Abstract
Acute kidney injury is one of the most common comorbidities among patients with congenital and acquired heart disease. Patients with acute kidney injury after cardiac surgery or with congestive heart failure have significantly higher incidence of morbidity and mortality. Surgery for congenital heart disease is the most common cause of acute kidney injury in childhood and the leading risk factor for mortality among patients with renal injury. In addition, the heart and kidney are intimately involved in maintaining homeostatic conditions in various disease states. This chapter will focus on normal and pathologic cardiorenal interactions. The diagnosis and significance of acute kidney injury in this population will be reviewed. Because there are no validated medication treatments for chronic kidney disease or acute kidney injury, this chapter will focus on the treatment of fluid overload, the primary sequela of kidney disease. Although diuretic medications are predominantly used as the primary therapy, renal replacement therapy has an important and growing role in this population.
Key Words
Acute kidney injury, cardiorenal interactions, cardiorenal syndrome, chronic kidney disease, renal replacement therapy
Acute kidney injury (AKI) is one of the most common comorbidities among patients with congenital and acquired heart disease. Patients with AKI after cardiac surgery or with congestive heart failure (CHF) have significantly higher incidence of morbidity and mortality. Surgery for congenital heart disease (CHD) is the most common cause of AKI in childhood and the leading risk factor for mortality among patients with renal injury. In addition, the heart and kidney are intimately involved in maintaining homeostatic conditions in various disease states. This chapter will focus on normal and pathologic cardiorenal interactions. The diagnosis and significance of AKI in this population will be reviewed. Because there are no validated medication treatments for chronic kidney disease (CKD) or AKI, this chapter will focus on the treatment of fluid overload, the primary sequela of kidney disease. Although diuretic medications are predominantly used as the primary therapy, renal replacement therapy (RRT) has an important and growing role in this population.
Vasomotor Balance in the Kidney
As with all organs, the kidney has the ability to maintain constant perfusion during a period of diminished blood flow through a process known as autoregulation. Afferent and efferent arterioles within the nephron dilate or constrict to modify vascular resistance and ensure a constant perfusion pressure in response to changes in cardiac output or metabolic demand. Although modulation of this system is necessary to ensure renal blood flow (RBF), this often has maladaptive effects, which in part explain sequelae such as AKI, fluid overload, and long-term outcomes of chronic cardiac and renal disease.
Renal Vasodilation
The natriuretic peptides, including atrial natriuretic peptide (ANP) and brain natriuretic peptide (BNP), produce multiple effects that collectively decrease blood pressure, commonly in response to fluid excess. Natriuretic peptides have properties that directly prevent salt and water retention, inhibit expression of vasoconstrictor peptides, decrease vascular resistance, down-regulate sympathetic activity, and increase vascular endothelial permeability. They also possess central activity to inhibit salt and water appetite and prevent compensatory cardiac hypertrophy. Natriuretic peptides are released in response to an increase in atrial-wall tension, often due to volume expansion, but are also released in response to ventricular stretch and an elevation in plasma levels of angiotensin II and endothelin-1. Renal effects of natriuretic peptides are mediated by direct activity on renal vasodilation and suppression of the renin-angiotensin-aldosterone system (RAAS). Sympathetic tone in the peripheral vasculature is decreased by a suppression of baroreceptors and decrease in catecholamine and sympathetic expression.
Systematic responsiveness to natriuretic peptides depends on patient age and duration of elevation. Animal models suggest the newborn is relatively insensitive to ANP after volume expansion, which may explain the diminished ability of infants to tolerate volume overload. Although natriuretic peptide concentrations are increased in patients with chronic heart failure, receptor downregulation and enzymatic upregulation cause a decrease in the renal responsiveness to ANP, resulting in hypertension and sodium and water retention.
Intrarenal prostaglandins such as prostaglandin E 1 (PGE 1 ), E 2 , and I 2 are renal cyclooxygenase metabolites that have vasodilatory properties. These molecules are important to balancing the vasoconstrictive effects of angiotensin and vasopressin on RBF to maintain fluid and salt removal. In animal models, prostaglandins have been demonstrated to maintain preservation of RBF during a period of acute reduction in total cardiac output and acute fluid volume depletion. In both infant and adult populations the use of medications that inhibit prostaglandin synthesis, such as nonsteroidal antiinflammatory drugs (NSAIDs), has been associated with acute renal failure and progression of CHF. Administration of a PGE 1 infusion in adults with chronic heart failure in a randomized clinical trial improved RBF and cardiovascular hemodynamics.
Nitric oxide (NO) has potent vasodilatory effects in the kidney and is involved in key pathways to counteract angiotensin’s effects. NO has been hypothesized to be involved in normal renal homeostasis, tubuloglomerular feedback response in pathologic processes, and natriuresis. However, in reperfusion/ischemia models, the roles of NO are less clear. Increased production of NO in AKI has been hypothesized to be protective by mediating vasodilation, inhibiting leukocyte adhesion, and reducing platelet aggregation. However, it is also believed that NO reacts with oxygen or superoxide to form injurious metabolites that cause oxidation injury and further upregulate the maladaptive inflammatory responses associated with AKI.
Bradykinin dilates the renovascular bed and is a key vasodilator targeted by pharmacologic intervention. Blockade of the renin-angiotensin system with angiotensin-converting enzyme (ACE) inhibitors decreases angiotensin II levels but also increases bradykinin levels. The angiotensin II receptor is divided into two subtypes (AT1 and AT2). AT1 blockade, with drugs such as losartan, appears to increase levels of angiotensin II, which is free to bind to the AT2 receptor subtype. The subsequent AT2 activation initiates a vasodilator cascade involving both bradykinin and NO. Animal models have demonstrated that bradykinin may play an important role in ameliorating renal ischemia-reperfusion injury by vascular changes, in addition to reducing DNA damage, apoptosis, and other morphologic renal changes. It may also have important protective roles in chronic renal failure to prevent hypertension, cardiac remodeling, and renal fibrosis.
