, Dilip R. Karnad2 and Snehal Kothari3
(1)
Cardiac Safety Services Quintiles, Durham, North Carolina, USA
(2)
Research Team, Cardiac Safety Services Quintiles, Mumbai, India
(3)
Cardiac Safety Services Global Head, Cardiac Safety Center of Excellence Quintiles, Mumbai, India
Treating physicians need to be thoroughly acquainted with the cardiotoxic effects of anti-cancer drugs so that they can diagnose them early on and avoid jeopardizing the overall success of treatment (Schlitt et al. 2014).
10.1 Introduction
The three preceding chapters, which comprise Part IV of this book, discussed topics falling within the domain of proarrhythmic cardiac safety. As we move into Part V, attention now broadens to other domains within the field of cardiovascular safety. This chapter addresses cardiotoxicity considerations in the therapeutic use of oncologic agents. The construct and employment of benefit–risk assessments have been discussed in several contexts in previous chapters. As we move into discussions related to the therapeutic use of drugs that have received marketing approval, the observation by Garattini (2010) that “drug authorization, prescription, and utilization are all based on benefit-risk assessment” provides an integrated perspective on this topic.
Oncology pharmacotherapy provides a good example of how a drug’s benefit–risk balance can be favorable even though the drug has well-acknowledged and relatively severe safety liabilities. Consider a scenario in which a patient has been told that his or her cancer will be terminal in several months, with 6 months being the physician’s best guess. Some patients may be willing to take a drug with a sizeable toxicity liability in an attempt to prolong their life by several additional months and/or to improve their quality of life for their remaining time. In this scenario, the risk of cardiotoxicity several years in the future (or even in the short term) may not outweigh the patients’ desire to try to maximize their life expectancy and quality of life by obtaining the therapeutic benefit of the drug: they may therefore deem the treatment option to have a favorable benefit–risk balance (Turner and Durham 2009).
However, a different scenario is now much more representative of current oncology pharmacotherapy. Advances on many fronts mean that drugs (sometimes in combination with surgery and/or radiation therapy) are now prolonging patients’ lives to a much greater degree. This is a laudable achievement. However, in the context of this chapter’s discussions, there is a significant corollary: patients can experience the clinical manifestations of cardiotoxicity on various timelines, including over a decade after cessation of therapy. These manifestations include cardiac dysrhythmias, cardiomyopathy, congestive heart failure, hypertension, ischemia and myocardial infarction, pericarditis or myocarditis, and valvular heart disease (Ferri et al. 2013). Cancer survivors therefore represent a population for whom ongoing medical monitoring and surveillance, and prevention or treatment of these clinical conditions, is of marked importance. As Cardinale and colleagues (2013) observed, “Cardiotoxicity due to cancer treatment is of rising concern, for both cardiologists and oncologists, because it may have a significant impact on cancer patient management and outcome.”
Two important papers published 25 years ago discussed cardiotoxicity manifesting itself following cessation of treatment with anthracyclines. Lipshultz and colleagues (1991) assessed the cardiac status of 115 children who had previously been treated with doxorubicin for acute lymphoblastic leukemia. Their disease was in continuous remission, and the median interval between cessation of treatment and cardiac evaluation was 6.4 years. Fifty-seven percent of the patients had left ventricular contractility abnormalities, with the cumulative dose of doxorubicin administered during treatment being the most significant predictor of abnormal cardiac function. Eleven patients had congestive heart failure within 1 year of treatment, five had recurrent heart failure between 3 and 10 years after completing treatment, and two required heart transplantation. The authors concluded as follows: “Doxorubicin therapy in childhood impairs myocardial growth in a dose-related fashion and results in a progressive increase in left ventricular afterload sometimes accompanied by reduced contractility. We hypothesize that the loss of myocytes during doxorubicin therapy in childhood might result in inadequate left ventricular mass and clinically important heart disease in later years” (Lipshultz et al. 1991).
