Neurologic conditions other than acute altitude illness While much of the attention regarding lowlanders traveling to high altitude is focused on the acute altitude illnesses discussed in Chapters 21–23, a range of other medical problems have been documented in travelers to high altitude and warrant further consideration. This chapter will focus on several general categories of problems including thromboembolic disease, ophthalmologic problems, altitude-related cough, sudden cardiac death, and neurologic conditions other than acute mountain sickness (AMS) and high altitude cerebral edema (HACE). The focus throughout the chapter will be on problems that occur in unacclimatized individuals traveling to higher elevations, rather than medical issues seen in chronic residents of high altitude. In considering these entities, it is important to note that, in comparison to the acute altitude illnesses, there is considerably less systematic information regarding their epidemiology, pathophysiology, and management. In some cases, this is related to an overall lack of attention to the topics in the research literature, whereas for others, the incidence of the disorders is low, limiting the literature to case reports and small case series and, therefore, making it difficult to conduct systematic analyses of each problem. Over more than a century, numerous cases of arterial or venous thromboembolism have been reported at high altitude, raising an, as yet, unconfirmed suspicion that hypobaric hypoxia at high altitude provokes a hypercoagulable state that predisposes to these adverse events. The thromboembolic events described in the literature generally fall within two broad categories. Cerebrovascular accidents or strokes, which can be distinguished from cerebral edema by their rapid onset and focal neurologic deficits, have been reported at high altitude as far back as the late 19th and early 20th centuries when Roborovski described his own hemiparesis and aphasia while exploring Tibet’s Amne Machin range (Roborovski 1896), and others described onset of hemiplegia in a Gurkha soldier and Sherpa porter on the 1924 and 1936 Everest expeditions, respectively (Norton 1925; Tilman 1948). Most recently, Jha et al. (2002) reported a series of Indian soldiers who developed strokes while stationed at high altitude, and Wilson et al. (2010) detected a middle cerebral artery infarction in the field using transcranial Doppler ultrasound and several reports describe cerebral infarction associated with paradoxical embolism through a patent foramen ovale (Chandler and Mellor 2016; Murdoch 2015). Transient ischemic attacks (TIAs) have also been described. Wohns (1986), for example, reported TIAs in three separate individuals during high altitude climbs in three separate individuals, including hemiplegia, hemiparesis, aphasia, and scotomas, while Murdoch (1996) reported cases with various focal neurologic defects at altitude that also demonstrated rapid recovery, consistent with TIA. Without the aid of diagnostic studies, however, it is difficult to conclude that these cases were due to thromboembolism rather than ischemia due to volume depletion in the setting of severe hypoxemia, for example. In addition to the arterial circulation phenomena described above, there have also been multiple reports of cerebral venous thrombosis (Boulos et al. 1999; Cheng et al. 2009; Hassan and Kumar 2013; Torgovicky et al. 2005). For example, Shrestha et al. (2012) reported a 47-year-old woman who presented with headache, speech deficits, and visual changes, and was found to have a cerebral venous sinus thrombosis, while Khanal et al. (2016) described a similar finding in a 35-year-old man who presented with headache and diplopia while climbing Mount Everest. As discussed further, the presence of a hypercoagulable state may predispose to such events, as a recent review of cerebral venous thrombosis at high altitude (Zavanone et al. 2017) found that thrombophilias, such as secondary polycythemia, protein C or S deficiency, and factor V Leiden mutations were found in nine of 17 patients with this problem. Viewed together, these cases of arterial and venous thrombosis demonstrate the need to consider alternative diagnoses to AMS and HACE when neurologic symptoms do not fit the typical pattern of acute altitude illness or fail to resolve with appropriate therapy or descent. Venous and arterial thromboembolism involving sites outside the central nervous system have also been described. In addition to the noteworthy case of Art Gilkey who developed deep venous thrombosis of the lower extremities during the 1954 American expedition to K2 (Houston and Bates 2008), there have been multiple reports of pulmonary embolism (Ashraf et al. 2006; Hull et al. 2016; Pandey et al. 2016). Because signs of deep venous thrombosis such as asymmetric lower extremity swelling are not universally present and CT imaging capabilities are typically unavailable in remote high altitude settings, distinguishing pulmonary embolism from high altitude pulmonary edema can be difficult in the field, and diagnosis may be delayed until following descent (Pandey et al. 2016). Aside from pulmonary embolism, Fagenholz et al. (2007) reported the case of a previously healthy man who presented at 4240 m with a pale, cold right lower extremity due to thrombosis of the right superficial femoral artery at its transition to the popliteal artery and later required a below-knee amputation, while Anand et al. (2005) have described 26 cases of portal venous thrombosis in Indian soldiers stationed above 3000 m. As noted earlier, the large number of reported cases of thromboembolic disease has long raised suspicion that hypobaric hypoxia predisposes to the development of a hypercoagulable state. To date, however, there has been insufficient evidence to support such a claim or other purported etiologies, such as dehydration and/or prolonged periods of immobility in a tent. Several large, retrospective series have attempted to evaluate whether the risk of thromboembolic events is increased at