© Springer International Publishing Switzerland 2015
Enrico Agabiti Rosei and Giuseppe Mancia (eds.)Assessment of Preclinical Organ Damage in Hypertension10.1007/978-3-319-15603-3_1616. Other Methods to Assess Renal Damage
(1)
Hypertension Unit, Internal Medicine, Hospital Clinico, INCLIVA Research Institute, University of Valencia, Valencia, Spain
(2)
CIBERObn, Health Institute Carlos III, Madrid, Spain
16.1 Introduction
Although glomerular filtration rate (GFR) and proteinuria remain gold standard in the evaluation of renal target organ damage, imaging techniques and image-derived parameters allow for both assessment of renal function and estimation of cardiovascular risk. Enhancement in digital image processing permits dynamic measurement of renal vascular and intraparenchymal processes in real time. The most relevant in terms of clinical use to assess hypertension-induced organ damage are the renal resistive index (RRI), renal calcium score, and functional magnetic resonance imaging.
16.2 Ultrasound and Doppler-Derived Renal Resistive Index
Ultrasound and Doppler-derived indexes of renal blood flow are standard procedures in the evaluation of acute and chronic kidney disease (CKD), regardless of the underlying cause. Doppler ultrasonography allows for detection of both macrovascular and microvascular abnormalities, together with structural or functional changes of the renal parenchyma, deduced from vascular impedance patterns [1].
The ESH recommends performing renal ultrasound and renal duplex Doppler ultrasonography in hypertensives when a secondary form of hypertension is suspected. However, current evidence suggests that ultrasound Doppler-derived RRI might be a good predictor of subclinical renal, vascular, and target organ damage as well as cardiovascular risk [2, 3].
The RRI is calculated by dividing the difference of the peak systolic velocity and the end diastolic velocity by the peak systolic velocity derived from intrarenal arteries, considering a range of 0.47–0.7, with a difference between the two kidneys of <8 % as normal in the adult population. Vascular compliance and resistance are the main determinants of RRI, and its usefulness has been demonstrated in a broad clinical spectrum, including renal artery stenosis, obstructive renal disease, chronic renal allograft rejection, and prediction of progression risk in CKD and critically ill patients, among others [4].
In the hypertensive population, the relationship between RRI and kidney injury is well established. Alteration of the vascular resistance at the renal parenchymal level is associated with a decline in glomerular filtration rate. Furthermore, RRI has shown to maintain a positive direct relationship with the quantity of UAE, reflecting its potential as a marker of cardiovascular risk. The interaction between renal impedance and vascular hemodynamics in the presence of hypertension has been further investigated. RRI is associated with age, blood pressure including abnormal circadian patterns, pulse pressure, and arterial stiffness. Taking the current evidence together, RRI may be considered as a monitor of the hemodynamic properties in hypertensive subjects [5–8].
Hypertension-induced target organ damage in other territories is also closely related to renal vascular patterns. Patients with a RRI beyond the threshold of 0.7 have higher pulse wave velocity, left ventricular mass, coronary artery calcification, and intima-media thickness than subjects with normal values of RRI. Interestingly, these observations are independent of the degree of renal functional impairment and therefore permit the usage of RRI in both hypertensives without apparent target organ damage and subjects with high cardiovascular risk [9, 10].
Renal impedance expressed by RRI is closely related to central and peripheral hemodynamics. In addition, RRI has shown to be a predictor of the development of diabetes mellitus and hyperuricemia in the hypertensive population. These findings suggest that RRI might be a useful tool for the prediction of cardiovascular events. Up to now, only a few prospective studies have addressed this question. However, recent evidence shows that high RRI values independently predict all-cause mortality and cardiovascular events (ischemic cardiomyopathy, congestive heart failure, stroke, and transient ischemic attack) in hypertensive subjects [11, 12].
The impact of treatment reducing RRI and consecutive changes on the prognosis of cardiovascular events remains uncertain. Blood pressure-lowering treatment has shown to reduce RRI and parallel urine albumin excretion, but long-term studies are necessary to determine whether the decline in RRI is followed by a significant reduction of cardiovascular risk [13, 14].