Steinherz and colleagues (1991) examined cardiac toxicity in 201 long-term survivors of pediatric malignancies between 4 and 20 years after completing anthracycline therapy: the median time from completion of treatment was 7 years. Echocardiography revealed that 23 % of the patients had abnormal cardiac function, and cumulative dose was again associated with the incidence of abnormalities. Of the original 201 patients, 56 individuals were followed for 10 years or more (with a median of 12 years): 38 %, an even higher percentage, had abnormal findings. The authors concluded that “The 23 % incidence of late cardiac abnormalities warrants continued evaluation of patients after anthracyclines to guide patient care and the design of future chemotherapeutic protocols” (Steinherz et al. 1991). This led to the current box warning for doxorubicin, which reads as follows: “Myocardial damage may lead to congestive heart failure and may occur as the total cumulative dose of doxorubicin HCl approaches 550 mg/m2. The risk of cardiomyopathy may be increased at lower cumulative doses with mediastinal irradiation” (Doxorubicin Package Insert 2016).
Across the next two decades, evidence continued to accumulate that “Cardiac disease in patients with cancer is common and influences the longevity and quality of life both of patients in active treatment and of survivors of cancer,” and, within the therapeutic specializations of cardiology and oncology, there was increasing recognition of the benefits to patients of cardiologists and oncologists collaborating in the care of cancer patients with cardiac disease (Lenihan et al. 2010). This recognition led to the creation of the International CardiOncology Society, which was founded at a conference in Milan, Italy, in 2009 (Lenihan et al. 2010).
10.2 Cardiac Imaging Techniques
Heart abnormalities, whether drug-induced or not, require methods of assessment. Christian and colleagues (2012) discussed three commonly used imaging modalities: echocardiography, magnetic resonance imaging (MRI), and radionuclide (nuclear) imaging. Each is discussed in turn.
10.2.1 Echocardiography
Echocardiography is a widely applied noninvasive modality that provides data on cardiac structure and function. Two-dimensional (2D) echocardiography with Doppler flow assessments can characterize hemodynamics and systolic/diastolic function and display cardiac structures and measurements of cardiac chamber sizes. Three-dimensional (3D) echocardiography offers the potential to enhance assessments of cardiac function, structure, and geometry.
The echocardiography measurement modality has both advantages and disadvantages. Advantages include the following:
It is easily performed, widely available, relatively inexpensive, and highly portable. There is no ionizing radiation, and there are no limitations with regards to patients’ experiencing claustrophobia or with existing metallic implants.
While the use of contrast agents enhances image quality and reduces variability, they are generally not required except when image quality is particularly poor.
It provides real-time data and is a preferred method when frequent assessments are required.
It provides detailed information on cardiac structure (atrial and ventricular cavity dimensions, areas, volumes, wall thickness, and mass), cardiac function (systolic and diastolic, right and left ventricular wall motion, fractional shortening and ejection fraction, global and regional, and preload and afterload), valvular disease (structure, function, and degree of regurgitation or stenosis), vascular structures, and hemodynamic data.
Disadvantages include the following:
Image variability. Sources of variability during image acquisition include the skill level of the sonographer, employment of different machines or imaging modes (e.g., fundamental imaging or harmonic imaging, 2D, or 3D), and biological variability of the patient (e.g., changes in preload or afterload). During image interpretation, sources include the algorithms used (e.g., single-plane or biplane method for left ventricular ejection fraction) and inter-reader variability. During chemotherapy in patients with advanced cancer, marked changes in intravascular volume status, heart rate, or blood pressure also potentially contribute to increased variability in serial assessments.
Image quality. Poor acoustic windows can result in poor visualization of the left ventricular endocardial border, making quantitation of LVEF difficult or inaccurate: this can happen in up to 10 % of patients (Christian et al. 2012).
10.2.2 Magnetic Resonance Imaging
Cardiac magnetic resonance imaging (MRI) is an accurate, reproducible, and well-validated technique for measuring left and right ventricular volumes and mass and is a recognized imaging modality for many cardiovascular applications (Pennell et al. 2004). Advantages include the following: production of high-resolution images, precision that is reliable even after cardiac remodeling, high reproducibility, and comprehensive data collection. With regard to the last advantage listed, cardiac MRI provides comprehensive structural and functional data including left and right ventricular dimensions, volume, mass, and systolic function, and degree of myocardial fibrosis and/or inflammation. Disadvantages include its higher cost relative to echocardiography and nuclear imaging, potentially limited access, and contraindications. Contraindications include patients’ claustrophobia, metallic implants (e.g., pacemakers, defibrillators, insulin pumps, aneurysm clips, and any other foreign metallic objects), and limitation in large body size (Christian et al. 2012).