Adrenomedullin is a peptide that functions as a renal vasodilator and has been posed to be protective in chronic heart failure. Adrenomedullin infusions in CHF patients decreased blood pressure, increased cardiac output, increased urine and sodium excretion, and decreased plasma aldosterone levels. It appears that the ability of adrenomedullin to inhibit oxidative stress production may combat changes induced by AKI and inflammatory pathways.
Renal Vasoconstriction
Physiologic stability of blood pressure, renal vascular tone, cardiac function, and salt and water homeostasis rely heavily upon the RAAS ( Fig. 16.1 ). Likewise, an exaggerated response of this hormonal system is a major driver of renal ischemia and AKI. In response to renal artery hypovolemia or hypotension, the kidney releases renin, which converts angiotensinogen to angiotensin I. This is converted to angiotensin II by ACE. Angiotensin II is a major vasoconstrictor and also stimulates the secretion of aldosterone from the adrenal cortex. Increased aldosterone levels promote sodium and water retention and potassium excretion by the kidney. Animal data suggest that the neonatal RAAS system is necessary for renal development because neonatal rats given ACE inhibitor medications developed persistent irreversible histopathologic renal abnormalities and abnormal renal functioning in adult life. This effect is diminished after the period of nephrogenesis, during the first 2 weeks of life in murine models. The activity of RAAS depends on age. Newborns have a very active RAAS with increased renin gene expression, angiotensin II expression, and plasma renin activity. The greater activity of RAAS in the newborn may explain their increased sensitivity to ACE inhibitors.

In response to hypovolemia and hypotension the endothelium releases various endothelial vasoconstrictor agents that are essential to maintaining consistent glomerular filtration rate (GFR). However, this response can cause excess vasoconstriction, which may impede RBF and lead to AKI. Ischemia may cause renal endothelial cell injury and microvascular dysregulation that might not improve when perfusion recovers. Primary pathways include the potent vasoconstrictor endothelin and products of the cyclooxygenase pathway, including thromboxane A 2 , prostaglandin H 2 , and superoxide anions. Animal models of AKI demonstrate increased local and systemic levels of endothelin, which are associated with increased endothelial dysfunction and renovascular constriction. Mouse models of renal artery ischemia demonstrated that blockade of endothelin results in increased RBF, increased GFR, and decreased afferent and efferent arteriolar resistance.
In response to a variety of physiologic stressors, catecholamines and arginine vasopressin (AVP), also known as antidiuretic hormone (ADH), are released. These endogenous hormones cause renal vascular vasoconstriction, which limits RBF and causes a reduction in GFR. Although commonly elevated in the postoperative setting, these hormones are also increased during states of hypovolemia, hypoxia, acidosis, hypercarbia, hypothermia, and pain. AVP can be administered as a synthetic peptide for maintenance of blood pressure and is commonly used in septic or cardiovascular shock. The renal effects of administration of AVP must be considered when using the medicine for hemodynamic purposes.
Cardiorenal Interactions
Pathophysiology of Salt and Water Retention
Cardiorenal interactions are central in the clinical presentation of CHF, low cardiac output syndrome, and other cardiac pathophysiology. Regardless of the primary cause, the body responds to a decrease in cardiac output via neurohormonal activation of the pathways mentioned earlier to maintain organ perfusion. With an upregulation in stress hormone response and the RAAS system, renal vasoconstriction causes a decrease in RBF and GFR ( Fig. 16.2 ). Increases in angiotensin II causes the release of endogenous vasopressin, and together these lead to increased absorption of sodium and free water from the renal tubule. This protective mechanism to maintain blood volume and blood pressure quickly becomes maladaptive as the renal tubules face the risk of ischemia, excess fluid is retained, and the struggling heart is forced to work against an increase in afterload.

In healthy patients, homeostasis is maintained because fluid retention causes atrial stretch stimulating the release of natriuretic peptides, which promotes salt and water excretion. However, persistent elevation of natriuretics with CHF causes the development of resistance to the renal effects, particularly in children. Elevation of BNP has been used in adults with CHD to predict mortality and in children with CHF to guide placement of ventricular assist devices (VADs) and has been demonstrated to correlate with outcome in the single-ventricle population. However, the BNP level in CHD is better used for monitoring trends because great variation is seen among different cardiac lesions and surgical procedure.
Angiotensin and Aldosterone Effects on the Heart
The effects of the RAAS pathway are more far-reaching than the temporary renal and vascular effects mentioned. Angiotensin acts as a growth factor, promotes fibrosis, and stimulates inflammation. Aldosterone has a maladaptive influence on the cardiac extracellular matrix in patients with CHF by stimulating cardiac collagen synthesis and fibroblast proliferation. Chronic elevation of angiotensin, renin, and aldosterone lead to pathologic left ventricular hypertrophy and myocardial fibrosis, independent of hypertension. In an effort to blunt these maladaptive interactions, pediatric and adult patients with CHF are treated with ACE inhibitors and spironolactone, an aldosterone receptor antagonist.
Cardiorenal Syndrome
Cardiorenal interactions are abundant in all critically ill patients, particularly those with impaired cardiac output. The pathophysiology of these interactions is best described by Ronco and colleagues, who defined the concept of the cardiorenal syndrome (CRS), a classification system that describes the time frame and origin of organ system dysfunction ( Fig. 16.3 ). Type 1 CRS describes an acute decrease in cardiac output leading to AKI. Type 2 is defined as chronic heart dysfunction leading to progressive CKD. Type 3 describes an acute worsening of renal function causing acute cardiac dysfunction. Type 4 is defined as CKD that contributes to adverse cardiovascular health. Type 5 is defined as a systemic condition such as sepsis, which causes both cardiac and renal dysfunction. Although most of the literature focuses on the effects of cardiopulmonary bypass (CPB) on AKI, a type 5 CRS, type 1 and 2 are frequently encountered. For example, type 1 CRS is seen during viral myocarditis, postoperative low cardiac output syndrome, acute heart transplant rejection, and innumerable other conditions with an acute decrease in cardiac output. Type 2 CRS is seen in patients with cardiomyopathy, failing Fontan physiology, or with graft dysfunction of a transplanted heart.

