Abstract
The splanchnic circulation receives over 25% of the cardiac output and contains a similar percentage of the total blood volume under normal conditions. Thus the splanchnic circulation can act as a site of regulation of distribution of cardiac output and also as a blood reservoir. Multiple regulatory pathways are involved in the distribution of the splanchnic circulation. This chapter focuses on the intestinal and hepatic circulations because of their functional importance and in particular, because they are sites of dysregulation during pathologic conditions and surgical stresses, such as cardiopulmonary bypass. A review of the anatomy and physiology of both the intestinal and hepatic circulation is presented followed by the effects of cardiopulmonary bypass on this circulation. Splanchnic complications associated with cardiac surgical procedures will be reviewed. These include postcoarctectomy syndrome, protein-losing enteropathy, intestinal ischemia, necrotizing enterocolitis, ischemic hepatitis, acute hepatic failure, chronic hepatic dysfunction and cirrhosis, and pancreatitis.
Key Words
Splanchnic circulation, cardiopulmonary bypass, protein-losing enteropathy, intestinal ischemia, necrotizing enterocolitis, postcoarctectomy syndrome, hepatic complications
The term splanchnic circulation refers to all blood flow originating from the celiac, superior mesenteric, and inferior mesenteric arteries, which is widely distributed to all abdominal viscera. The splanchnic circulation receives 25% of the cardiac output and therefore holds a similar percentage of the total blood volume under normal conditions. Thus the splanchnic circulation can act as a site of cardiac output regulation and also as a blood reservoir ( Fig. 15.1 ). Multiple regulatory pathways are involved in the distribution of the splanchnic circulation. This chapter will be limited to the intestinal and hepatic circulations because of their functional importance and particularly because they are sites of dysregulation during pathologic conditions and surgical stresses, such as cardiopulmonary bypass (CPB).

Basic Principles of the Splanchnic Circulation
Intestinal Circulation
Anatomy.
The named mesenteric arteries branch into multiple serosal arteries, which provide intestinal blood flow. These serosal arteries course from the mesenteric to the antimesenteric surface of the intestine, where they penetrate the muscular layer, giving rise to the submucosal and mucosal vasculature. The terminal arteriolar branches supply blood flow to the intestinal villus. This circulatory arrangement is of particular interest because of the proximity of the arteriole and the venule in the villus, which creates a considerable countercurrent exchange of oxygen. Thus an oxygen gradient is produced between the base and tip of the villus ( Fig. 15.2 ). The potential for arteriovenous shunting and the complex plexus of capillaries contribute to making the distal portions of the villus prone to ischemia. The lability of flow at the villous tip is readily evident in studies examining the progression of mucosal injury to a graded ischemic insult.

Physiology.
The balance of systemic constrictor and local dilatory mechanisms regulates intestinal blood flow. Systemic activation of sympathetic nerves results in vasoconstriction of the intestinal vasculature and decreased splanchnic blood flow. Catecholamines and other circulating factors, such as angiotensin II, also contribute to the constrictor response. Substances released from the intrinsic nonadrenergic noncholinergic (NANC) nerves largely mediate the dilatory mechanisms. These include neurotransmitters, adenosine, substance P, and nitric oxide. All of these factors, with the probable exception of substance P, may be released by nonneural tissue. The constrictor mechanisms act globally on the entire splanchnic circulation, whereas dilators work locally to affect alterations in the distribution of blood flow, particularly between the mucosal and muscular portions of the intestine (see Fig. 15.2 ). Low concentrations of nitric oxide may also have other potent effects, such as antithrombosis and antiadhesion of leukocytes. Hypothermia, a pertinent factor in patients receiving CPB, may also decrease leukocyte adhesion and help ameliorate ischemia/reperfusion injury.
Unlike the circulating catecholamines and angiotensin II, the constrictor effects of endothelin most likely act in a paracrine fashion. Endothelin-1 and endothelin-3 mediate both vasoconstriction and vasodilation in the intestinal blood vessels and cause contraction of intestinal smooth muscle. Endothelin-A receptors mediate the contractile response, whereas the endothelin-B receptors mediate both contraction and relaxation, the latter via coupling to nitric oxide synthase. Upregulation of the endothelin after CPB may be a factor in decreased intestinal perfusion and villus injury after bypass.
