normally does not have any resistance on blood flow, myocardial blood flow becomes lacking as degree of stenosis increases. To offset this, coronary autoregulation works to reduce microvascular resistance to maintain blood supply to the subtended myocardium. However, as epicardial stenosis worsens, increase of coronary blood flow even upon maximal hyperemia becomes insufficient, resulting in reduced CFR. Criterion of CFR for predicting functional significance of coronary stenosis is known to be 2.0 [12, 13].
Meanwhile, since CFR is affected not only by epicardial stenosis but also by microvascular functions, these two factors must be considered together when interpreting an obtained result [14]. While the advantage of CFR is that it is an index which reflects epicardial artery and microvascular function as a whole, CFR is also limited in that it cannot specifically (or independently) evaluate epicardial artery or microvascular function [15]. Also, as CFR is affected by baseline blood flow, it might be lower in case of abnormal increase in baseline blood flow (i.e., high blood pressure, left ventricular hypertrophy, after interventional procedure, acute phase of myocardial infarction). In addition, considering that it is affected by factors such as left ventricular preload and heart rate and that the “normal” cutoff value is not clear, CFR has many limitations to be a specific index for assessing the functional significance of epicardial stenosis [14, 16]. Therefore, rather than functionally evaluating the degree of epicardial stenosis, it has been used for studies mainly focused on evaluating microvascular functions of the infarct-related artery or predicting myocardial viability and/or clinical prognosis after successful revascularization removing epicardial narrowing [17, 18]. According to a recent study that evaluated the prognostic value of CFR and index of microcirculatory resistance (IMR) in patients with FFR > 0.8, even for cases with negative FFR, both CFR and IMR independently showed improvements in risk stratification. Furthermore, prognosis was poorest in the case of low CFR with high IMR [19].
To overcome the aforementioned limitations of CFR, relative CFR (rCFR) was designed. The rCFR is a method which evaluates the degree of stenosis using the ratio of CFR of reference coronary artery without stenosis and the target vessel to be measured. While rCFR better reflects the functional significance of coronary stenosis than CFR, it is more complicated since two vessels are being measured and cannot be used in multivessel disease [20, 21]. Other limitations are that the reference vessel might not be truly normal and that the microvascular status of the two vessels may be different. Therefore, it may be better to use a more specific index for the purpose of evaluating functional significance of epicardial stenosis.
24.2 Hyperemic Stenosis Resistance
The hyperemic stenosis resistance (HSR) has been proposed by the groups of Spaan and Piek as an index indicating the resistance to coronary flow opposed by epicardial stenosis. It is calculated as the ratio of the pressure gradient across a stenosis divided by the coronary blood flow velocity at maximal hyperemia. As Meuwissen et al. demonstrated that it would be a better predictor of reversible perfusion defects caused by a coronary stenosis than either CFR or FFR [22], it can be expected to provide the most accurate information of the functional significance of a given stenosis theoretically since it considers both the pressure gradient and the flow across epicardial narrowing. The measurements require both intracoronary pressure and flow velocity distal to the stenosis in the target vessel using a pressure-sensor guidewire and a Doppler-tipped guidewire . To overcome the limitation makes its practical application difficult and expensive, and a single wire with dual sensor (ComboWire®; Volcano Corp., San Diego, CA, USA) has been recently developed and became clinically available for combined measurements. Its accuracy has been proven to be higher than measuring with two separate single-sensor Doppler wire and pressure wire [23]. Figure 24.2 demonstrated an example of HSR measurement. High HSR values indicate poor clinical outcomes [24]; however, further study is needed to establish a simple cutoff value and its clinical utility.


Fig. 24.2
Measurement of hyperemic stenosis resistance index. Hyperemic stenosis resistance is the ratio of transstenotic pressure gradient to average peak velocity during maximal hyperemia. (APV average peak velocity, FFR fractional flow reserve, HSR hyperemic stenosis resistance, Pa aortic pressure, Pd distal coronary pressure). Modified with copyright permission by Elsevier
24.3 Resting Indices
Although functional assessment of coronary narrowing at rest has been available since the early period of coronary intervention [25], it had not been used in clinical practice due to crucial limitation by bulky low-fidelity equipment. Meanwhile, maximum hyperemia ought to be induced to discriminate between stenoses by increasing flow across them using pharmacologic agents. However, there have been concerns regarding limitations of exogenous induction of hyperemia and needs and efforts for developing a reliable resting physiologic index [26–28]. By reducing procedural time and cost, avoiding adverse effects or patients’ discomfort due to hyperemic agents, and allowing continuous online measurements, nonhyperemic resting indices are appealing to evaluate functional severity of epicardial stenosis.