Defining cardiorenal interactions within this framework helps the clinician understand pathologic processes and appropriate management. For example, if a patient managed for chronic cardiomyopathy has an elevated creatinine level, oliguria, and fluid overload, a type 2 CRS, more diuretic may be detrimental, and some method of cardiac support may be necessary.
Cardiorenal Interactions in Specific Populations
There are specific pediatric cardiac cohorts that are particularly vulnerable to pathologic cardiorenal interactions. AKI after Fontan palliation is frequent in the postoperative period, but CKD remains common years after completion. This is likely secondary to multiple renal insults and persistence of a high central venous pressure (CVP). Even with the best Fontan hemodynamics, the renal venous pressure elevates 8 to 10 mm Hg above typical, significantly affecting renal perfusion pressure. Therefore patients with CKD secondary to suboptimal Fontan hemodynamics may see improvement with diuretics, pulmonary vasodilators, a Fontan revision, or improvement in atrioventricular synchrony.
Kidney injury among pediatric patients with CHF is common and associated with a higher rate of transplantation, mechanical support, and mortality. Among patients with a new diagnosis of dilated cardiomyopathy, one study showed 61% had decreased renal function, and this conferred a 5-year mortality hazard ratio of 2.4. The pathophysiology of cardiorenal interactions is often twofold; left heart failure causes decreased cardiac output and decreased renal perfusion, whereas right heart failure causes venous congestion and an elevation in renal venous pressure. This dichotomous etiology causes some to improve with diuresis and others to improve with vasoactive medications improving cardiac output, although often both are required. The presence of AKI in acute decompensation is especially common among those with an ejection fraction less than 25%. Among patients with CHF and CKD, some centers endorse a strategy of VAD placement to improve end-organ function before listing for transplantation. Unfortunately, adult data show the incidence of AKI remains high after VAD placement and portends a worse outcome. After cardiac transplantation in pediatric patients, AKI is common, affecting up to 73% of patients, and is associated with a worse outcome. This population continues to be at risk for CKD due to diastolic dysfunction of the graft transplant and need for treatment with nephrotoxic immunosuppressants.
An example of a type 3 cardiorenal interaction in children with heart disease is a patient who develops AKI due to nephrotoxic medication, leading to fluid retention. This may cause an increase in left ventricular afterload and depression of systolic cardiac function as the ventricle stretches along its Starling curve, particularly in a patient with impaired baseline cardiac function. Pediatric patients with cardiac disease, especially those with a history of AKI, are at risk for contrast- and medication-induced kidney injury due to the need for ACE inhibitors, NSAIDs, calcineurin inhibitors, or diuretics. Monitoring inpatient use of nephrotoxins with electronic medical records to limit overuse has been associated with decreased AKI incidence. Modifying composition and osmolality of contrast has been studied but not found to be protective. The use of N -acetylcysteine to prevent contrast-associated AKI has also been proposed, but thus far, avoidance of nephrotoxin exposure is the only proven method of decreasing the risk of nephrotoxic AKI.
Although less common, type 4 interactions are present in pediatric populations. Among children on dialysis, mortality has been reported to be as high as 50%, and the incidence of cardiovascular mortality of children on maintenance dialysis is roughly 1000-fold as high as the general population, most commonly due to cardiac arrest or arrhythmia. CKD leads to cardiac morbidity via multiple mechanisms, including chronic uremia, renal osteodystrophy, vitamin D pathophysiology, and fibroblast growth factor–related direct effects on the myocardium and the large vessels. It is hypothesized that younger pediatric patients are more sensitive to uremia because their rate of mortality is highest, despite the fact that they had not yet developed chronic changes of atherosclerosis, left ventricular hypertrophy, and cardiomyopathy.
Acute Kidney Injury
Pathophysiology of Acute Kidney Injury After Cardiac Surgery
The etiology of cardiac surgery associated AKI is multifactorial and incompletely understood, extending beyond low cardiac output and impaired RBF. The primary driving mechanisms include ischemia-reperfusion injury, mechanical blood trauma, oxidative stress, venous congestion, and proinflammatory cytokine activation. A reduction in mean arterial pressure and nonpulsatile flow during CPB result in activation of apoptotic and necrotic pathways of tubule and endothelial cell death. The kidney is able to tolerate up to 60 minutes of ischemia with only mild structural changes and no loss of function, but with release of vascular clamps, subsequent reperfusion injury results in activation of the oxidative pathways that exacerbate tubular and microvascular injury. Red cell hemolysis due to the CPB circuit releases free hemoglobin and iron, which exacerbates oxidant-mediated injury, resulting in release of proinflammatory cytokines from injured tubules and endothelial cells. Contact of the blood with the artificial surfaces of the CPB circuit exacerbates injury via activation of neutrophils, platelets, and proinflammatory cytokines. Perioperative nephrotoxic medication use may increase the severity of existing injury.
Incidence and Risk Factors for Acute Kidney Injury
AKI occurs in approximately 40% of children following cardiac surgery. AKI has consistently been associated with increased morbidity and mortality, including prolonged length of stay, prolonged need for mechanical ventilation, fluid and electrolyte disturbances, and changes in drug metabolism. The incidence of AKI is similar among those with CHF and receiving extracorporeal therapies. Adults with CHD may have other renal comorbidities, including diabetes and hypertension, and are at significant risk for postoperative AKI and CKD. In fact, nearly 50% of adults with CHD have some degree of renal dysfunction, and approximately 20% of those patients have moderate CKD. Compared to the general population, the incidence of renal dysfunction has been found to be 18 times higher in acyanotic adult patients, and 35 times higher in cyanotic patients. Renal function also is found to decline at a faster rate than in the general population.
Although perioperative risk factors for the development of AKI have been identified, few of them are modifiable. Younger age, longer CPB duration, higher surgical complexity, and preoperative ventilator support are risk factors for the development of AKI but also correlate with a sick patient substrate. Intraoperative risk factors include low blood pressure and hemoglobin during CPB, need for emergent intervention, and need for thoracic aortic surgery. After controlling for age and surgical severity; bypass times above 180 minutes had an odds ratio of 7.6 for the development of AKI. Postoperative risk factors include volume overload, preexisting CKD, nephrotoxin use, and use of mechanical circulatory support.