10.2.3 Radionuclide (Nuclear) Imaging
Nuclear imaging has a long history of employment in detection and monitoring of chemotherapy-induced cardiotoxicity (Alexander et al. 1979). Advantages of this modality include that it is relatively operator independent, less expensive than MRI, and a well-established standard for monitoring left ventricular function. Accuracy and precision of left ventricular systolic function is good, and hence reproducibility of LVEF measurements is good. Disadvantages include the following:
It is expensive compared with echocardiography.
It is not as readily available as echocardiography. Treatment centers’ availability of and expertise in specific nuclear imaging modalities vary.
It requires exposure to ionizing radiation, which can be especially limiting when multiple occasions of measurement are needed.
It provides limited information on cardiac anatomical details.
10.3 Biomarkers
Lenihan and Cardinale (2012) grouped the multiple potential cardiovascular complications of oncologic therapy into three main categories:
Vascular conditions: these include atherosclerosis, thrombosis, and hypertension and are predominant.
Cardiac structural problems: these, especially valvular degeneration, can have a dramatic long-term impact.
Cardiac dysfunction and heart failure: these potentially common late effects can be prevented or detected early during active cancer therapy to result in optimal patient outcomes.
The authors emphasized the importance of the latter category precisely because of the benefits of early identification and appropriate intervention. They also observed that future research on late cardiac effects in cancer survivors needed to include “advanced cardiac imaging techniques, novel cardiac biomarkers, and genetic determinants of response to cancer treatment” (Lenihan and Cardinale 2012). This section discusses biomarkers.
Decisions to investigate the potential utility of existing biomarkers and their associated assays have typically been predicated on their employment in assessing cardiac dysfunction in other settings, such as ischemia and heart failure (Morrow et al. 2007; Yancy et al. 2013). Biomarkers of interest in this domain include troponin (cTn), natriuretic peptides, glycogen phosphorylase BB (GPBB), myeloperoxidase (MPO), and circulating microRNAs (Christenson et al. 2015).
While echocardiography is currently commonly used to assess cardiac function prior to and during therapy with anthracyclines, this technique is acknowledged to be insensitive. Normal hearts “have excellent reserve capacity, so even modest loss of left ventricular function is indicative of significant cardiovascular damage” (Christenson et al. 2015; see also Ewer and Lenihan 2008). Moreover, as more individuals receive chemotherapy, it is not currently possible to differentiate to a desirable degree the progression of underlying cardiac disease from drug-induced cardiotoxicity. Therefore, much attention is currently focusing on biomarkers that may have predictive value in the early detection of cardiotoxicity. Christenson and colleagues (2015) commented that, at present, “there is no effective means of accurately detecting and predicting myocardial damage occurring with chemotherapy.” Biomarkers have the potential to fill this void, allowing for risk stratification of patients to distinguish those at increased susceptibility for side effects of therapy, in whom lower doses are warranted, from patients for whom more aggressive chemotherapeutic regimens can be entertained (Christenson and colleagues, 2015).
10.3.1 Cardiac Troponin
Circulating cardiac troponin (cTn) is the most widely used biomarker for detection of myocardial injury (Newby et al. 2011). With regard to its employment in the context of drug-induced cardiotoxicity, most data to date have examined anthracycline-based chemotherapy, and so the broader applicability of these data is currently uncertain (Christenson et al. 2015). However, a notable 2004 publication from the Expert Working Group on Biomarkers of Drug-induced Cardiac Toxicity (Wallace et al. 2004) reported that troponin I (cTnI) and troponin T (cTnT) are sensitive, specific, and robust biomarkers of drug-induced cardiotoxicity.
Blaes and colleagues (2015) reported a study examining the utility of various biomarkers, including high-sensitivity cTnT, in patients receiving anthracycline therapy to investigate whether baseline levels or changes were informative in the prediction of the onset of congestive heart failure. Elevations in baseline high-sensitivity cTnT were suggestive of a subgroup of oncology patients at high risk of developing drug-induced cardiotoxicity. Noting that the optimal treatment for drug-induced cardiotoxicity is prevention, the authors observed that “early detection with the use of biomarkers remains crucial in evaluating these patients, and in potentially introducing interventions to prevent these long-term complications” (Blaes et al. 2015).