high altitude. For example, Anand et al. (2001) retrospectively reviewed the records of more than 20,000 hospital admissions in India over a three-year period and found a higher incidence of venous and arterial thrombosis among high altitude dwellers compared to those from low altitude regions, while Jha et al. (2002) used a similar retrospective approach and reported a higher incidence of stroke in soldiers stationed at high altitude compared to those at lower elevation. The conclusions of these studies, however, are limited due to methodological concerns such as the narrow patient populations examined (e.g., military soldiers), limited diagnostic evaluations, and the retrospective nature of the analyses. They also provide no information regarding risks associated with short-term stays at high altitude, as each of these studies concerned longer-term exposures. Zafren et al. (2011) sought to evaluate the risk associated with short-term exposures in a study of climbers on Mount Everest. They characterized the incidence of deep venous thrombosis in asymptomatic individuals by performing d-dimer testing in all individuals followed by lower extremity compression ultrasound in those individuals with a positive test. Zero out of the 76 climbers had a positive test and no ultrasounds were necessary, suggesting that the incidence of asymptomatic deep venous thrombosis is low. In the absence of systematic studies demonstrating increased risk of thromboembolism at high altitude, many studies have examined changes in coagulation parameters as surrogate measures for thromboembolic risk. This approach has also not yielded convincing evidence of a hypercoagulable state at high altitude. Although there is consistent evidence that the activated partial thromboplastin time is shorter during acute hypoxic exposures (Bartsch et al. 1988; Maher et al. 1976; O’Brodovich et al. 1984), the literature contains conflicting results about the effects of acute hypoxic exposure on platelet function (Chatterji et al. 1982; Gray et al. 1975; Lehmann et al. 2006; Maher et al. 1976; Sharma 1980), bleeding times (Bartsch et al. 1989; Doughty and Beardmore 1994), and other biochemical markers of coagulation activity, such as markers of fibrinolytic activity (Bartsch et al. 1988; Mannucci et al. 2002) and thrombin formation (Bartsch et al. 2001; Bendz et al. 2000). One important issue with all of these studies is that they tend to assess particular facets of the coagulation system, such as thrombin-antithrombin complexes, in isolation rather than looking at the function of this complex system as a whole. To address this issue, Martin et al. (2012) performed thromboelastography (TEG), a functional test that examines the kinetics of clot formation and is reflective of all aspects of the clotting system, on healthy volunteers at sea level, 4250 m, and 5300 m, and actually found evidence of slowed coagulation, including increased reaction (R) and kinetic (K) times at 5300 m compared to sea level (Figure 23.1). R-time is a measure of time to clot initiation and initial fibrin formation, while K-time evaluates the time to achieve a certain clot strength due to thrombin and activation of platelets. Figure 23.1Changes in the thromboelastography during ascent to 5300 m. Panel A depicts changes in the R-time, a marker of the time to clot initiation. Panel B depicts changes in the K-time, a marker of the time to achieve a certain level of clot strength. Data were collected from 17 healthy volunteers. The gray area denotes the normal range for whole blood samples with kaolin. * denotes statistically significant difference relative to sea level. (Source: Martin et al. 2012.) In the absence of clear evidence that hypobaric hypoxia causes hypercoagulability, some other factor is likely responsible for the observed cases of thromboembolism at high altitude. Further insight into this issue comes from a study by Schreijer et al. (2006) in which they examined 71 healthy volunteers and found increased levels of thrombin-antithrombin complexes following eight hours of air travel at cabin pressures equivalent to 1800–2100 m compared to following the nonhypoxic exposures. Of note, the greatest changes were noted in those volunteers with the factor V Leiden mutation who used oral contraceptives, which suggested that the presence of a pre-existing coagulopathy, rather than hypobaric hypoxia, may be the key variable increasing risk for thromboembolism at high altitude. This result is particularly intriguing because in many of the cases of thromboembolism at high altitude, the affected individual had some underlying predisposition to coagulopathy (Zavanone et al. 2017), such as oral contraceptive use (Shlim and Papenfus 1995), protein C deficiency or S deficiency (Boulos et al. 1999; Nair et al. 2008; Shrestha et al. 2012), hyperhomocysteinemia (Ashraf et al. 2006; Hassan and Kumar 2013), or S-C hemoglobinopathy (Heffner and Sahn 1981). This finding is not universal, however, as the patients in other cases lacked evidence of coagulopathy (Fagenholz et al. 2007). Other factors not accounted for in the studies and reported previously may play a role in some thromboembolic events including a combination of polycythemia and dehydration due to increased insensible losses and urinary losses (Ward 1975), low temperature, use of constrictive clothing, and prolonged periods of immobility while tent-bound during inclement weather (Gupta and Ashraf 2012). Interestingly, even though polycythemia vera is known to increase the risk of thrombotic events, patients with secondary polycythemia at sea level due to, for example, chronic hypoxia do not have similar risk for such problems (Schwarcz et al. 1993). This would suggest that the polycythemia that develops with prolonged stays at high altitude may not predispose to thromboembolism unless there is an additional risk factor, such as dehydration and prolonged immobility. In the end, the evidence regarding coagulation system function at high altitude remains inconsistent and no firm conclusions can be drawn regarding an