In patients with acute heart failure, age, inotrope use, and higher admission creatinine and blood urea nitrogen (BUN) were associated with severe AKI, in-hospital mortality, and need for mechanical circulatory support. Lesions with a high postoperative CVP also have high incidence of AKI. This includes lesions with residual right ventricular hypertrophy and diastolic dysfunction, such as tetralogy of Fallot, and lesions with obligate CVP elevation due to surgical palliation, such as the Fontan surgery.
Given the same set of risk factors and a similar renal insult, there will be some patients who develop AKI and others who do not. Although some of this may be due to chance or differences in surgical or bypass techniques, there is growing evidence that there may be gene variations that either predispose patients to or protect them from the development of AKI. Given the common mechanisms of postoperative AKI, polymorphisms associated with renal inflammatory, oxidative stress, or vasoconstrictor responses have been of highest interest. A study among adult patients undergoing aortic-coronary surgery found two alleles (interleukin 6–572C and angiotensinogen 842C) associated with AKI in whites. The ability to predict AKI increased fourfold when genetic polymorphism use was added to clinical risk factors.
Acute Kidney Injury Definitions
One of the major limitations for assessing AKI prevalence was lack of consensus definition, and many studies use variable definitions for AKI. A decade ago the Acute Dialysis Quality Initiative Group created the RIFLE criteria due to the lack of a consistent definition of AKI. Since then, several modifications have occurred. The RIFLE (Risk, Injury, Failure, Loss, End Stage) criteria were adapted to include children (pediatric RIFLE [pRIFLE]). This was followed by the Acute Kidney Injury Network (AKIN) criteria that expanded the diagnosis of AKI to include patients with an increase of 0.3 mg/dL or higher in serum creatinine level in a 48-hour period. Most recently the Kidney Disease Improving Global Outcomes (KDIGO) classification system integrated the RIFLE, pRIFLE, and AKIN classifications systems. The different classifications systems are summarized in Fig. 16.4 . All three definitions offer advantages: pRIFLE is sensitive, identifying more mild AKI cases; AKIN does not require heights or baseline creatinine; and KDIGO is applicable to both pediatric and adult populations with a less restrictive diagnostic time frame compared to AKIN.

Several adult and pediatric studies have compared incidence and outcomes across all definitions. Most recently Sutherland et al. reported an AKI incidence ranging from 37% to 51% of hospitalized children depending on the definition used. In this study intensive care unit (ICU) mortality increased with each AKI severity stage. Outside the ICU, AKI was not associated with mortality, but length of stay increased with AKI severity stage among all patients. Application of the three definitions with regard to the presence of AKI resulted in only moderate agreement. For AKI staging, AKIN and pRIFLE had 77% agreement, compared to KDIGO and AKIN, which had 93% agreement. These findings are similar to those described by Zappitelli et al., who found pRIFLE more sensitive than AKIN because it picked up more stage 1 events. Several adult studies have evaluated outcomes across definitions and have found that regardless of the definition used, AKI was associated with higher mortality.
Unfortunately, many studies use only creatinine criteria rather than urine output due to difficulties quantifying urine, use of diuretics, and the laborious data collection necessary for analysis. This may underestimate the incidence of AKI, in which urine output is a more sensitive marker of kidney injury. Furthermore, diagnosis is difficult in neonates who are born with an elevated creatinine level and a depressed GFR, which improves over the ensuing months.
Outcomes After Acute Kidney Injury
AKI after CPB is often a self-limited complication, occurring in the first 24 to 48 hours postoperatively. In the Translational Research Investigating Biomarker Endpoints in Acute Kidney Injury (TRIBE-AKI) consortium, almost half of patients met AKI diagnostic criteria for just 1 day and only one of nine patients still met the definition by the fourth postoperative day. For this reason the importance of postoperative AKI has historically been minimized. However, recent research has emphasized the strong association of AKI with worse postoperative and long-term outcomes.
AKI has been shown to be an independent risk factor for prolonged duration of mechanical ventilation, longer ICU and hospital stays, and mortality. In the TRIBE-AKI consortium 30% of patients with AKI were mechanically ventilated at 48 hours after surgery as opposed to 8% of those without AKI. Blinder and colleagues studied 430 infants after bypass, finding that 52% developed AKI. Stage 2 AKI was associated with a 5-times risk of death, and stage 3 AKI was associated with nearly a 10-times risk. These were stronger predictors of death than having single-ventricle physiology or needing mechanical circulatory support.
Even small increases in creatinine level are important. Compared to those with no change in creatinine level, adults after cardiac surgery with a creatinine level increase of just 0.1 to 0.5 mg/dL had a threefold increase in the rate of mortality, and this association worsened with larger creatinine changes. There are also data among pediatric patients after cardiac surgery that small early changes in creatinine level predict later development of AKI.
It previously was assumed that patients with a single episode of AKI would recover kidney function without long-term consequence. However, during the last decade epidemiologic data from critically ill children and adults suggest that AKI survivors are at considerable risk of developing CKD. Coca and colleagues demonstrated that adults who experienced AKI have a ninefold increased risk of developing CKD, a threefold increased risk of developing end-stage kidney disease, and a twofold increased long-term mortality risk as compared to those without AKI. Mammen and colleagues found that 10% of previously critically ill children (including patients following cardiac surgery) had developed CKD 1 to 3 years following AKI, and almost half were considered at risk of CKD development. Wong and colleagues describe the incidence and significance of AKI throughout the three stages of palliation for hypoplastic left heart syndrome and its variants. AKI was common among these high-risk patients, and the sequelae of severe AKI as a neonate were substantial, predisposing patients to death or need for extracorporeal membrane oxygenation (ECMO) after stage 1 palliation. Despite normalization of creatinine level, risk of severe AKI after stage 2 was elevated in those affected as a neonate. Morgan and colleagues followed a cohort of neonates after cardiac surgery and found that 2 to 4 years later those who developed AKI were at higher risk of growth impairment, cardiac-related hospitalization, and increased health care utilization, even when controlling for gestational age, surgical type, preoperative ventilation, lactate level elevation, and use of mechanical circulatory support. Additionally, although the creatinine level typically normalizes before discharge, there is evidence that those who were affected by AKI have persistent elevation of kidney injury biomarkers up to 7 years postoperatively. These data highlight the importance of following AKI survivors throughout adulthood to understand the long-term implications of AKI.