10.4 Examples of the Cardiotoxicity of Oncologic Drugs
Table 10.1 provides some examples of cardiotoxicity information listed in drug labels of oncologic drugs and biologics. These examples include QT prolongation/torsades, hypertension, and cardiomyopathy, each of which is then discussed in more detail.
Table 10.1
Examples of cardiotoxicity information in drug labels
Drug (class) | Parameters mentioned in the “highlights of prescribing information” |
---|---|
Bevacizumab (vascular endothelial growth factor-specific angiogenesis inhibitor) | Arterial thromboembolic events, e.g., myocardial infarction, cerebral infarction, hypertension |
Doxorubicin (anthracycline topoisomerase inhibitor) | Acute left ventricular failure, heart failure, cardiomyopathy (note: information presented in Sect. 5.1 of the full prescribing information) Boxed warning: cardiomyopathy |
Lapatinib (kinase inhibitor) | QT prolongation possible in some patients, decreased left ventricular ejection fraction |
Nilotinib (kinase inhibitor) | QT prolongation, sudden deaths in patients with resistant or intolerant Ph + CML (ventricular repolarization abnormalities may have contributed to their occurrence) Boxed warning for QT prolongation and sudden deaths |
Pazopanib (kinase inhibitor) | QT prolongation and TdP, congestive heart failure and decreased left ventricular ejection fraction, hypertension, fatal hemorrhagic events, arterial thrombotic events |
Sorafenib (kinase inhibitor) | Hypertension, cardiac ischemia, and/or infarction may occur |
Sunitinib (kinase inhibitor) | QT prolongation and torsades, cardiac toxicity including left ventricular ejection fraction declines to below the lower limit of normal and cardiac failure including death, hypertension, hemorrhagic events |
Vandetanib (kinase inhibitor) | QT prolongation, torsades, and sudden death, heart failure, hemorrhage, hypertension Boxed warning for QT prolongation, torsades, and sudden death REMS in place |
10.4.1 QT Interval Prolongation/Torsades
QT interval prolongation and torsades are listed for several of the examples presented in Table 10.1. Of particular relevance here are earlier discussions in Sect. 7.6.1 addressing approaches taken when the standard TQT was not feasible, including cases where the test drug cannot ethically be given to healthy participants, the population that participates in TQT studies. Such a modified approach has been the case for oncologic drugs during the proarrhythmic cardiac safety regulatory landscape circa 2005–2015 discussed in Chap. 7. Going forward, if, as seems likely, the exposure–response methodology discussed in Chap. 8 assumes prominence in the preapproval clinical assessment of QT interval prolongation, one assumes the same caveat will apply. In cases where the potential therapeutic benefit of a drug intended to address a serious and unmet medical need is considered by regulators to outweigh the known cardiac risk (and the drug has an acceptable general safety profile), it will receive regulatory approval for marketing, albeit with the addition of a precautionary statement in the package insert, a black box warning, and/or requirements associated with a risk evaluation mitigation strategy (REMS) designed to make therapeutic employment of the drug as safe as is possible (the topic of REMS is addressed in Chap. 12). As an example presented in Table 10.1, this information is in the label for vandetanib, a kinase inhibitor indicated for the treatment of symptomatic or progressive medullary thyroid cancer in patients with unresectable, locally advanced or metastatic disease.
10.4.2 Hypertension
Epidemiological studies have suggested that an increase of 3 mmHg in blood pressure is associated with a 10–20 % increase in congestive heart failure (ALLHAT Collaborative Research Group 2000). Conversely, a 3–4 mmHg reduction in blood pressure in hypertensive individuals decreases risk of myocardial infarction by 22 % and of stroke by 33 % (Heart Outcomes Prevention Evaluation Study Investigators 2000). Currently, there is no consensus concerning the clinical significance of an off-target drug-induced increase in blood pressure of these magnitudes in healthy patients in whom the pressure remains within the normotensive range. Nonetheless, it is possible that small drug-induced blood pressure increases may have significant impact in patients with enhanced cardiovascular risk due to older age, cardiovascular comorbidities, or other traditional cardiovascular risk factors. Consequently, off-target drug-induced blood pressure elevations induced by multiple drug classes have increasingly attracted scientific interest (Grossman and Messerli 2012; O’Brien and Turner 2013; Sager et al. 2013), and this topic is therefore discussed in further detail in the following chapter. Here, attention focuses on blood pressure increases induced by oncologic agents.