increased risk of coagulopathy. Lacking evidence that hypobaric hypoxia increases the risk of thromboembolism, there is no indication for pharmacologic prophylaxis, such as aspirin, clopidogrel, subcutaneous heparin, or direct oral anticoagulants when traveling to high altitude. Instead, individuals should simply remain vigilant about maintaining adequate hydration and mobility. Individuals already on therapy for prior venous thromboembolism should continue their anticoagulation at high altitude. Those taking warfarin should arrange for follow up of their pro-time or international normalized ratio (INR) in the event of prolonged stay or upon return following a short stay at altitude, as a retrospective analysis has shown that a change in altitude may lead to deviations in the INR from the therapeutic range (Van Patot et al. 2006). Changes in diet often associated with mountain travel may also alter the INR by changing daily vitamin K intake. Such monitoring issues can be obviated through the use of either direct thrombin inhibitors (dabigatran) or factor Xa inhibitors (apixaban, edoxaban, rivaroxaban), although reversal of such agents in the event of bleeding may more challenging than with warfarin. Patients who develop clinical evidence of thromboembolism should be evacuated to lower elevation as soon as feasible. While awaiting evacuation, supplemental oxygen should be administered to improve tissue oxygen delivery, while anticoagulation with aspirin or low molecular weight heparin, agents that do not require any monitoring of therapeutic levels, can be considered when available. However, given that central nervous system findings may be due to hemorrhage rather than thrombosis, a distinction that can be difficult to make in the field, it would be prudent to hold anticoagulation in cases of acute central nervous system findings until more definitive evaluation is completed. An evaluation for inherited thrombophilia may be warranted following descent. Whether people who have had thromboembolic events at high altitude are at risk for such events with future high altitude travel is unclear. While the most conservative approach is to avoid any further high altitude travel, consideration can also be given to use of prophylactic anticoagulation on future trips, particularly if the individual is discovered to have an inherited thrombophilia. There are several different ophthalmologic issues that may affect travelers to high altitude. Each is considered below. HAR refers to a broad spectrum of retinal disorders, including retinal hemorrhage, retinal vessel engorgement and tortuosity, optic disc hyperemia and swelling, cotton wool spots, and vitreous hemorrhage, that occur following altitudes greater than 3500 m (Bosch et al. 2012). HAR and, in particular, retinal hemorrhages have been documented extensively in the literature (Frayser et al. 1970; Rennie and Morrissey 1975; Wiedman and Tabin 1999; Barthelmes et al. 2011; Ho et al. 2011), with varying incidence between studies. In two larger, more recent studies, Wiedman and Tabin (1999) reported an incidence of 74% in climbers ascending to between 4950 m and 7600 m and 90% in those traveling above 7600 m, while Barthelmes et al. (2011) reported a retinal hemorrhage incidence of 78% among 28 climbers during an expedition to Muztagh Ata (7546 m). The incidence may be affected by the degree and duration of hypoxic exposures with more frequent occurrence in those exposed to higher elevations and/or for longer durations (Barthelmes et al. 2011; Bosch et al. 2012; McFadden et al. 1981). Hemorrhages may be observed during all stages of high altitude travel. In fact, in the study by Barthelmes et al. (2011), during which retinal examinations were conducted at multiple points during the expedition, the majority of hemorrhages were detected following descent to base camp at the end of the expedition. The incidence was highest among those individuals who spent the greatest duration at high altitude and ascended to the highest elevation. While some data suggest that high altitude retinopathy may correlate with development of HACE (Wiedman and Tabin 1999), other studies have not confirmed this relationship (Barthelmes et al. 2011). The precise etiology of retinal hemorrhages remains unclear. They may be related to hypoxic injury to the retinal vessels or increased cerebral blood flow secondary to hypoxemia with subsequent increases in retinal vessel flow. Frayser et al. (1970), for example, have shown that blood flow through the retinal vessels is increased by 105%, while Rennie and Morrisey (1975) found increases in arterial and venous diameter of 24% and 23%, respectively, presumably secondary to the increased flow. Similar increases in vessel diameter have also been reported by Bosch et al. (2009) during a climb of Muztagh Ata. In the setting of increased flow and vascular dilation, any sudden increase in vascular pressure due to coughing or straining may cause microvascular rupture. Such additional stresses may not be necessary, however, as Sakaguchi and Yurugi (1983) have produced retinal hemorrhage with animals in a hypobaric chamber when cough was presumably absent. Another potential etiology is increased shear stress related to changes in blood rheology at high altitude. In their study on Muztagh Ata, Barthelmes et al. (2011) found that increases in hematocrit correlated positively with the number of hemorrhages, leading them to suggest that increased blood viscosity might increase shear stress in dilated vessels with altered endothelial integrity, thereby leading to hemorrhage. Most climbers who experience high altitude retinal hemorrhages are asymptomatic because the hemorrhages typically occur away from the macula. In fact, the only reason they are identified is that systematic attempts were made to examine the retinas of climbers in each of these studies. The hemorrhages are usually multiple, often flame-shaped, and adjacent to a vessel (Figure 23.2). Although lack of symptoms is the norm with high altitude retinal hemorrhage, painless vision loss may