23
Office workers and teachers*
In contrast to blue collar industrial workers, white collar workers (including office workers and teachers) are often thought to work in nonhazardous environments. In fact, a long history exists of concerns for office and school worker health in relation to large buildings that require mechanical ventilation and air conditioning. In the early decades of the twentieth century, ventilation standards were developed to provide for control of body odor in indoor environments where large numbers of people congregated. As early as the 1920s, ventilation parameters were established to control transmission of infectious diseases. Public interest in indoor environmental quality arose in the late 1970s after changes in building environments and their ventilation occurred in the wake of the energy crisis in the USA. Office workers in diverse parts of the USA had symptoms of headache, mucous membrane irritation and difficulty concentrating in tight temporal association with occupancy of their work buildings. The public health response to investigation was stymied when industrial hygienists failed to measure any contaminant at levels exceeding industrial health standards. Some public health professionals attributed widespread complaints in particular buildings to mass psychogenic illness, although the type of symptoms and endemic nature of complaints did not meet criteria for hysteria. European investigators took an epidemiologic approach and established building-related risk factors for the common symptoms of mucous membrane irritation and discomfort. These included air conditioning, extent of carpet and fleecy materials such as textile-covered partitions and chairs, open shelving with paper in the indoor environment, housekeeping and eventually outdoor air ventilation rate and ventilation maintenance practices, among others.
Indoor air quality consultants incorporated these observations into their recommendations for ventilation rates and maintenance, and the complaints of nonspecific sick building syndrome symptoms alone decreased in the 1990s. In their place, however, the proportion of building-related chest symptoms have increased relentlessly, at least as reflected in the number of worker requests for health hazard evaluations (http://www.cdc.gov/niosh/hhe/HHEprogram.xhtml) for indoor air quality concerns submitted to the US National Institute for Occupational Safety and Health (NIOSH). These chest symptoms often arise in buildings with a history of dampness. As this chapter will explore, abundant evidence now exists that indicates environments in offices and schools can lead to asthma, hypersensitivity pneumonitis, rhinosinusitis and perhaps even sarcoidosis. In addition, the traditional tools of industrial exposure assessment of chemicals, particulates, bioaerosols and ventilation have roles in understanding the emerging hazards of offices and schools.
In the USA almost 70% of the workforce is employed in nonindustrial, indoor settings. Office and administrative support occupations accounted for an estimated 20 million workers in the US census in 2000, and management, business and financial operations occupations accounted for an additional 16.6 million. In 2006, the US census bureau estimated that there were 6.8 million school teachers. Schools also employ nonteaching staff with work environments similar to those of teachers.
In keeping with the historical view that office workers have few occupational risks, this group is commonly used as the comparison group in analyses looking for occupational risk of respiratory conditions. Population-based information on health risks to office workers is meager. A study of middle-aged professional women in France documented that, in comparison to work in naturally ventilated buildings, work in buildings with heating, ventilation and air-conditioning (HVAC) systems was associated with an increased risk for annual visits to otorhinolaryngologists, as well as sickness absences. These observations hint that occupational respiratory disease occurs in the indoor environment and may present in pulmonary and occupational medicine practices.
Using office workers as a comparison group, an analysis of 20,991 US adults, 18 years of age and older who participated in the 2001 National Health Interview survey, found that workers in elementary and secondary schools and colleges had an increased risk for asthma. It is of interest that from 1990 to 1999, US secondary school teachers had excess proportionate mortality from asthma as compared with the expected based on all occupations. In state-based reporting of physician-diagnosed work-related asthma (WRA) from 1993 to 1995, 1101 cases were identified in California, Massachusetts, Michigan and New Jersey. Indoor air pollutants (including those arising from poor ventilation, pesticides, dusts and dirt, molds, environmental tobacco smoke, paint odors and other nonspecific building odors) were reported as a cause of both new-onset and work-aggravated asthma in all four states and represented the most frequent putative cause. Nonindustrial workers reportedly affected by indoor air pollutants included teachers, office workers, nurses, secretaries, librarians, computer operators and programmers, technicians and clerks. A subsequent analysis of 2995 WRA cases reported between 1993 and 2000 from the same four states found that 265 (9%) WRA cases were employed in educational services, of which 69% (182/265) were new-onset asthma. Indoor air pollutants, mold, dusts and cleaning products were the most frequently reported agents. There is some evidence that respiratory conditions other than asthma are found in excess in teachers. Teachers had excess work-related eye, nose and throat symptoms, wheezing and five or more episodes of cold or flu in the past year over other working women. Some of these health effects may have been related to exposure to children with infectious conditions. A large case-control study of sarcoidosis in the USA started in the mid 1990s (the ACCESS study) found that elementary and secondary school teachers were at an elevated risk for sarcoidosis.
In this chapter, we concentrate on indoor environmental quality and conditions that are commonly associated with office worker and teacher concerns with respect to respiratory disorders (Table 23.1). Chief among these are dampness-related exposures and conditions. In our experience with worker requests for assistance with indoor quality issues, renovation-associated symptoms are commonly reported by office workers and teachers. Finally work activities in office buildings and schools generate a host of exposures which may be implicated in occupational respiratory disease.