16.3 Computed Tomography and Renal Artery Calcium
Multidetector computed tomography has gained importance in vascular medicine due to its ability to measure arterial wall properties and to detect vessel alterations. The presence of vascular calcium in coronary and peripheral arteries has shown to maintain a direct relationship with systemic atherosclerosis. Among others, coronary artery calcium is a well-established marker of asymptomatic organ damage and has been validated as a predictor of cardiovascular disease.
Renal artery calcium (RAC) can be assessed noninvasively using the multidetector or the electron beam computed tomography. To determine and quantify the arterial calcium, regions of interest are identified as having a density of more than 130 Hounsfield units (HU) in an area larger than or equal to 1 mm2. Detectable RAC is commonly classified with the Agatston scoring method adjusted by slice thickness. The established threshold for the presence of RAC is defined as a density of more than 130HU in a given vascular area [15].
The prevalence of RAC differs among the reported data and depends on the studied subpopulation. While RAC is identified in about one third of the general population, the prevalence rises up to 80 % in diabetic patients [16]. However, the presence of RAC is closely related to hypertension, cardiovascular risk factors, systemic atherosclerosis, and end-organ damage of the kidney. Recent evident shows that the presence of RAC results in twofold higher odds of microalbuminuria and hypertension compared to subjects without renal artery alterations. The risk of progression to end-stage renal disease is greater in patients with RAC, independent of other risk factors, favoring the role as a prognostic tool of CKD. Also, RAC has shown to be associated with coronary artery calcium and, more importantly, an increased risk of cardiovascular mortality. Interestingly, there is no independent association between RAC and patients with diabetes mellitus, despite the greater burden of vascular calcium in this subpopulation group. Therefore, the detection of RAC in diabetics might serve as a predictor of systemic vascular calcium [17–20].
Despite the promising properties of RAC in the assessment of cardiovascular disease, there are currently no studies available considering RAC as a treatment target. The question of whether specific treatment is able to reduce RAC and consecutively the cardiovascular risk remains unanswered.
16.4 Functional Magnetic Resonance Imaging
Functional magnetic resonance imaging (MRI) of the kidneys has gained ground in the past several years. MRI permits in vivo measurement of renal perfusion, filtration, diffusion, and oxygenation and provides several benefits compared to other (imaging) techniques to assess renal function: no exposure to ionizing radiation, avoidance of iodinated contrast agents, noninvasiveness, and ability to determine renal function for each kidney separately [21].
There is a lack of current evidence on long-term prospective studies to assess the impact of cardiovascular risk factors and the corresponding treatment on MRI-based determination of renal function. However, various techniques available to assess GFR are described as followed.
16.4.1 Dynamic Contrast-Enhanced MRI
Dynamic contrast-enhanced (DCE) MRI, also termed MR renography, is based on the acquisition of dynamic images before, during, and after administration of gadolinium chelate. The signal intensity of the renal tissue is converted to gadolinium concentration and plotted versus the time to generate various functional parameter curves. With this method, it is possible to resolve the renal cortex from the medulla and collecting system, and it offers the potential to measure renal properties in vivo that previously could only be assessed by biopsy. Current applications of DCE MRI include estimation of GFR as well as diagnostics of renovascular hypertension, functional urinary obstruction, and renal allograft dysfunction [22–24].