24.3.1 Instantaneous Wave-Free Ratio
The instantaneous wave-free ratio (iFR) is the ratio of resting distal coronary pressure to aortic pressure, during diastole, when microcirculatory resistance is “naturally” constant and minimized compared with the rest of the cardiac cycle [29]. It is calculated as the mean distal coronary pressure (Pd) divided by the mean aortic pressure (Pa) during the diastolic wave-free period which extends from 25% of the way into diastole to 5 ms before the end of diastole. The concept is based on that resting blood flow was preserved across any given coronary stenosis, and this likely occurs by the vasodilatory compensation of microvasculature for the epicardial stenosis at the expense of Pd, which falls even at rest. During the wave-free period when the resistance waves are quiescent with constantly minimized (Fig. 24.3) [30], pressure and flow velocity linearly correlate and pressure ratios across a stenosis can reflect the flow limitation imposed by itself. No need for pharmacologic induction of hyperemia is the most significant practical advantage of iFR, facilitating the adoption of invasive coronary physiology. iFR has been rigorously validated to be closely correlated with FFR and proposed as a good surrogate for FFR. By comparing iFR and FFR in a routine clinical population, the ADVISE registry found a classification match of 80%, which is similar to the classification match between repeated measures of FFR in the DEFER trial (85% match) [31]. When iFR and FFR were compared to the HSR as third-party arbiter of ischemia in the CLARIFY study , both iFR and FFR had equal diagnostic efficiency to match an ischemic classification with HSR (both 92%, with no significant difference between the two tests) [32]. To verify its clinical utility more clearly, several clinical trials are now in progress to investigate the value of various clinical strategies based on iFR measurements (iFR SWEDEHEART NCT02166736, DEFINE-FLAIR NCT02053038, SYNTAX-2 NCT02015832, J-DEFINE NCT02002910). While awaiting clinical outcome data, the hybrid iFR-FFR strategy can be applied in daily clinical practice [33]. This strategy aims to achieve a high diagnostic agreement with FFR that has validated outcome data, while reducing the need of inducing maximal hyperemia. After iFR is measured in all patients, if the value is within a narrow range, then a hyperemic agent such as adenosine is administered to measure FFR. It is possible to use various hybrid iFR-FFR approaches based on the classification match sought: a match of 95% requires adenosine if iFR values are between 0.86 and 0.93, sparing almost 60–70% of patients from adenosine administration [33, 34]. This hybrid approach is being used in SYNTAX-2 . Furthermore, DEFINE-FLAIR and the iFR-SWEDEHEART are ongoing to assess the validity and safety of single threshold of significance, iFR 0.90, which was determined against an FFR threshold of 0.80 in the RESOLVE study [35].


Fig. 24.3
Illustration of the distal pressure traces and instantaneous resistance for the instantaneous wave-free ratio measurement. Instantaneous wave-free ratio is the ratio of coronary pressure distal to the stenosis to aortic pressure during a specific period of diastole extending from 25% of the way into diastole (a) to 5 ms before the end of diastole (b). (Pa aortic pressure, Pd coronary pressure distal to the stenosis). Modified with copyright permission by Elsevier
24.3.2 Resting Pd/Pa
Interest has recently emerged as to whether nonhyperemic measure of pressure might be useful to evaluate the severity of coronary stenosis. Resting Pd/Pa is another nonhyperemic index, the ratio of distal coronary artery pressure to aortic pressure over the entire cardiac cycle at baseline. It is calculated in similar fashion to iFR except that the pressure measurements were time averaged over the entire cardiac cycle, thus including both systole and diastole. The most practical advantage of resting Pd/Pa is that it is always available before hyperemic measurements such as FFR. A cutoff value of Pd/Pa ≈ 0.90 has been shown to provide the best classification match, approximately 80%, with the clinically adopted FFR cutoff 0.8 [33, 35–37]. The combined application of resting Pd/Pa with FFR seems to provide a more comprehensive physiological assessment of coronary stenosis and a closer pressure-based appraisal of the flow reserve for the subtended myocardium [38]. iFR predicts the FFR value with the same accuracy as the mere baseline Pd/Pa ratio [39]; however, a recent study from Korea comparing resting indices demonstrated that iFR and the whole-cycle resting Pd/Pa had excellent diagnostic accuracy compared with FFR, with iFR demonstrating greater discriminatory power than resting Pd/Pa [40], which was similar to the result ADVISE registry [31].