Hepatic Circulation
Anatomy.
The terminal portions of the hepatic circulation are arranged in a series of repeating functional units called acini or lobules, depending on whether the portal triad or the central vein is used as the anatomic reference point. The basic unit is approximately 0.5 mm in diameter and consists of a portal venular inflow, a network of sinusoids, and a hepatic venular outflow. Blood flow through these subunits is fairly uniform during normal conditions.
The portal vein provides approximately 75% of the blood supply to the liver. This volume is equal to the total blood flow of the other splanchnic viscera, because it is formed from their venous effluent. Portal vein perfusion pressures are low (approximately 10 mm Hg), and portal blood is relatively oxygen-poor. The low perfusion pressure requires a low vascular resistance to accommodate the relatively high portal venous blood volume. In contrast, the hepatic artery, which supplies the remaining 25% of total hepatic blood flow, is oxygen-rich (mimics the partial pressure in systemic arterial blood) and perfuses the liver at systemic arterial pressure. Because the hepatic artery flow joins the portal venous system in the portal venules or directly in the hepatic sinusoid, arterial resistance must remain high to maintain a sufficient pressure drop to prevent retrograde flow through the portal vein.
Physiology
Regulation of Hepatic Blood Flow.
Blood flow is unidirectional from the portal to the central vein within the sinusoidal beds of the hepatic lobule. Because the oxygen content is low, a significant oxygen gradient is established. This situation results in relative centrilobular hypoxia, even under normal conditions. During conditions of low flow, this gradient becomes greater, and overt ischemia can develop in the centrilobular areas. The tendency toward ischemia may produce centrilobular necrosis, the hallmark of low-flow injury in the liver.
The regulation of total portal blood flow lies primarily upstream in the resistance vessels of the intestine during normal conditions. Although intrahepatic or posthepatic diseases have relatively little influence on the total volume of portal blood flow, they can have great significance in the distribution of blood flow and portal pressure. In patients with chronic disease (i.e., cirrhosis), severe disruption of acinar blood flow results in portal hypertension. This leads to the development of extrahepatic shunts. Under these conditions a substantial portion of portal blood can reenter the circulation without first flowing through the liver. Total liver blood flow may not be affected before the development of extrahepatic shunts, which develop over days to weeks. However, heterogeneous distribution of blood flow can exist earlier as a result of intrahepatic shunts, which may impair liver function. In addition to shunting blood, contraction of intrahepatic vessels may cause substantial pooling of blood within the liver and mesenteric veins, resulting in a loss of circulating blood volume.
Systemic Venous Hypertension and Increased Outflow (Downstream) Pressure of the Liver.
The portal circulation, with low perfusion pressure, is sensitive to elevation of outflow (downstream) pressures. The site of increased pressure may occur anywhere along the pathway from the sinusoid to the hepatic vein to the right heart. Three cell types line the sinusoid: the endothelial cell, the Kupffer cell, and the hepatic stellate cell. All three cell types are capable of secreting vasoactive substances. Among the various vasoactive substances, endothelin-1 is a prominent vasoconstrictor of endothelial cells and hepatic stellate cells, leading to contraction of the hepatic sinusoid during shock. In addition, sinusoidal contraction resulting in increased portal venous pressures has been associated with CPB. Cytokines, such as interleukin-1 and tumor necrosis factor; arachidonic acid metabolites, including the thromboxanes, leukotrienes, and platelet activation factor; neutrophil products, such as oxygen free radicals and peptidases; and endothelin-1 seem to mediate this process. Sinusoidal perfusion failure is associated with the release of oxygen free radicals, cholestasis, enzyme release from the liver, and hepatocyte necrosis.
Systemic venous pressure elevation represents another cause of increased downstream pressure for the hepatic microcirculation. This increase in systemic venous pressure may arise from a variety of derangements, such as clot in the great veins, right heart failure, tricuspid valve insufficiency, or pulmonary hypertension. A failing Fontan circulation in the patient with a univentricular heart represents a specific cause of systemic venous hypertension. Because the increase in downstream pressure originates in the heart or great vessels, there is a diffuse increase in systemic capillary pressure in addition to the increase in hepatic vein and sinusoidal pressure. Therefore the manifestations are more generalized with hepatomegaly, ascites, pleural effusions, and edema.