Acute Kidney Injury Biomarkers
Novel AKI biomarkers in the serum and urine have been developed that offer additive benefit to the traditional approach of measuring serum creatinine level. Many of these biomarkers have been studied in children following cardiac surgery because children lack the comorbidities seen with adults, and the timing of injury is well defined. Biomarkers are rapidly becoming the framework for research investigation and clinical management and are being used to leverage the possibility of detecting kidney injury before obvious functional changes such as a decrease in urine output or increase in serum creatinine level occur ( Fig. 16.5 ).

Several biomarker categories exist: (1) markers of glomerular filtration, (2) markers of tubular injury, (3) markers of cell-cycle arrest, and (4) markers of inflammation. A detailed discussion of each group is beyond the scope of this chapter and can be found elsewhere. Three biomarkers, cystatin C, neutrophil gelatinase–associated lipocalin (NGAL), and [TIMP-2]*[IGFBP-7] (the product of tissue inhibitor of metalloproteinase-2 [TIMP-2] and insulin-like growth factor–binding protein 7 [IFGBP-7]) are currently being used clinically and are discussed briefly.
Cystatin C is a marker of glomerular filtration that is not subject to the inherent limitations seen with creatinine, specifically gender and muscle mass. Measurement is available in many hospitals and laboratories. Two meta-analyses demonstrated that serum cystatin C is superior to creatinine for the diagnosis and risk stratification of AKI severity. A prospective multicenter study in children following cardiac surgery showed that early measurement of cystatin C by 6 hours following CPB initiation strongly and independently predicted the later development of serum creatinine–based AKI with an adjusted odds ratio (OR) of 17.2 and an area under the curve (AUC) of 0.89.
NGAL is a marker of tubular injury, and is upregulated very early after injury. Well over 300 publications have reported on NGAL in human AKI, with several systematic reviews and meta-analyses of its diagnostic utility. NGAL significantly improved risk prediction over clinical models alone and is predictive of graded AKI severity and duration. Clinical trials are beginning to use NGAL as an outcome because it is a more sensitive and earlier indicator of AKI then other methods. Most recently a prospective randomized double-blind controlled trial demonstrated a renoprotective effect of fenoldopam by a dramatic reduction in urine NGAL levels compared to the placebo group in children following cardiac surgery. Although clinical use is becoming more common outside the United States, it is not yet FDA approved. Increased sensitivity above the “gold standard” of creatinine allows discovery of subclinical AKI, in which serum creatinine is negative but NGAL is positive. Subclinical AKI is associated with a twofold to threefold increased risk of death or dialysis in both adults and children. NGAL has important limitations, including persistent elevation in CKD. It has also not performed as well in adult cohorts, possibly due to other comorbidities. This test has a fairly large indeterminate zone (100 to 150 ng/mL), which requires the clinician to use other clinical or laboratory corroborators of renal risk.
Recently the FDA approved a urine-based test (NephroCheck) that uses the product of two cell-cycle arrest biomarkers, TIMP-2 and IFGBP-7, to risk stratify critically ill adults for developing AKI within 12 hours after testing. These individual biomarkers are inducers of G1 cell-cycle arrest, a mechanism in the progression of AKI. In adults, [TIMP-2]*[IGFBP-7] has been shown to predict AKI as early as 4 hours post bypass and to accurately predict renal recovery. Although not yet validated in pediatric populations, this biomarker combination shows promise of early diagnosis and may be useful particularly in postoperative patients.
It is likely that future diagnostics will be aided by the use of biomarkers. Furthermore, panels of biomarkers that detect different injury pathways, often with different temporality, will maximize the diagnosis of AKI and potentially illustrate the pathophysiology of injury. As a precursor to this concept, Basu and colleagues demonstrated that combining the functional biomarker cystatin C and the tubular injury biomarker NGAL could increase sensitivity and specificity of the diagnosis of AKI in children after CPB superior to creatinine alone.
Fluid Overload
It has been hypothesized that AKI and CKD are markers of poor perioperative condition and hemodynamic instability and that kidney disease itself does not lead to morbidity and mortality, but that continued poor cardiac output is ultimately to blame. In fact, there is an adage, “No acute renal failure patients have ever died because of acute renal failure, but they have died in acute renal failure.” This notion has lost favor as research has stressed the independent association of kidney disease with morbidity. The mechanism by which AKI and CKD cause worse outcomes is primarily the development of fluid overload. Renal tubule injury and hypoperfusion lead to a decrease in GFR, which causes retention of free water along with the other retained filtrate. Progressive oliguria or anuria leads to fluid overload. In addition to external swelling, edema of various organs occurs, with ascites, pulmonary edema, pleural effusions, myocardial wall edema, and bowel wall edema. These edematous organs all individually become dysfunctional; pulmonary gas exchange is impaired and lung compliance decreases; bowel walls absorb less nutrition; skin breakdown leads to pressure ulcers and infection at catheter sites; ascites impairs cardiac preload, limits lung excursion, and causes abdominal tamponade of peritoneal organs; and the swollen cardiac myocardium is less contractile and suffers from diastolic dysfunction. Among the other morbid effects, these collectively further impair cardiac output and renal perfusion, which worsens renal dysfunction, leading to progressive fluid overload. Without the ability to break this cycle, AKI and CKD quickly may become major contributors to morbidity and mortality.
A large study from the Prospective Pediatric Continuous Renal Replacement Therapy Registry group showed that patients with multiorgan failure had a 3% higher risk of mortality for every additional 1% of fluid overload, and that a greater than 20% fluid overload conferred an 8.5-times mortality risk. It has been demonstrated that increasing percentage fluid overload is associated with worsening oxygenation index in children. In the pediatric population with cardiac disease, retrospective studies have demonstrated an association between early postoperative fluid overload and worse outcomes, including the duration of ICU stay, inotropic medication use, duration of mechanical ventilation, and oxygenation index. In neonates after cardiac surgery, greater than 16% fluid overload was an independent predictor of poor outcome (death, ECMO, or need for dialysis).