Hypertension is the most common adverse effect of antivascular endothelial growth factor (anti-VEGF) agents that either bind to circulating VEGF or inhibit tyrosine kinases associated with VEGF receptors stimulated by VEGF proteins. This group includes VEGF binding agents such as the monoclonal antibody bevacizumab, the recombinant fusion protein aflibercept, and the multi-targeted kinase inhibitors (MTKIs), e.g., axitinib, pazopanib, sorafenib, sunitinib, and vandetanib. These VEGF-targeted therapies cause hypertension in 30–80 % of patients. Unlike traditional “off-target” side effects, hypertension is a mechanism-dependent, “on-target” toxicity, reflecting effective inhibition of the VEGF signaling pathway rather than nonspecific effects on unrelated signaling pathways (Robinson et al. 2010). The exact factors that predispose to VEGF inhibitor (VEGFI)-induced hypertension still remain to be established. However, risk factors that have been associated with VEGFI-induced hypertension include a previous history of hypertension, combination therapy with more than one anti-VEGFI, aged 65 years or older, smoking, and possibly high cholesterol (Small et al. 2014). The actual cause of elevated blood pressure is multifactorial, with decreased nitric oxide production, reduction in the density of microvascular beds, loss of antioxidative effect, reduced prostacyclin production, capillary rarefaction, and activation of the endothelin-1 system being suggested as possible mechanisms.
Hurwitz and colleagues (2004) investigated the safety of bevacizumab plus irinotecan, fluorouracil, and leucovorin for the treatment of metastatic colorectal cancer, reporting that severe hypertension (blood pressure >200/100 mmHg) was three to five times higher as compared with the placebo group. In an observational cohort study, the Bevacizumab Regimens’ Investigation of Treatment Effects (BRiTE) study, de novo hypertension was observed in 22 % of patients: 18.7 % of patients with pre-existing hypertension experienced worsening of the condition (Kozloff et al. 2009). Sorafenib, approved for advanced renal cell carcinoma and hepatocellular carcinoma, has been reported to increase mean systolic blood pressure by 8.2 mmHg and diastolic blood pressure by 6.5 mmHg within 24 h of treatment with 400 mg given twice a day: hypertension occurred in 23.4 % of patients on sorafenib, with severe hypertension seen in 5.7 % (Wu et al. 2008). Respective figures of 21.6 and 6.8 % have been reported for sunitinib (Zhu et al. 2009). Sunitinib is indicated for several conditions: gastrointestinal stromal tumor after disease progression or intolerance to imatinib mesylate, advanced renal cell carcinoma, and progressive, well-differentiated pancreatic neuroendocrine tumors in patients with unresectable locally advanced or metastatic disease.
10.4.3 Cardiomyopathy and Left Ventricular Dysfunction
Cardiomyopathies include a heterogeneous group of myocardial diseases associated with mechanical and/or electrical dysfunction. They usually exhibit inappropriate ventricular hypertrophy or dilatation and can be further classified into dilated, hypertrophic, restrictive, and arrhythmogenic right ventricular cardiomyopathy according to morphological and functional criteria (Thiene et al. 2008).
While several oncologic drugs have been associated with cardiomyopathy, anthracyclines such as daunorubicin and doxorubicin are salient examples. Anthracycline-induced cardiotoxicity may be defined as acute, early-onset chronic, and late-onset chronic (Cardinale et al. 2015). Acute cardiotoxicity occurs after a single dose, or a single course, of anthracyclines, and the onset of clinical manifestations is within 2 weeks from the end of treatment. Early-onset chronic toxicity develops within 1 year, and is the most frequent and clinically relevant form of cardiotoxicity. It usually presents as a dilated and hypokinetic cardiomyopathy leading to heart failure. Late-onset chronic cardiotoxicity develops years, or even decades, after the end of chemotherapy (Cardinale et al. 2015).