Liver dysfunction secondary to high right-sided venous pressure is associated with decreased hepatic blood flow and oxygen delivery. The increased hepatic venous pressure produces pressure atrophy of hepatocytes and perisinusoidal edema. These effects summate to create the classic pathologic picture of centrilobular necrosis.
Hypotension and the Hepatic Artery Buffer Response.
Even mild hypotension compromises splanchnic and therefore portal blood flow. However, total liver blood flow and hepatic oxygen delivery are preserved until hypotension becomes severe. Oxygen delivery is maintained as a result of a compensatory increase in hepatic artery flow. This compensation is termed the hepatic artery buffer response . Besides hypotension, any condition that results in a decrease in portal blood flow, such as CPB, will invoke a hepatic artery buffer response. Although this arterial buffer response preserves total oxygen delivery, the response does not appear to be regulated by the metabolic needs of the hepatic parenchyma. Rather, hepatic arterial resistance is sensitive to changes in the volume of the portal circulation. A vasodilator substance such as adenosine is likely released from hepatocytes at a constant rate. This substance is washed out of the interstitium, so that it does not accumulate, when portal flow is normal or elevated. When portal blood flow decreases, the vasodilator substance accumulates in the vicinity of the hepatic arterial resistance vessels, causing vasodilation and increased flow in the hepatic artery vascular bed. Classic arterial autoregulation can also be explained by this mechanism. Increased hepatic artery blood flow, for example, will cause faster washout of adenosine from the interstitium, with resultant arterial vasoconstriction. Other vasoactive agents such as nitric oxide and angiotensin II may also contribute to regulation of total liver blood flow in this setting.
Effects of Cardiopulmonary Bypass on the Splanchnic Circulation
Overview
A number of factors may play a role in the regulation of hepatic and splanchnic blood flow and organ function during CPB for congenital heart surgery ( Box 15.1 ). A decrease in splanchnic perfusion, detectable via new noninvasive technologies, is associated with early postoperative life-threatening complications. Bypass technique, duration, and mediator release affect splanchnic perfusion.
↑ Extracellular fluid
↑ Exchangeable Na + , ↓ K +
↓ O 2 delivery
↑ O 2 consumption
↑ Hepatic venous lactate and pyruvate
Hyperglycemia (hypothermic CPB, 24°C-28°C)
CPB, Cardiopulmonary bypass; K + , potassium ions; Na + , sodium ions; O 2 , oxygen; ↓ , decreases; ↑ , increases.
Physiologic Factors
Bypass flow rate is the major determinant of portal and hepatic arterial blood flow during CPB. Failure to maintain normotension or high flow rates during CPB may jeopardize hepatic blood flow. Normothermic CPB causes a 50% decrease in hepatic blood flow during nonpulsatile bypass in dogs perfused at a lower than normal blood pressure. Under these experimental conditions, hepatic blood flow decreases less than portal venous blood flow. Ostensibly the hepatic artery buffer maintains hepatic flow during low CPB pump flow by increasing the contribution of hepatic arterial blood flow at a time when portal venous flow decreases. This is true for any body temperature or perfusion technique. Conversely, portal venous flow improves more consistently by use of a higher flow rate, in keeping with previous studies showing the sensitivity of portal flow during conditions of decreased cardiac output.
The presence of a potent vasoconstrictor, especially in the setting of diminished cardiac output, during or after cardiac surgery may place the splanchnic circulation at risk for ischemia. Vasoactive mediators that may be released during cardiac surgery include angiotensin II, vasopressin, thromboxane A 2 and B 2 , and catecholamines, including epinephrine and norepinephrine and the endothelins. All these substances may cause vasoconstriction, a potentially catastrophic event in the face of an already diminished oxygen delivery. Clinically the presence of the hepatic buffer to maintain total hepatic flow when portal venous flow falls and the maintenance of portal flow during high-flow CPB may account for the relatively low incidence of gastrointestinal complications after bypass.