In addition to its negative sequelae, fluid overload may also obfuscate the diagnosis of AKI if the excess intravascular volume dilutes the measured creatinine concentration. In a study of postoperative neonates, correcting serum creatinine concentration for the degree of fluid overload unmasked additional cases of AKI. In these patients, previously unrecognized AKI was associated with a worse outcome. Novel biomarkers that are less affected by dilution may help diagnose this “subclinical” AKI. Among patients after cardiac surgery, NGAL elevation, even in the absence of a creatinine rise, is associated with worse outcomes.
Because there are no validated medications to treat AKI, the focus of management often shifts to control of fluid overload. Without a doubt, the most quintessential management for fluid overload is restriction of input, but this is easier said than done. A common postoperative total fluid allowance for an infant after surgery is two-thirds of maintenance. However, the challenge of management can be illustrated in the example of a critically ill 3-kg patient who is restricted to 8 mL per hour. This volume will be exceeded by inotropic infusions, continuous sedation medications, diuretics, necessary electrolyte replacements, and line flushes, even if adequate nutrition is withheld. Furthermore, if the infant is oliguric, they may make 1 mL of urine per hour, and even the most modest fluid inputs will lead to fluid overload.
Pharmacologic interventions intended to improve urine output include diuretics, renal vasodilators, and medications to improve cardiac output. Renal vasodilators and medications to improve cardiac output are theorized to improve glomerular perfusion, therefore improving urine output and potentially mitigating AKI. Although retrospective studies of fenoldopam, nesiritide, and aminophylline have suggested an improvement in urine output in neonates after cardiac surgery, prospective studies have not shown benefit. Diuretic use in critically ill patients with AKI is ubiquitous; however, diuretics are often ineffective and may even be detrimental, associated in some studies with a higher risk of mortality.
Renal Pharmacology
Diuretics
Diuretics are the mainstay of fluid removal in critically ill children with heart disease. The location of action of the various diuretic classes can be seen in Fig. 16.6 . In the case of tubular immaturity, delivery of diuretics to their site of action is slower, leading to a delayed onset of action and an increased elimination time, which may prolong diuretic effect. Disease states may decrease renal sensitivity to diuretic medications. Specific dosing and monitoring recommendations are beyond the scope of this chapter and can be found in several other excellent sources.

Loop Diuretics.
Loop diuretics are the most commonly used diuretic in the management of volume overload in CHF and following cardiac surgery. They may be used alone or in combination with other diuretic classes to achieve a synergistic response. Of all the diuretics classes, they are functionally the most potent and can block up to 25% of sodium reabsorption. They inhibit sodium and chloride reabsorption in the thick ascending loop of Henle via the sodium-potassium-chloride (Na + /K + /2Cl − ) cotransporter. This receptor is located in the urinary side of the tubular lumen, and thus the loop diuretic must be filtered to be effective. This is critical because if filtrate is not produced due to AKI or inadequate renal perfusion, loop diuretics cannot reach their target. Therefore increasing the dose may be ineffective, and alternative fluid removal strategies may be needed. Common loop diuretics include furosemide, bumetanide, and ethacrynic acid, and are administered intravenously or orally. To avoid rapid fluid shifts and hemodynamic instability, continuous infusions may be used.
Furosemide.
Furosemide is the most common diuretic used. It is a potent bilirubin displacer of the albumin binding sites, and therefore critically ill premature neonates may be at higher risk for hyperbilirubinemia. Furosemide is eliminated by hepatic and renal glucuronidation, with 90% appearing as unchanged drug in the urine.
Bumetanide.
This loop diuretic is 40 times more potent that furosemide. Because therapeutic concentrations displace bilirubin from albumin to a lesser extent, it may be safer in neonates with hyperbilirubinemia. There is anecdotal evidence that a bumetanide infusion has increased compatibility with a large group of drugs compared to furosemide.
Ethacrynic Acid.
Ethacrynic acid is rapidly absorbed and hepatically metabolized to an active cysteine conjugate. A recent clinical trial compared furosemide and ethacrynic acid in pediatric patients after cardiac surgery and found ethacrynic acid produced more urine output with no differences in renal function.
Side Effects.
The most significant effects include electrolyte derangements: hyponatremia, hypocalcemia, hypokalemia, hypochloremia and hypomagnesemia, and a resultant contraction metabolic alkalosis. Transient or permanent ototoxicity may occur with prolonged administration and high doses of all loop diuretics, especially ethacrynic acid, but potentially less likely with bumetanide. Nephrocalcinosis may also occur with higher doses and increased duration and is more common among premature infants.
Thiazide and Thiazide-like Diuretics.
Thiazide diuretics are considered to be of moderate potency and are most often used in combination with loop diuretics to achieve a more potent effect. They function by blocking the thiazide-sensitive Na + /Cl − cotransporter in the distal convoluted tubule. Potassium reabsorption is blocked, and carbonic anhydrase is also is inhibited, thereby allowing for the excretion of bicarbonate, sodium, and chloride, along with water. Thiazide-like diuretics primarily act upon the distal convoluted tubule and secondarily upon the proximal tubule. In these regions, metolazone inhibits sodium reabsorption, causing increased excretion of sodium and water, as well as potassium and hydrogen ions. When thiazides and thiazide-like diuretics are used in combination with loop diuretics, there is a synergistic diuretic effect created by sequential nephron blockade of the proximal and distal tubule. The risk for electrolyte disturbances, renal dysfunction, and hypovolemia is enhanced with drug combinations. Common thiazides include chlorothiazide and hydrochlorothiazide. Metolazone is the most commonly used thiazide-like diuretic but is erratically absorbed, unlike the less commonly used Mykrox formulation, which is 100% bioavailable. Side effects of this class include electrolyte disturbances, hyperuricemia, drug fever, hypersensitivity reaction, cholestasis, dermatitis, and vasculitis. Lipid and carbohydrate metabolism disturbances have been seen in adults with long-term thiazide diuretic use but have not been described in children. Thiazides cause less calcium loss and therefore are sometimes preferred in the premature neonatal population.