Acute cardiotoxicity is seen in approximately 1 % of cancer patients. The cardiotoxicity manifests as acute pericarditis/myocarditis. Characteristics include a transient decline in indices of myocardial contractility as seen by the employment of echocardiography and the combination of alterations in the ST segment and T-wave morphology in conjunction with prolongation of the QT interval as seen on the ECG. Early-onset chronic cardiotoxicity, which is dose dependent, has been reported in 1.6–2.1 % of patients: late-onset cardiomyopathy is observed in up to 5 % of patients (Curigliano et al. 2012).
Cardiotoxicity of oncologic drugs can be classified as irreversible (type I) or reversible (type II) dysfunction (Suter and Ewer 2013). Type I cardiotoxicity occurs due to cell loss which induces the progressive myocardial dysfunction classically seen with anthracyclines (Yusuf et al. 2011). Type II cardiotoxicity results from cellular dysfunction that is usually reversible and associated with normalization of cardiovascular function on completion of therapy. Anti-human epidermal growth factor receptor 2 (HER2) agents (e.g., pertuzumab, lapatinib, ado-trastuzumab) typically cause type II cardiotoxicity. Trastuzumab usually produces type II toxicity but may cause type I toxicity in patients with pre-existing heart disease or prior anthracycline therapy (Curigliano et al. 2012). Trastuzumab use typically results in small to modest risk for cardiotoxicity, which is typically manifested by an asymptomatic decrease in left ventricular ejection fraction and less often by clinical heart failure. Although limited, available data for pertuzumab and ado-trastuzumab support the view that they may be less cardiotoxic than trastuzumab.
Establishing the diagnosis of cardiomyopathy in patients undergoing active cancer treatment is often challenging, as common symptoms and signs such as fatigue, dyspnea, increased jugular venous distension, and lung crepitations may be due to other causes (Ferri et al. 2013). Useful noninvasive diagnostic modalities for diagnosing chemotherapy-induced cardiomyopathy include echocardiography, radionuclide ventriculography, multiple-gated acquisition computed tomography scans, and magnetic resonance imaging scans (Raschi et al. 2010; Yusuf et al. 2011). Since some drug-induced cardiomyopathy is irreversible, the employment of biomarkers may help identify myocardial damage prior to a decline in left ventricular ejection fraction (Gottdiener et al. 2004). Increased serum levels of cardiac troponin can help identify patients who may subsequently develop a reduction in LVEF (Florea and Anand 2012).
10.5 Therapeutic Management
Mounting evidence suggests that trastuzumab, especially when given in combination with anthracyclines, has been associated with both asymptomatic and symptomatic left ventricular dysfunctions that can lead to premature discontinuation of trastuzumab therapy and significant cardiac morbidity. Seicean and colleagues (2013) retrospectively evaluated the incidence of new symptomatic heart failure in patients with breast cancer treated with anthracyclines, trastuzumab, or both, at an academic medical center between 2005 and 2010. Using 1:2 propensity matching, patients on continuous β-blockers during cancer treatment were compared with those not on β-blockade. The effect of incidental β-blocker use on new symptomatic heart failure and noncardiac mortality was assessed during a median follow-up of approximately 3 years. Results showed that trastuzumab, when given alone or in combination with anthracyclines, substantially increased the risk of symptomatic heart failure compared with anthracyclines alone. Furthermore, incidental β-blocker use significantly reduced the incidence of symptomatic heart failure, but did not affect noncardiac mortality in patients with breast cancer treated with trastuzumab, anthracyclines, or both.
10.5.1 Strategies for the Prevention of Anthracycline- and Trastuzumab-Induced Cardiotoxicity
Current strategies to prevent cardiac toxicity include limiting the cumulative anthracycline dose, prolonging infusion times to limit peak serum concentrations of anthracyclines, using liposomal formulations of anthracyclines, administering anthracyclines and trastuzumab sequentially rather than concurrently, and using non-anthracycline-based chemotherapy regimens for the treatment of HER2+ breast cancer. However, despite these attempts, cardiotoxicity remains prevalent. The iron chelator dexrazoxane is the only drug currently approved to prevent anthracycline-induced cardiomyopathy. However, its use in clinical practice is limited by concerns of myelosuppression and reduced tumor response rates. Beta-adrenergic antagonists have also been added to this list of promising agents (Seicean et al. 2013).