occur if hemorrhages involve the macular region (Ho et al. 2011; Wiedman and Tabin 1999). When identified, HAR can be graded on a scale from 1 (mild) to 4 (severe) based on the degree of retinal vein dilation and the number and location of retinal hemorrhages (Wiedman and Tabin 1999). Figure 23.2High altitude retinal hemorrhages. Panels A and B depict images from separate climbers on an expedition to Muztagh Ata (7546 m) obtained using digital fundus photography. Image A was taken at 6865 m and demonstrates a single large flame-shaped hemorrhage (black arrows), while image B was taken at 4497 m following descent from higher elevation and depicts a large hemorrhage and two smaller ones. (Images courtesy of Martina M. Bosch, Daniel Barthelmes, and the Muztagh Ata Medical Research Team.) In many cases, high altitude retinal hemorrhages resolve without permanent sequelae. Barthelmes et al. (2011) for example, examined climbers 4.5 months after the Muztagh Ata expedition and found no evidence of persistent pathology. Other, smaller studies, however, have documented residual problems such as persistent visual field constriction (Ho et al. 2011; Wiedman 1975), persistent scotomas (McFadden et al. 1981), or supra- and infradisc nerve fiber layer defects (Ho et al. 2011) that could be related to the retinal hemorrhages themselves or other concurrent pathologies such as vessel occlusion (Bosch et al. 2012). Individuals who develop vision loss at high altitude should descend as soon as feasible, particularly if both eyes are involved. Individuals should be accompanied on descent in the event the visual deficits worsen and markedly impair sight. Consultation with an ophthalmologist is indicated if the visual disturbance fails to resolve within a week of descent (Bosch et al. 2012). While climbers with symptomatic retinopathy have returned to summit peaks over 8000 m (Mader and Tabin 2003), the safety of reascent to high altitude after retinal hemorrhage has not been systematically studied and remains a matter of debate. Some have argued that because retinal hemorrhages are transient in nature, so they should not preclude future ascents (Bosch et al. 2012), but others claim that macular hemorrhages or any retinopathy associated with vision changes should be viewed as a contraindication to further ascent (Mader and Tabin 2003). The cornea receives the bulk of its oxygen supply by diffusion directly from the environment with only a small proportion coming as a result of diffusion from the aqueous humor of the anterior chamber (Klyce 1981). As a result, when ambient oxygen tensions decrease at high altitude, corneal oxygen supply decreases leading, in turn, to increased corneal thickness. In their study of climbers on Muztagh Ata (7546 m), for example, Bosch et al. (2010a) found that central corneal thickness increased by 13% upon ascent, but decreased rapidly following descent. Similarly, Morris et al. (2007) reported an increase in central corneal thickness from 543 µm at sea level to 561 µm on the first day at 5200 m, noting further increases over time at the same elevation and significant inter individual variability in the observed responses. Changes in corneal thickness can occur with more modest ascents to elevations as low as 2800 m (Karakucuk et al. 2012). The mechanism for this change is not clear, but may relate to an increase in anaerobic metabolism in the cornea in response to hypoxia, which leads to increased lactate concentrations in the corneal stroma and an influx of water due to altered osmotic forces. Coupled with reduced activity of the endothelial pumps, this leads to water accumulation and the subsequent corneal thickening. In their study on Muztagh Ata, Bosch et al. (2010a) did find that those individuals who undertook a slower ascent profile and had higher oxygen saturations had less thickening of the cornea, suggesting that systemic delivery of oxygen to the anterior chamber and diffusion to the cornea may provide protection against the processes described above at high altitude. The observed increases in corneal thickness do not appear to have any effect on visual acuity in normal individuals or those with myopia (Bosch et al. 2010a) but, as discussed further, may have adverse effects on individuals who have undergone refractive surgery. Although there are reports of safe contact lens use at the extremes of altitude (Clark and Duff 1976) the risk of infectious complications in this environment has not been well studied. Given that the bulk of corneal oxygen supply occurs as a result of diffusion from the environment and that corneal hypoxia is exacerbated during sleep in hypoxia, use of extended wear contact lenses at high altitude is generally not recommended due to an increased risk of microbial keratitis and ulcerative keratitis (corneal ulceration). While daily disposable or soft/hard lenses that are removed at night improve oxygen supply to the cornea and have been shown to be associated with decreased infectious risk at low elevation (Buehler et al. 1992), frequent handling of these types of lenses, particularly in less hygienic environments often experienced on field expeditions, may still increase the risk of microbial keratitis. Lenses and their cleaning solution may also freeze if left exposed to cold temperatures overnight (Butler and Chalfin 2017). Contact lenses wearers should travel with a back-up pair of glasses as well as fluoroquinolone eye drops to use in the event of corneal infection. Disposable extended wear lenses can be kept in for up to one week but should be removed after that time and replaced with another pair after a night without lenses (Butler and Chalfin 2017). Care should be taken to protect the lenses and cleaning solution from freezing. Extended-wear contact lens users who develop eye pain should remove the lenses, initiate treatment with the fluoroquinolone eye drops, and strongly consider evacuating to lower elevation for medical attention, particularly if symptoms persist or worsen, as untreated ulcerative keratitis can progress to permanent vision