The effect of CPB pulsatility on hepatic blood flow depends on the CPB flow rate and temperature. Although some investigators show better preservation of hepatic blood flow by pulsatile bypass perfusion, Mathie did not observe any change in hepatic flow as long as the CPB flow rate was maintained at a high level. Pulsatile CPB decreases systemic vascular resistance and increases hepatic blood flow during hypothermia. The beneficial effects of pulsatile flow may be apparent only when low-flow CPB is used. Angiotensin II levels increase during and after CPB in dogs and adults, an increase that may be accentuated by nonpulsatile bypass and low perfusion rates. The increase in angiotensin II levels during CPB may depend on the baseline preoperative angiotensin level, because angiotensin II levels actually decreased from very high preoperative levels following CPB in children.
Changes in both PaO 2 and PaCO 2 have been shown to affect hepatic blood flow. Hypoxemia causes a mild constriction of the hepatic artery, an effect probably mediated by sympathetic stimulation. Hyperoxia does not appear to alter hepatic blood flow. Acidosis constricts hepatic artery flow but simultaneously dilates portal flow. Total hepatic blood flow will either increase or decrease depending on whether the constrictor or dilator effects predominate. Hypocapnia decreases both hepatic artery and portal blood flow in dogs. There are no data on the effect of hypocapnia on hepatic blood flow in infants undergoing congenital heart surgery; however, adults treated with hypocapnia for trauma head injury have been shown to have no decrease in hepatic blood flow.
A general decrease in core temperature unrelated to CPB has little effect on hepatic arterial blood flow but increases intestinal and therefore portal blood flow. When flow is kept constant during CPB, the same increase in portal and intestinal blood flow in response to a decrease in core temperature would be expected. Lowering the core temperature from 37°C to 28°C decreases oxygen consumption by 50%. However, hypothermia impairs splanchnic oxygen extraction and may have deleterious effects on splanchnic function during hypothermic CPB.
Rewarming after CPB may also affect oxygen delivery. Rewarming is characterized by a decrease in oxygen delivery and an increase in oxygen consumption. This uncoupling of oxygen delivery and consumption may be responsible for the lactic acidosis that occurs after CPB. During rewarming there is a generalized increase in sympathetic nervous system activity, resulting in increased levels of circulating norepinephrine. Selective rewarming of either the spinal cord or the hypothalamus decreases splanchnic oxygen delivery in animal models. Spinal or hypothalamic warming results in increased splanchnic sympathetic nervous system output and thus superior mesenteric artery vasoconstriction. The effects of core rewarming on splanchnic blood flow, mucosal blood flow, and hepatic function have not been fully elucidated.
CPB affects hepatic lactate metabolism. Decreased peripheral oxygen delivery and increased oxygen consumption result in increased lactic acid levels both during and after cardiac bypass. In normal circumstances, hepatic lactate clearance is highly effective, but this clearance capacity is saturated in the presence of very high systemic lactate levels and hepatic blood flow less than 25% of normal. Hepatic venous lactate and pyruvate levels have been shown to increase during the first 30 minutes of CPB. The combination of a rise in both lactate and pyruvate with a relatively constant lactate-pyruvate ratio suggests that there is not only an increase in anaerobic metabolism, but also a possible alteration in the activity of key enzymes such as pyruvate dehydrogenase.
Although prospective data are not available, several retrospective studies reveal that most major gastrointestinal complications occur in CPB patients who experience episodes of hypotension or prolonged shock, particularly when pressor support is required ( Box 15.2 ). Centrilobular hepatocellular necrosis, cholestasis, and intestinal ischemia are perhaps the most common complications, but other disorders such as gastritis, pancreatitis, and intestinal hemorrhage also occur with increased frequency in these critically ill patients.