Further evidence for the emerging role of prophylactic β-blocker therapy for mitigating the effect of trastuzumab on left ventricular function comes from the recently reported results of the Multidisciplinary Approach to Novel Therapies in Cardiology Oncology Research (MANTICORE) trial (Pituskin 2015). This Phase III trial was designed to determine whether prophylactic use of a β-blocker or an ACE inhibitor (two drugs used to treat established cardiovascular disease) could reduce trastuzumab-associated cardiotoxicity in patients with HER2-positive early breast cancer. At baseline, all patients had a left ventricular ejection fraction ≥ 50 %. Patients were randomized to receive bisoprolol, perindopril, or placebo during trastuzumab-based chemotherapy for 24 months. Cardiac magnetic resonance imaging parameters (see Sect. 10.4.2) were assessed at baseline, at 3 months, at 12 months, and at 24 months. Mean age at baseline was approximately 50 years. Bisoprolol significantly prevented reduction from baseline in left ventricular ejection fraction vs. placebo and also prevented trastuzumab interruptions due to a drop in left ventricular ejection fraction. However, since it did not prevent left ventricular remodeling (as measured by left ventricular end diastolic volume) associated with trastuzumab therapy, more research is needed on optimal cardioprotection for patients receiving trastuzumab.
10.5.2 Therapeutic Management of Hypertension
As more cancer patients are treated with VEGFIs, and more potent VEGFIs are developed, the burden of hypertension toxicity will increase. This will be further compounded as the use of antiangiogenic drugs broadens to include older patients and those with cardiovascular risk factors and pre-existing hypertension (Small et al. 2014). While there are currently no widely accepted guidelines addressing VEGFI-induced hypertension, several authors have addressed this issue.
Steingart and colleagues (2012) reported recommendations for physicians from the Cardiovascular Toxicities Panel of the National Cancer Institute (NCI). These included the following:
Conduct and document a formal risk assessment for existing cardiovascular disease and potential cardiovascular complications before starting VEGFI treatment, recognizing that pre-existing hypertension and cardiovascular disease are common in patients with cancer.
Actively monitor for blood pressure elevations and cardiac toxicity with more frequent assessments during the first VEGFI treatment cycle.
Aggressively manage blood pressure elevations and early symptoms and signs of cardiac toxicity to prevent clinically limiting complications of VEGFI therapy.
Small and colleagues (2014) noted that in the absence of specific guidelines, angiotensin-converting enzyme inhibitors and dihydropyridine calcium channel blockers (e.g., amlodipine, nifedipine) are commonly used as antihypertensive agents in this setting. Their review concluded that “expert opinion recommends that patients be fully assessed for hypertension and cardiovascular disease before VEGFI treatment, that blood pressure is monitored frequently, and that hypertension is aggressively treated to target (<140/90 mmHg)” (Small et al. 2014).
Rutkowski and Stepniak (2016) focused on regorafenib, a multi-targeted inhibitor with activity against multiple kinases that has demonstrated clinical benefit in gastrointestinal stromal tumor (GIST) patients after progression on prior treatment with at least imatinib/sunitinib, currently the approved standard third-line option in therapy of advanced GIST. They provided expert opinion on the management of several adverse events, including liver toxicity and hypertension. Management strategies for hypertension included dose reduction and the use of antihypertensive agents and, if necessary, cessation of therapy. They also noted that “Patients should be counseled on the risks of serious adverse events associated with regorafenib and advice should be given to health care providers on patient monitoring” (Rutkowski and Stępniak 2016).