loss (Butler and Chalfin 2017; Mader and Tabin 2003). The effect of hypobaric hypoxia on intraocular pressure (IOP) remains unclear as various studies have reported an increase, decrease, or no change in this parameter (Bayer et al. 2004; Bosch et al. 2010b; Ersanli et al. 2006; Willmann et al. 2017). The discrepant results may be due to a variety of factors, such as failure to correct for changes in corneal thickness, differences in measurement techniques, altitudes attained, and confounding effects of exercise and temperature. Somner et al. (2007) corrected for many of these issues and documented a statistically significant, but clinically insignificant, increase in IOP from 11.4 ± 3 mmHg at sea level to 12.4 ± 3.2 mmHg following ascent 5200 m. IOP declined over subsequent days at the same elevation, falling below sea level values by day 7. The observed changes bore no relationship to the development of AMS or high altitude retinopathy. In another study, Willmann et al. (2017) corrected for increases in corneal thickness and found that IOP actually decreased by day 3 at 4559 m. Like Somner et al., they also found no correlation with AMS or other clinical parameters including heart rate and oxygen saturation. There is no evidence that high altitude exposure worsens open angle glaucoma or provokes episodes of acute narrow angle glaucoma, although the hypoxia at high altitude could increase optic nerve damage in patients with elevated IOP at the time of their sojourn. If taken for acute altitude illness prophylaxis, acetazolamide has the added benefit of decreasing intraocular fluid production and thereby limiting increases in IOP (Mader and Tabin 2003). Intraocular gas injection is commonly used in retinal surgery procedures to address issues such as retinal detachment, macular hole, or proliferative diabetic retinopathy. Depending on the type of gas used (e.g., sulfur hexafluoride or perfluoropropane), intraocular gas may remain in the intraocular space for many weeks before being gradually replaced by fluid. A long-standing concern is that the decrease in barometric pressure following ascent will cause these bubbles to expand according to the principles of Boyle’s law, leading to an increase in intraocular pressure that, in turn, may cause significant complications such as central retinal artery occlusion (Fang and Huang 2002). For these reasons, it is generally recommended that patients who have undergone such surgery avoid air travel or travel to high altitude until the gas collections have resolved (Mader and Tabin 2003). The rate of gas expansion and risk of complications may vary as a function of the rate of ascent (air travel vs. slower ascent by hiking, for example), the altitude at which the retinal surgery was performed, the type, concentration, and intraocular volume of gas, and the adequacy of ocular compensatory mechanisms such as scleral expansion or drainage of fluid from the anterior chamber (Silvanus et al. 2008). Levasseur and Rahhal (2013), for example, have shown that patients who traveled by land, rather than airplane, to elevations as high as 1187 m at a rate of 9 m min−1 within one day of vitrectomy with intraocular gas saw small rises in IOP, with mean values remaining within a safe range and no evidence of complications. This study involved ascent to only a very mild elevation, however, and given the large number of variables that affect the risk of gas expansion and the potentially severe consequences of any complications, the most prudent approach is to avoid any significant gain in elevation following vitrectomy with intraocular gas collection until cleared by an ophthalmologist. High altitude exposure increases tear film osmolarity and reduces tear film break-up time (Willmann et al. 2014). Together with environmental conditions commonly seen at high altitude, including decreased humidity, wind, and glare, this may predispose to development of dry eye syndrome. Often seen at low altitude due to decreased tear production and alterations in the ocular surface and lacrimal gland function, symptoms of dry eye syndrome vary based on the degree of dryness and include minor irritation, burning, foreign body sensation, light sensitivity, and blurred vision (Mader and Tabin 2003). In all but the most severe cases, topical tear substitute eye drops and lubricating ointments are sufficient to treat dry eye symptoms, although important differences exist between the available preparations. Artificial tear preparations can be reused after opening because they contain preservatives, although the preservatives, themselves, can cause irritation. Preservative-free preparations avoid this problem but are prone to bacterial contamination and can only be used for 24 hours after opening. Wrap-around glasses or goggles may prevent dry eye syndrome by shielding the eye from wind, dust, and ultraviolet light and potentially increase the humidity around the cornea (Mader and Tabin 2003). Changes in tear film osmolarity and break-up time are reversible with descent (Willmann et al. 2014), and as a result, individuals not otherwise predisposed to this condition should see resolution of dry eye symptoms upon return to low elevation. Just as it causes sunburn, excessive ultraviolet (UV) light exposure can cause damage to the epithelial layer of the inadequately protected eye. At high altitude, the risk of such damage is significantly increased and the time frame over which it develops is significantly shortened as a result of the significant increase in UV exposure as one moves higher in elevation—a 4% increase for every 300 m gain in elevation—particularly when traveling in snow-covered terrain. This problem is described in greater detail in Chapter 27 on other environmental illnesses. In general, the hypoxia at high altitude does not affect visual acuity or pre-existing refractive errors, although those individuals who rely on glasses or contact lenses do need to consider the effect of the wilderness environment on their ability to maintain ocular hygiene and prevent significant ultraviolet