Hypotension
Prolonged shock
Prolonged cardiopulmonary bypass
Vasopressor support
Postoperative low cardiac output
High right-sided venous pressure
Acidosis
Prematurity
Clinical Conditions
Congenital Malformations
Certain congenital syndromes combine heart disease and intestinal anomalies. The infant with Down syndrome (see Chapter 75 ) may have an intracardiac defect combined with duodenal atresia. Bilious vomiting and less commonly abdominal distention suggest the possibility of bowel obstruction, which is confirmed with the classic double bubble sign on abdominal radiograph. The long-term survival rate for duodenal atresia is 86% with virtually all deaths occurring among patients who also had complex cardiac disease.
Heterotaxy syndrome (HS) refers to embryologic abnormalities in the usual left and right asymmetry and results in complex congenital heart disease (CHD) and abnormalities of abdominal organ anatomy. Although intestinal rotational anomalies are the most common finding, gastric volvulus, preduodenal portal vein, esophageal hiatal hernia, and biliary atresia may also occur. The cardiac disease in this population is very complex. Those with asplenia syndrome (right atrial isomerism) demonstrate atrioventricular canal defect, double-outlet right ventricle, transposition of the great arteries, anomalous pulmonary venous return, and pulmonary stenosis or atresia. Bilateral superior vena cavae are usually present. The subgroup with polysplenia syndrome (left atrial isomerism) usually have the combination of ventricular septal defect, atrial septal defect, and pulmonic stenosis. The inferior vena cava is usually interrupted. Instead, a dilated azygous venous system connects the infradiaphragmatic veins with the superior vena cava. The normal visceral asymmetry is absent in this population (particularly in the asplenia group) so that the liver is midline and the stomach is on the side opposite to the cardiac apex. Thus a chest and abdominal radiograph immediately suggest the diagnosis. Given the complexity of the heart disease and the immune deficiency associated with asplenia/polysplenia, the addition of intestinal obstruction leads to a very high (90%) mortality rate.
Much recent interest has focused on the treatment of patients with HS and intestinal rotational anomalies (IRAs). Forty to 90% of HS patients may have some degree of IRA. There is much debate on whether or not an asymptomatic HS patient should be screened for IRA, or even treated if the condition is discovered. Of note, pediatric surgeons continue to debate this same topic in non-CHD patients as well. Previously mortality rates were reportedly as high as 40%; subsequent advances in pediatric critical care, anesthesia, and cardiac surgical care have vastly improved survival in HS patients. These improved outcomes have lead physicians caring for these children to place more emphasis on treatment of malrotation and to have a desire to avoid the dreaded complication of midgut volvulus. The more interventionist school of thought argues that the known high incidence of malrotation in HS patients warrants screening and prophylactic surgery (the Ladd procedure or intestinal derotation), and that this surgery is much better tolerated in an elective fashion, as opposed to an emergent situation in which volvulus has occurred and bowel ischemia exists. Others have argued that the risk of volvulus is extremely small in HS patients, and that their major morbidities after the Ladd procedure are high. The largest review to date suggests that HS patients have a higher morbidity after the Ladd procedure, and a very small percentage of the patients actually had volvulus. However, several single-center retrospective reviews report no difference in the tolerance of the procedure between HS and non-HS populations and advocate an operative approach in asymptomatic patients with HS once the diagnosis of IRA is made. Other studies have suggested the HS patients with predominately right-sided isomerism have a higher risk of narrowed mesentery and therefore catastrophic volvulus than HS patients with left-sided isomerism, further complicating the decision making.
Until a definitive randomized controlled trial is performed, it is reasonable to take an individualized approach to patients with HS and concern for IRA. Patients with abdominal symptoms (feeding intolerance, emesis, signs of obstruction) should be screened with an upper gastrointestinal series, and a decision made regarding surgery should involve cardiology, anesthesia, and the family. Parents should have the opportunity to give fully informed consent to a prophylactic and possibly high-risk surgical procedure, knowing that although the risk of volvulus might be low, it is a catastrophic event. In the case of HS patients with no abdominal symptoms, routine screening does not appear to be mandatory, although increased vigilance in patients with known right-sided isomerism seems warranted. When the decision not to perform prophylactic surgery is made, extensive counseling regarding the signs and symptoms of volvulus, and what to do should those signs and symptoms occur, is extremely important before the patient leaves the hospital setting.