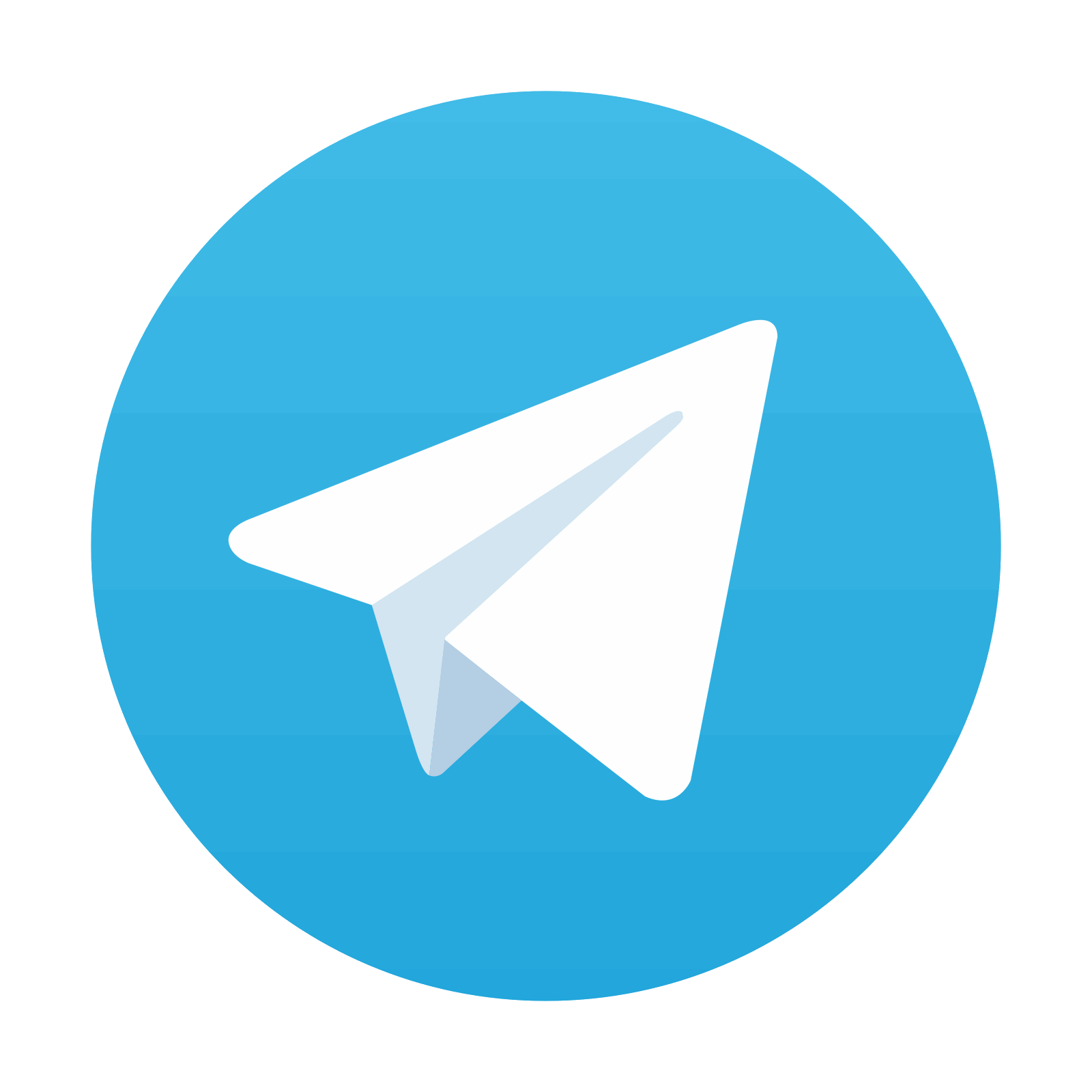
Stay updated, free articles. Join our Telegram channel

Full access? Get Clinical Tree
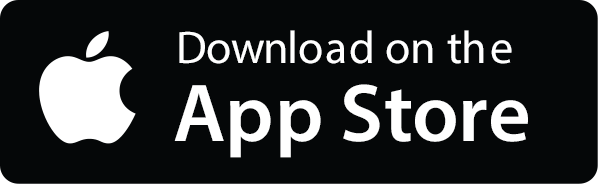
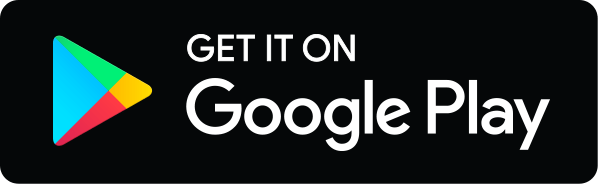