24.4 Summary
Although FFR is the most verified index in evaluating inducible myocardial ischemia caused by epicardial narrowing and now regarded as the reference invasive method to functionally assess CAD, there is still room for further improvement in the diagnosis and treatment guidance of patients using other physiologic indices (Table 24.1). With rapidly advancing technology in catheterization laboratories, nonhyperemic indices are increasingly applied for functional assessment of coronary stenoses in place of hyperemic indices. In addition, using the combination of pressure and flow velocity is emphasized to understand the entire coronary physiology profoundly and improve accuracy of physiologic evaluations. Since the ischemic heart disease cannot be solely explained by epicardial stenosis, a more integrated physiologic approach considering pressure, flow, and resistance for the entire coronary system may lead to better treatment strategies in combination with epicardial indices such as FFR.
Table 24.1
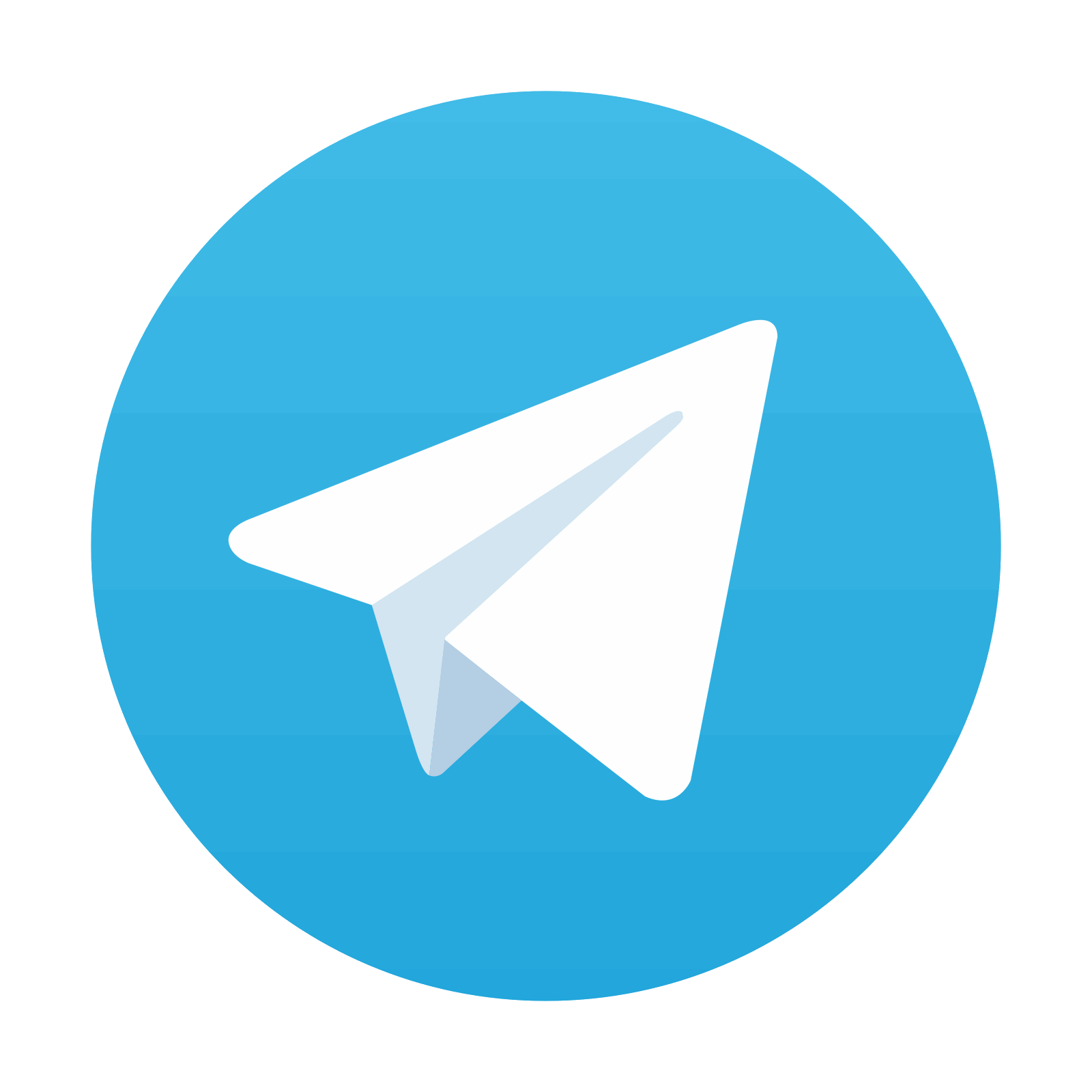
Characteristics of FFR, iFR, and resting Pd/Pa
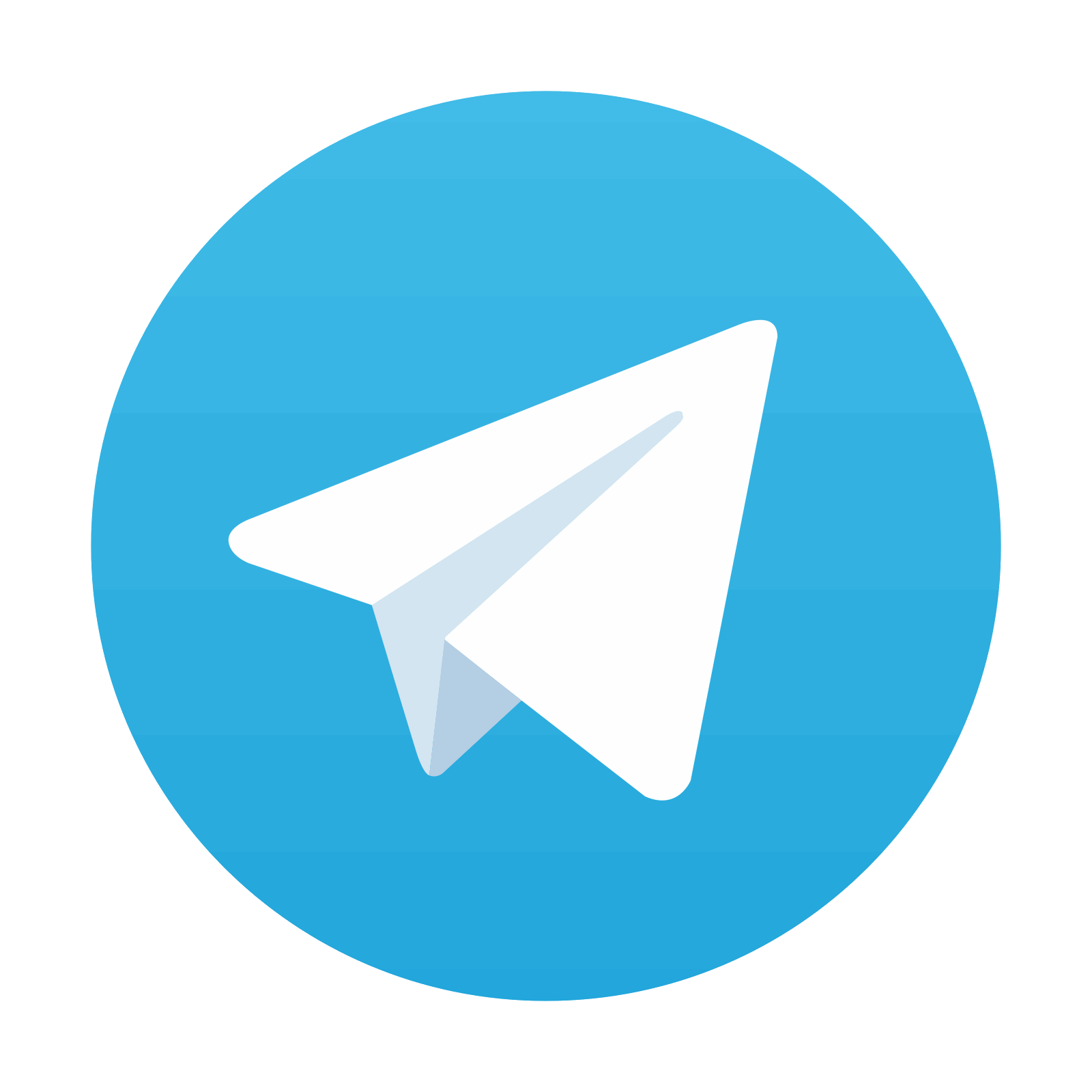
Stay updated, free articles. Join our Telegram channel

Full access? Get Clinical Tree
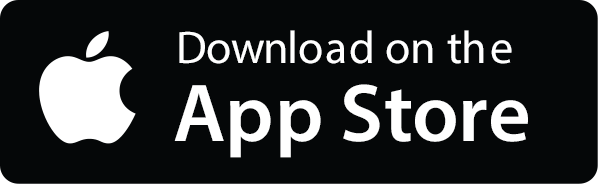
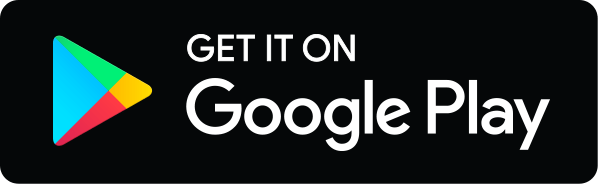