Aldosterone Receptor Antagonists/Potassium-Sparing Diuretics.
Spironolactone, a synthetic steroid, is an aldosterone receptor antagonist with relatively weak diuretic effects and is most commonly used for its potassium-sparing effects. It is the only diuretic that does not need to reach the tubular lumen to exert its action. Spironolactone competes with aldosterone for the mineralocorticoid receptor, leading to decreased potassium secretion and decreased sodium and chloride reabsorption. In conjunction with other diuretics, there is an increased diuretic effect due to sequential nephron blockade. This drug class has antifibrotic effects and promotes reverse remodeling through antagonizing the RAAS. Although no pediatric data exist, adult data showed improved survival when combined with ACE inhibitors and beta-blockers. Potassium levels should be monitored closely, especially when using with other medications that can increase serum potassium (e.g., ACE inhibitors). Gynecomastia is related to dose and duration of therapy and is usually reversible with cessation of spironolactone.
Other Diuretics.
Carbonic anhydrase inhibitors (acetazolamide) are weak diuretics, with the main site of action in the proximal tubular lumen and cell. Blockade of carbonic anhydrase leads to decreased bicarbonate and sodium reabsorption via the Na + /HCO 3 − cotransporter, resulting in reduced water reabsorption. They are most often used for the treatment of a significant metabolic alkalosis. Acetazolamide may promote nephrocalcinosis and nephrolithiasis when combined with loop diuretics. Osmotic diuretics (mannitol) act mainly in the proximal tubule. Mannitol undergoes glomerular filtration and is not reabsorbed along the tubular system. Osmotic diuretics increase osmolality of the tubular fluid and subsequently water and sodium excretion. Mannitol results in a shift of extracellular fluid into the intravascular space in infants with low cardiac output and poor renal perfusion after cardiac surgery. It therefore has the potential to exacerbate CHF and pulmonary edema in patients with myocardial dysfunction.
A common complication of CHF is acute and chronic renal disease with hypervolemic hyponatremia. This can be attributed to venous congestion, decreased renal perfusion, and extended use of diuretics. Although diuretics are necessary, the associated electrolyte abnormalities may exacerbate hyponatremia by limiting the kidney’s ability to excrete free water. Tolvaptan is an oral selective vasopressin V 2 -receptor antagonist that induces hypotonic diuresis without affecting electrolytes. The efficacy and safety of tolvaptan is established in adult patients. A multicenter clinical trial of tolvaptan in children with euvolemic or hypervolemic hyponatremia is currently under way ( ClinicalTrials.gov , NCT02012959).
Angiotensin-Converting Enzyme Inhibitors/Angiotensin Receptor Blockers
The primary goal of ACE inhibitors is typically to decrease ventricular afterload via blockade of the RAAS system and vasodilation. However, as mentioned previously, ACE inhibitors also prevent and even reverse pathophysiologic myocardial remodeling and have been demonstrated to decrease mortality and hospitalization in adults with CHF. Several pediatric retrospective cohort and case-control studies have demonstrated improvement in hemodynamics and echocardiographic parameters. A prospective randomized controlled trial in which patients with single ventricle were randomized to receive enalapril or placebo did not result in improved outcomes, and therefore the routine use of ACE inhibitors in this population is not substantiated. It is recommended to start at the lowest dose and titrate to avoid hypotension. ACE inhibitors may cause hyperkalemia, and potassium levels should be monitored closely, particularly in patients also receiving spironolactone or other potassium replacements. Renal function may worsen with ACE inhibitors and should also be periodically monitored. In adults, angiotensin receptor blockers (ARBs) have similar benefits to ACE inhibitors. There is no evidence in children to suggest that ARBs are superior to ACE inhibitors.
Medications to Prevent Acute Kidney Injury
Several pediatric trials have been conducted evaluating pharmacologic methods to reduce rates of AKI and its associated complications with mixed results. Fenoldopam is a short-acting dopamine A1 receptor agonist that promotes renal artery vasodilation and is proposed to be renal protective. Unfortunately, the evidence in both adults and children is equivocal. Adult studies have shown that fenoldopam may reduce AKI but has no effect on survival or need for RRT. Retrospective studies in children demonstrated increased urine output in some studies but no effect in others. Ricci and colleagues performed a placebo controlled trial of fenoldopam in neonates after CPB and found no difference in renal function, frequency of AKI, fluid balance, duration of ventilation, or hospital length of stay, although in a subsequent trial they found biomarker improvement of AKI and decreased use of diuretics. Aminophylline is a phosphodiesterase inhibitor and adenosine receptor blocker, which both result in renal vasodilation. Tamburro and colleagues recently found that administration of aminophylline was associated with an increase in urine output, without a change in creatinine or BUN, but a larger placebo controlled trial by Axelrod and colleagues found no effect of aminophylline to prevent AKI in children after cardiac surgery. Importantly, in this study aminophylline was administered as late as 10 hours after initiation of CPB. At this time, injury may have already occurred, thus mitigating any potential benefit of the drug. N-acetylcysteine (NAC) is a derivative of the naturally occurring amino acid l -cysteine and is thought to prevent oxidative stress and scavenge oxygen free radicals, limiting ischemia reperfusion and modulating apoptosis. It has been used prophylactically to prevent renal injury after intravenous contrast for radiology studies. Two studies in patients with CKD found a reduction in mechanical ventilation duration, intensive care length of stay, and mortality. In a placebo controlled trial, neonates receiving perioperative NAC after the arterial switch procedure demonstrated better urine output, shorter time to negative fluid balance, and less creatinine elevation.