Alagille syndrome (AGS) frequently involves both CHD and liver disease. This is an autosomal dominant disorder with highly variable expressivity characterized not only by liver and heart abnormalities but also by a characteristic facies, butterfly vertebrae, corneal opacities, renal disease, and intracranial bleeding. AGS is associated with two mutations in the Notch signaling pathway, which determines cell fate early in embryogenesis. Mutations can occur in the JAG1 gene, found in approximately 95% of affected individuals, or in the NOTCH2 gene (1% to 2%). Patients with AGS typically exhibit right heart obstructive lesions ranging from peripheral pulmonic stenosis to tetralogy of Fallot. Cholestasis distinguishes the liver disease in this syndrome, which may progress to liver failure. Liver transplantation may be necessary because of intractable pruritus and failure to thrive. Elevated right heart pressure from peripheral pulmonic stenosis is not a contraindication for liver transplantation.
Splanchnic Complications Associated With Specific Cardiac Operations
Etiology.
The multiple etiologic factors that produce splanchnic complications with cardiac surgery or CPB are poorly understood ( Box 15.3 ). The prevailing hypothesis is that ischemia/reperfusion injury plays a major role. This is supported by the clinical observation that most splanchnic complications occur in the setting of hypotension or overt shock. The involvement of other factors such as microemboli, toxins, infectious agents, and vasopressors is suggested by the fact that complications do not develop in all patients who experience hypotension or shock. These splanchnic complications can occur without a previous episode of hypotension. Likewise, there does not appear to be a specific splanchnic target organ, although the liver and the intestinal tract appear to be the most vulnerable. Table 15.1 presents some of the potential complications associated with certain operative procedures.