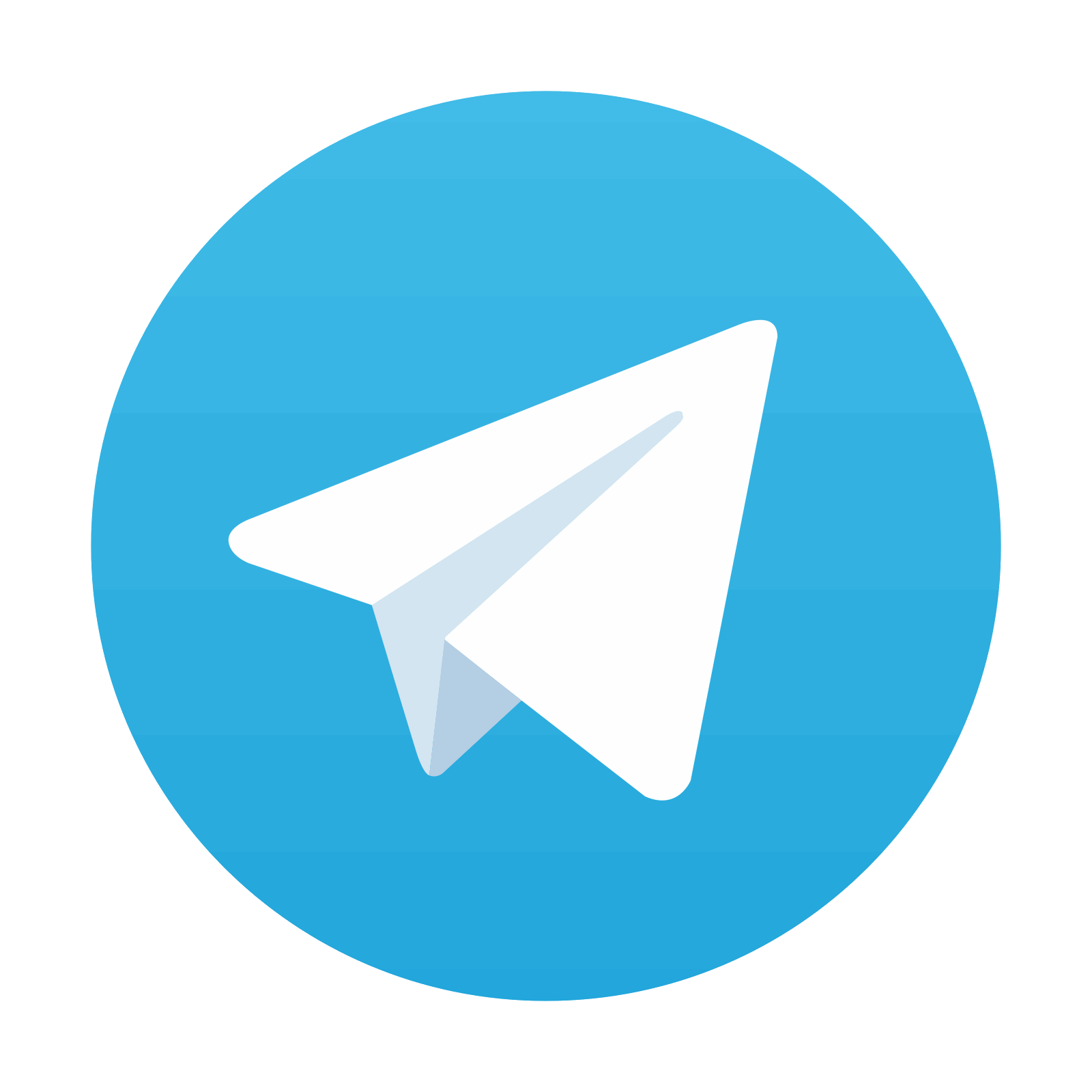
Stay updated, free articles. Join our Telegram channel

Full access? Get Clinical Tree
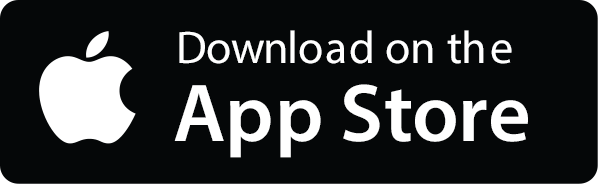
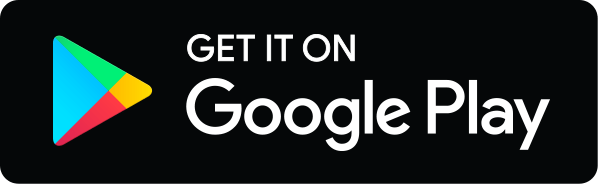