Drug Dosing in Acute Kidney Injury and Renal Replacement Therapy
Much of the pediatric drug dosing in patients with depressed GFR or in those receiving RRT is derived from adult data. There are several considerations specific to drug dosing in children. Critical illness can affect the GFR more in neonates than in adults, and GFR does not reach adult levels until 2 years of age. The quality and quantity of plasma proteins do not reach adult levels until a year of age, and protein binding may vary. The volume of distribution of water-soluble drugs is larger relative to body weight in pediatric patients than adults. Nephrotoxic medications should be avoided when possible because this is one of the only modifiable AKI risk factors, and review of all medications should be performed in a patient with a creatinine elevation irrespective of AKI diagnosis. In addition, factors that affect creatinine levels, including volume status, muscle mass, and medications, should be accounted for, and use of cystatin C should be considered for a more accurate assessment of GFR.
Drug clearance on RRT is dependent on rate of ultrafiltration and several molecular characteristics of the specific medication, including :
- 1.
Molecular weight : Smaller molecules are more readily removed. Continuous renal replacement therapy (CRRT) membranes have larger pores than intermittent hemodialysis (HD) and thus cause greater clearance.
- 2.
Charge: Drugs with an anionic charge pass through the membrane more easily than cationic drugs, such as aminoglycosides.
- 3.
Volume of distribution : Drugs with a greater volume of distribution are less cleared by RRT. However, this property is less important with slow continuous CRRT.
- 4.
Water solubility: Drugs that are highly water soluble have a lower volume of distribution, and thus more drug is available for removal by RRT. In contrast, highly fat-soluble drugs are not removed easily.
- 5.
Protein binding: Drugs that are highly protein bound are not readily removed. The clinician should consult both pharmacy and nephrology experts for drug dosing on RRT.
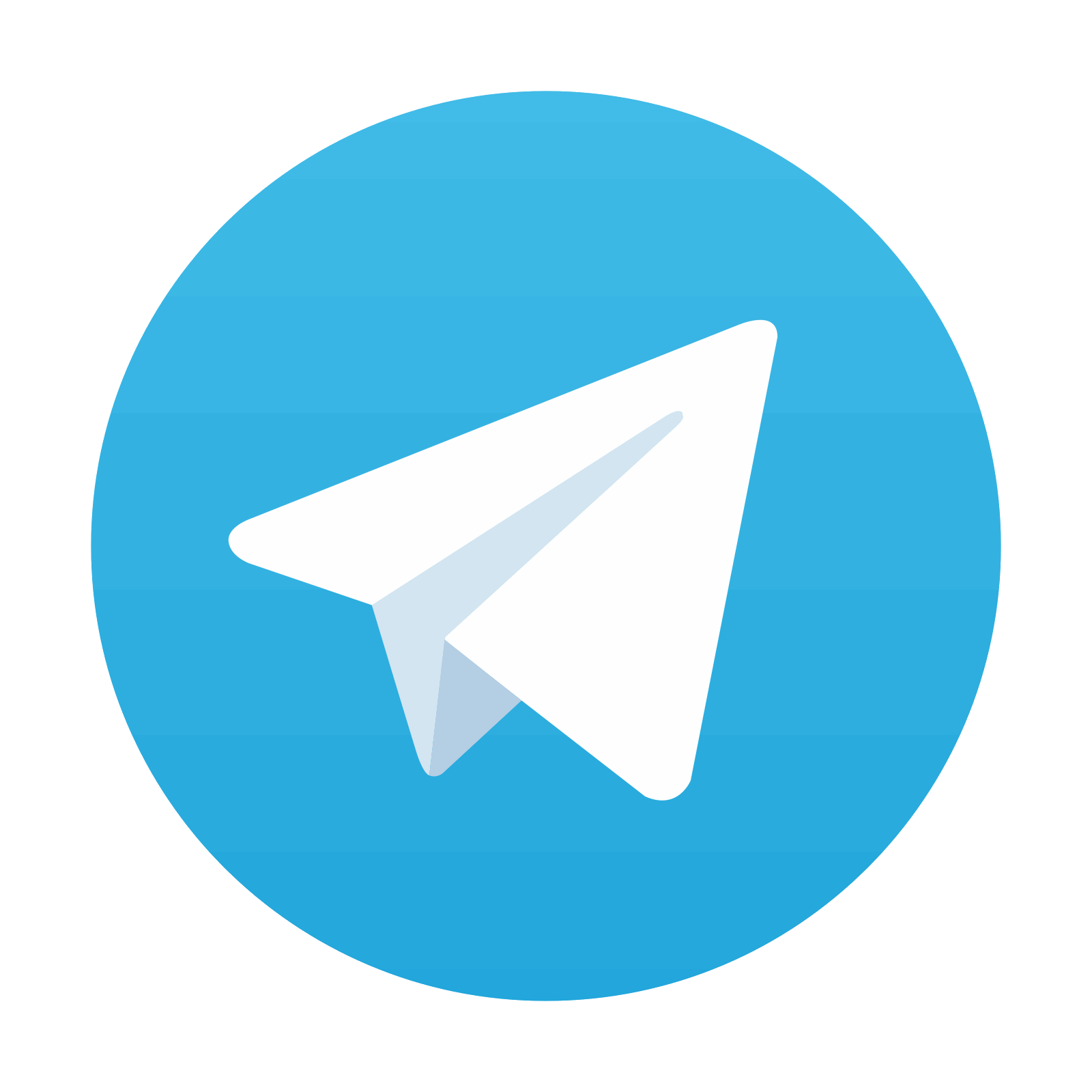
Stay updated, free articles. Join our Telegram channel

Full access? Get Clinical Tree
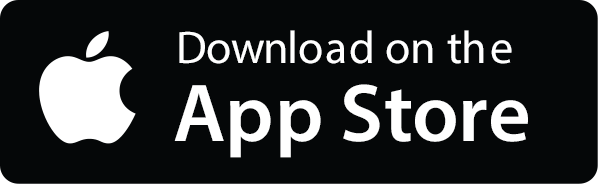
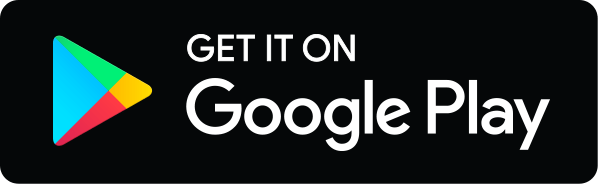