Transposition of the great vessels
Hypoplastic left heart syndrome
Tricuspid atresia
Tetralogy of Fallot
Pulmonary atresia
Ventricular septal defect (large left-to-right shunt)
Coarctation of the aorta
Cardiac Pathology/Operation | Splanchnic Complication | Risk Factors (Perioperative) | Reference |
---|---|---|---|
Cardiopulmonary Bypass Group | |||
Hypoplastic left heart syndrome/Norwood | Mesenteric ischemia | Hypotension | |
Cardiac arrest within 48 h of mesenteric ischemia | |||
Tricuspid atresia/Fontan | Acute hepatic failure | Elevated CVP | |
TOF/repair | Low cardiac output | ||
AV canal/repair | |||
Pulmonary atresia/repair and valve | |||
Single ventricle/modified Fontan | Acute hepatic failure | Low cardiac output | |
Tricuspid atresia/modified Fontan | Low urinary output | ||
Mitral atresia/modified Fontan | |||
VSD/repair | Perforated peptic ulcer | Hypotension | |
TOF/repair | UGI bleed | Hypoxia | |
ASD/repair | |||
VSD/repair | UGI bleeding—gastric | None reported | |
ASD/repair | Stress ulceration | ||
Transposition/Mustard | Protein-losing enteropathy | SVC obstruction | |
Tricuspid atresia/Fontan | SVC/IVC obstruction | ||
Non–Cardiopulmonary Bypass Group | |||
Coarctation of aorta | Peptic ulcers | Hemoconcentration | |
Subclavian flap | |||
Coarctation | Perforated peptic ulcer | None | |
Subclavian flap | |||
Coarctation/end-to-end anastomosis | Renal failure | Associated cardiac anomalies | |
Nonoperated Group | |||
Hypoplastic left heart | Mesenteric ischemia | Low birth weight | |
PDA + CHF | Major associated anomalies |
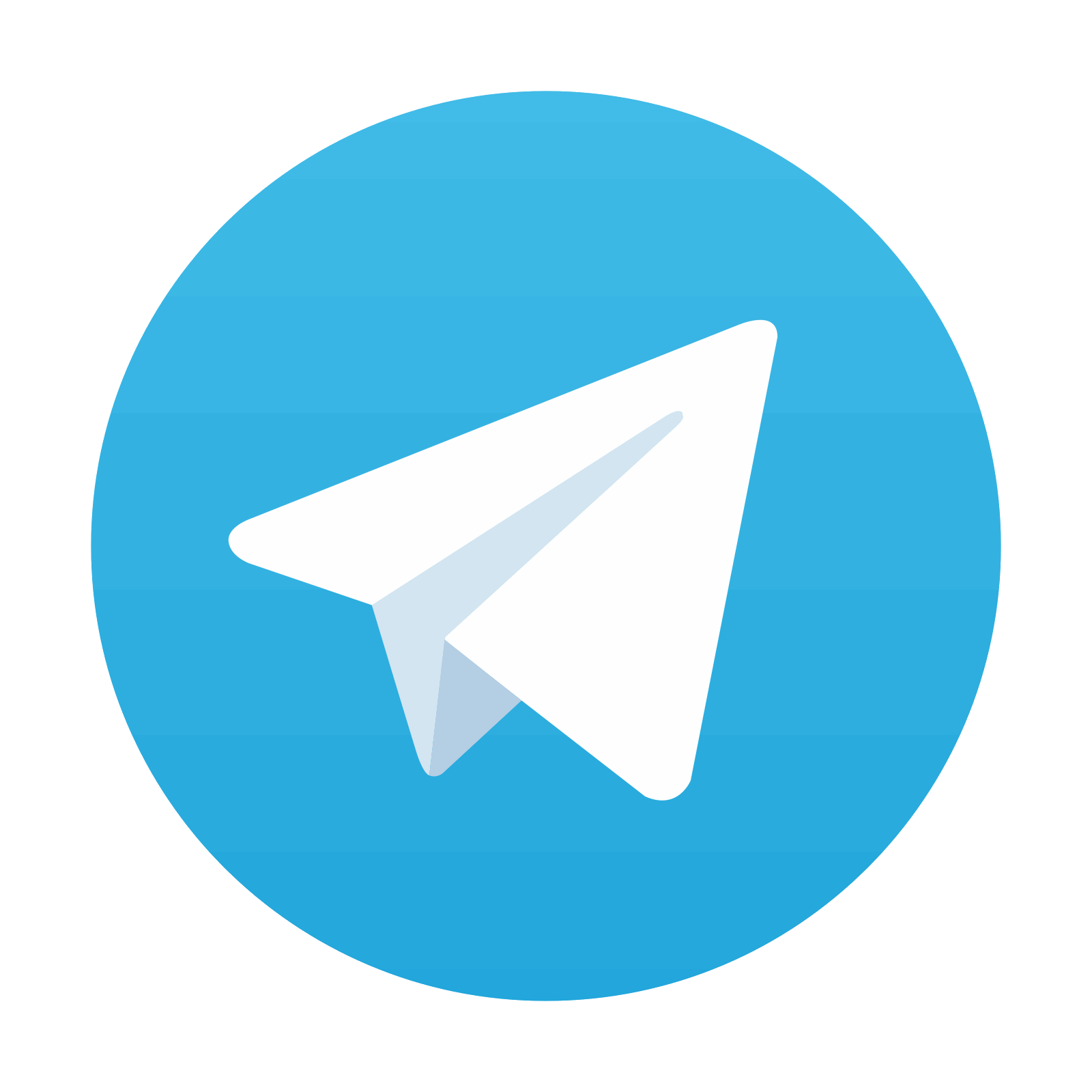
Stay updated, free articles. Join our Telegram channel

Full access? Get Clinical Tree
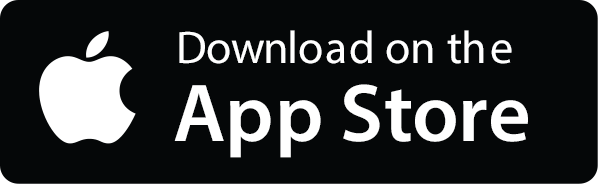
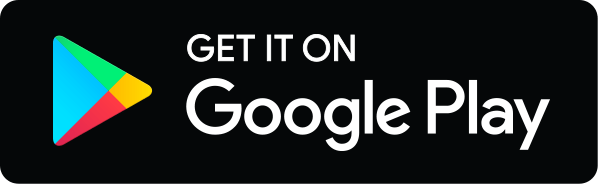