exposure. Concern exists, however, for those individuals who have undergone surgery for repair of refractive errors and plan to travel to very high altitude. Three procedures have been used to correct refractive errors and obviate the need for glasses and contact lenses. In radial keratotomy (RK), four to eight radial incisions are made at 90% depth in the periphery of the cornea, leading to a change in the refractive error of the lens. In photorefractive keratectomy (PRK), a laser is used to reduce corneal curvature and create refractive changes directly on the corneal surface, while in laser-assisted in situ keratomileusis (LASIK), a thin flap is created in the cornea and lifted so that an excimer laser can then reshape corneal stroma (Mader and Tabin 2003). Because each procedure alters the normal structure of the cornea and hypobaric hypoxia induces corneal changes, as noted, concern exists regarding the risk of visual complications in individuals who travel to extremely high elevations following these procedures. Several studies have shown that RK corneas are susceptible to problems at high altitude (Mader et al. 1996; Mader and White 1995). Mader et al. (1996), for example, studied six subjects with RK, six with PRK, and nine with myopia over a three-day period at 4300 m and noted no change in refraction in the subjects with myopia or PRK, but significant changes in refraction in those who underwent RK. These changes occur because the peripheral corneal incisions made in RK weaken the cornea in such a way that hypoxia causes preferential expansion in the periphery of the cornea that, in turn, causes flattening of the central cornea and subsequent hyperopic (far-sighted) shift (Mader and Tabin 2003; Winkle et al. 1998). Of note, the changes generally occur 24 hours or more after high altitude exposure and may be worsened during sleep when the eyelids are closed and the cornea is under increased hypoxic stress. This may result in a false sense of security for a climber with RK corneas who arrives at high altitude and initially has intact vision. LASIK corneas are also at risk for vision changes at high altitude. Dimmig and Tabin (2003), for example, examined 12 LASIK-treated eyes in six climbers ascending Mount Everest, all of whom had 20/20 or better vision at sea level. Vision was maintained at 5365 m. One of the six climbers noted blurred vision above base camp, while two climbers noted problems at 8200 m and 8600 m, respectively. In all cases, the problem improved with descent. Similarly, Boes et al. (2001) documented decreased visual acuity in two climbers on Aconcagua (6963 m) that worsened with increasing altitude and declining oxygen saturation, but resolved with descent, although in one of the climbers, full resolution required several weeks. More systematic data regarding the precise magnitude of changes are lacking at this time. Aaron et al. (2012) have shown an absence of significant changes in contrast visual acuity and refractive error in LASIK-treated individuals with exposure to 10,668 m for a period of 30 minutes, but no studies have examined the extent of such changes during more prolonged exposures as may be experienced on mountaineering expeditions, for example. Of the three procedures, only PRK is thought to be without problems at high altitude as hypoxia causes uniform thickening of the PRK corneas, which preserves the shape of the corneal surface and, as a result, does not cause myopic shifts (Mader et al. 1996; Mader and White 2012). No studies have systematically assessed the effect of hypoxia on vision in patients who have undergone LASEK or EPI-LASIK procedures (Mader and White 2012). There is an element of unpredictability to the vision changes seen following RK and LASIK at altitude, as many factors including cold, drying of the cornea, duration and extent of high altitude exposure, the degree of refractive error remaining after surgery, and time since surgery may affect the likelihood of any problems. For this reason, all climbers who have undergone these procedures should be prepared for problems at high altitude and should travel with back-up pairs of eyeglasses with plus lenses. Because it is hard to predict how much lens power will be needed ahead of time, it may be necessary to travel with multiple pairs of glasses (Mader and Tabin 2003). In addition to the cerebral arterial or venous thromboembolic events described above, a variety of other neurologic disorders have been described at high altitude, which are distinct from HACE. Many of these disorders have been reviewed elsewhere (Basnyat et al. 2004; Baumgartner et al. 2007) and are considered briefly next. While it is tempting to attribute all headaches at high altitude to acute altitude illness, it is important to consider other potential causes in the differential diagnosis. Given how common they are at low elevation, migraine headaches can be expected to occur at altitude in people with a known history of the problem. In addition to anecdotal reports suggesting high altitude may be a trigger for attacks (Hackett 2001), several studies suggest, but do not prove, that hypoxia predisposes to migraines. In a study by Arngrim et al. (2016), for example, eight of 15 patients with a history of migraine with aura developed headache when exposed to 180 minutes of normobaric hypoxia sufficient to lower the SpO2 to 70–75%, compared to only one of 14 people with no headache history exposed to the same conditions. Broessner et al. (2016) exposed healthy volunteers with no history of migraines to a simulated elevation of 4500 m and noted development of headaches that met the criteria for migraines according to the International Classification of Headache Disorders in 8% and 15% of subjects after 6 and 12 hours of exposure, respectively. Beyond the possible link between hypoxia and migraine headaches, other, older reports suggest the severity of migraine attacks may be worse at altitude and may be accompanied by transient, focal neurological defects (Jenzer and Bartsch 1993; Murdoch 1995a). Jenzer and Bärtsch (1993) described a healthy 25-year-old mountaineer with known migraines at low altitude who experienced a very severe headache together with some aphasia and sensory impairment of his tongue and right hand following a climb above 8000 m. Four years later, he had another episode, similar in many ways to the first. Both episodes lasted a several hours and subsequent neurological tests, including computed tomography (CT) scans, were all negative. Distinguishing migraine headache from AMS at high altitude may be challenging, as demonstrated by a recent case from Karle and Auerbach (2014) in which a 36-year-old with a history of migraine developed a headache while trekking to Everest base camp that was attributed to a migraine, but subsequently was found to have acute altitude illness and required evacuation to lower elevation. In general, the presence of aura—an uncommon feature of headaches in AMS—similarity of symptoms to those of migraine headaches experienced at sea level, and the presence of focal neurologic deficits should prompt consideration of migraine headache. At the same time, when headaches are attributed to migraine but fail to resolve with appropriate interventions, consideration must be given to the possibility of AMS. Several reports have documented problems related to vascular abnormalities. Litch et al. (1997), for example, reported an individual who developed spontaneous subarachnoid hemorrhage while descending from a trek to 4700 m in Nepal, while Hackett (2001) described three patients at altitude with neurological defects, two of whom were subsequently shown to have cerebral arteriovenous malformations and the third, an aneurysm. Rupture of such vascular abnormalities should be easy to distinguish from AMS, as the presentation—rapid onset of a maximal severity of headache often with associated nausea, meningismus or mental status changes—is very distinct from the typical headache in AMS or the more gradual onset of mental status changes in HACE. However, pressure from a large aneurysm or a small leak from a sentinel hemorrhage may cause more subtle symptoms that can be harder to differentiate from altitude illness. It is unclear whether ascent to altitude is a risk factor for subarachnoid hemorrhage in those with aneurysms or arteriovenous malformations. Jehle et al. (1994) have shown evidence of an association between the incidence of subarachnoid hemorrhage and changes in barometric pressure associated with changes in weather, but no studies have established a relationship with the larger barometric pressure changes seen with ascent to high altitude. From a theoretical standpoint, hypoxemia-induced increases in cerebral blood flow or increased systemic blood pressure due to sympathetic nervous system activation could serve as a trigger for vascular rupture, but there is no systematic evidence of this phenomenon. Seizures have been described in men and women over a wide age range ascending to a broad range of altitudes from 2500 m to 7600 m, with generalized tonic-clonic seizures being more common than partial seizures (Maa 2011). They are not typically seen as part of AMS or HACE and may occur in the absence of obvious precipitating events (Hennis et al. 2014; Kupper and Classen 2002). Despite these reports, the overall incidence of seizures at high altitude appears to be low. Kupper, for example has described an analysis of 2730 alpine helicopter rescue operations, in which only 0.3% of patients suffered a seizure and all such events occurred in patients with a prior history of neurologic issues (Kupper 2014). The etiology of these events is not clear but may involve a lowering of the seizure threshold as a result of direct effects of hypobaric hypoxia, sleep deprivation, hyperventilation, and cerebral edema. A detailed approach to patients with seizures at high altitude has been reviewed elsewhere (Maa 2011). The majority of seizures are self-limited and field management should focus on protecting the patient by clearing the area around the patient, protecting the head from striking hard objects, and keeping the mouth clear of material. Seizures that do not cease spontaneously should be managed as done at sea level with benzodiazepines being the first-line treatment if available. Given the potential role of hypobaric hypoxia and periodic breathing in triggering seizures, consideration can be given to initiating supplemental oxygen and acetazolamide. Because seizures may occur in clusters and repeated events pose a risk to the patient, evacuation to lower elevation and/or a medical facility should also be considered, where further work-up can be pursued in those with no known seizure history (Maa 2011). Although prospective studies are lacking, future travel to high altitude is likely feasible if such evaluation fails to reveal a predisposing neurologic condition (Kupper 2014). Shlim et al. (1991) reported three patients who were asymptomatic at low altitude, developed neurologic symptoms at altitude, and were later found to have brain tumors, while Hackett (2000) reported a case of a man who suffered diplopia and ataxia on two occasions when he ascended from sea level to 4000 m and was later diagnosed with a left frontal subarachnoid cyst. The reason for symptom onset at high altitude may relate to subclinical brain edema at high altitude, although several magnetic resonance imaging (MRI) studies suggest that increases in brain volume may not actually be responsible (Fischer et al. 2004; Morocz et al. 2001). Rather than diffuse cerebral edema, it may be that hypoxia induces perilesional edema that, in turn, causes symptoms (Baumgartner et al. 2007). Unfortunately, the frequency of these events is too small to draw firm conclusions. Similarly, there is no evidence regarding whether prior traumatic brain injury predisposes to problems at high altitude. A variety of transient neurologic deficits have been described at high altitude. For each type of deficit, the overall number of reports is low, making it difficult to draw conclusions about incidence, risk factors, and management.
Introduction
Thromboembolic Disease
Types of thromboembolic events
Cerebrovascular Thromboembolism
Other Sites of Thromboembolism
Risk for thromboembolic disease at high altitude
Prevention and management of thromboembolism
Ophthalmologic Problems
High altitude retinopathy (HAR)
Incidence
Pathophysiology
Clinical Features
Management
Corneal issues
Corneal Thickness
Infectious Complications in Wearers of Contact Lenses
Intraocular pressure
Intraocular gas following retinal surgery
Dry eye syndrome
Ultraviolet keratitis
Surgery for refractive errors
Neurologic Conditions Other Than Acute Altitude Illness
Other causes of headache
Migraines
Ruptured Vascular Abnormalities
Seizures
Space-occupying lesions
Transient neurologic deficits
Global Amnesia
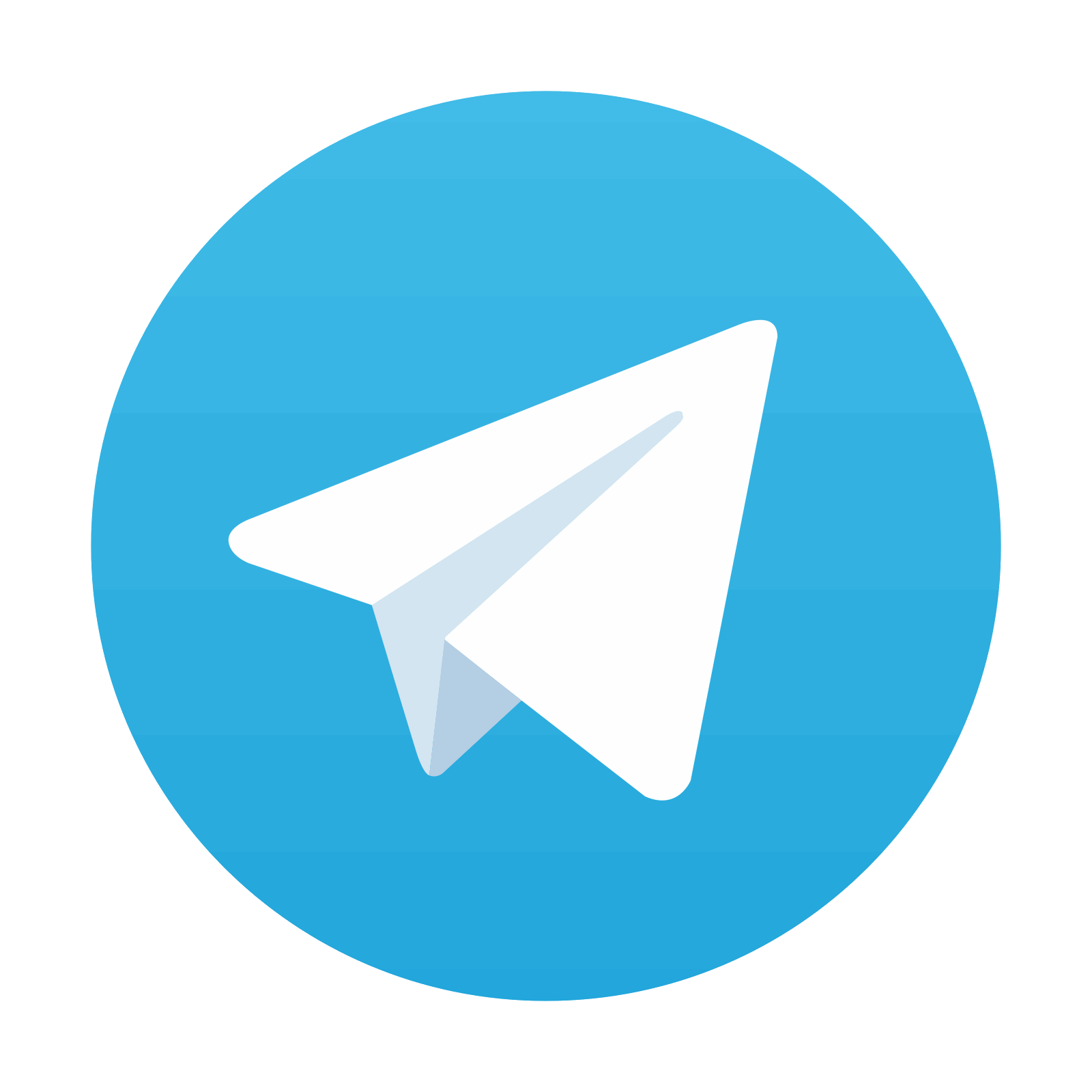
Stay updated, free articles. Join our Telegram channel

Full access? Get Clinical Tree
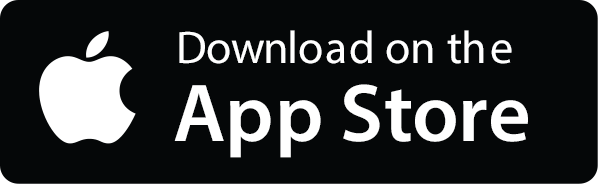
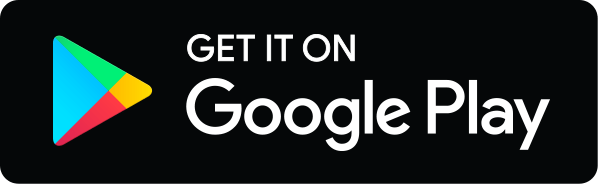