Table 23.1 Indoor environmental conditions pertinent to respiratory diseases
aOpportunistic infections particularly in immuno-compromised individuals.
bLegionnaire’s disease, Pontiac fever.
cHistoplasmosis.
In this chapter we do not cover environmental tobacco smoke, cosmetic fragrances, asbestos, man-made fibers and indoor exposures to radon gas.
23.2 Exposures in office buildings and schools
There are a variety of environmental conditions leading to microbial, chemical and particulate contamination associated with work-related respiratory conditions in office workers and teachers (Table 23.1). In describing the contaminants, their sources and exposure pathways, we concentrate on issues related to building dampness, ventilation systems and renovation activities.
Damp building conditions can result from both external and internal sources of water (Figure 23.1). External leaks can occur through roofs, windows, exterior walls and foundations. Internal leaks occur when building water-pipe or plumbing systems fail. Condensation and high humidity are also sources of building dampness. Many design and maintenance issues in office buildings and schools can lead to dampness problems, e.g. flat roofs, curtain walls, either no moisture barriers for walls or foundations or incorrect placement and installation of the moisture barriers, poor installation of insulation, flashing and caulking of windows and roofs, incorrect grading around the building foundation, lack of maintenance of mechanical ventilation systems, and inattention to repair of the building. Damp building conditions can result in both biological and chemical exposures to occupants.
Heating, ventilation and air-conditioning systems are meant to supply adequate outside air to building occupants as well as conditioning the air (such as heating, cooling, humidity control and particulate filtration). For office buildings and schools, outside air ventilation rates are usually adequate to dilute body odors but are not in general set to dilute other possible air contaminants. There are a range of recommended minimum ventilation rates per person depending on the type of facility and room function. Contaminants from outside the building may be entrained into the HVAC via air intakes and can lead to poor indoor air quality (Table 23.2). Diesel school buses may park and idle their engines close to school buildings, leading to diesel exhaust exposures inside the schools. Commonly, outside air intakes on roofs are too close to building exhaust vents such as those from the bathrooms, boiler room, kitchens or laboratories. The HVAC system itself may become contaminated and circulate these contaminants throughout the building. In such situations, increasing ventilation rates can increase occupants’ exposures. When the building (other than the HVAC system) has internal sources of microbial, chemical or particulate contamination, the HVAC may increase occupant exposures by circulating these contaminants throughout the building. The spreading of influenza viruses within office buildings and schools is of special importance with the possibility of pandemics.
Table 23.2 Types of contaminants circulated by ventilation systems throughout a building
|
In our studies of indoor environmental quality we have found that renovation activities in general or remediation activities related to dampness and mold are frequently carried out without relocation of the building occupants and without adequate containment, thus leading to occupant exposures (Table 23.3). Some of the exposures are construction dusts, volatile organic compounds (VOCs) from solvents, paints and glues, and microbial agents from contaminated materials.
In both office buildings and schools, day-to-day work activities such as handling of paper, the use of carbonless copy paper, printing and copying can generate particulate and VOC exposures (Table 23.4). Housekeeping practices can resuspend carpet dust which may have accumulated microbial particulates and detergent dust. Pest management practices can lead to pesticide exposures, and chemicals used in art, craft and laboratory classes can cause exposures.
Table 23.4 Exposures related to work activities in office buildings and schools
aVolatile organic compounds.
23.2.2 Dampness and associated exposures
The little information available on the proportion of office or school buildings in the USA that have dampness problems, or the severity of such conditions, indicates that the problem is widespread. From a large study of US office buildings not known to have indoor air quality complaints in the 1990s, it was found that 85% of 100 buildings had incurred some past water damage, while 43% of the buildings had current water damage. In 1995, the Government Accounting Office reported that about 30% of schools in the USA needed repairs to plumbing, roofs or external walls, windows and doors. Individual buildings can become damp indoor environments through being poorly designed, poorly built, poorly maintained or poorly run.
Since the late 1990s, three influential literature reviews (two from Europe and one from the USA) have concluded that living, working or going to school in damp indoor environments poses a respiratory health threat. Despite the absence of a standardized way to describe levels of dampness in buildings, studies have shown an increasing risk for respiratory symptoms in occupants with increases in the number of signs of dampness, water incursions and water damaged materials, both as reported by occupants or as scored by researchers. A NIOSH study of college employees demonstrated that exposure to dampness, as estimated by weighting the dampness score of individual rooms by employees’ time spent in the specific rooms, was a good predictor of employees’ respiratory illnesses. The dampness scores were calculated based on the extent of water stains, visible mold, mold odor and moisture observed by industrial hygienists in seven room components (walls, ceiling, floor, windows, ventilation system, furniture and pipes). A Finnish research group has also developed a moisture damage index based on a study of homes. The index was based on the location of damage, type of damage, duration, type of damaged material and size of damaged area, as measured by civil engineers. They also demonstrated that this index was correlated with health symptoms.
Dampness itself cannot be a cause of respiratory disease, and there is still uncertainty about the causal agents of respiratory problems related to dampness. However, there is growing epidemiological evidence of associations between respiratory health effects and microbial contaminants derived from mold and bacteria, allergens from dust mites and cockroaches, and chemical agents released from wetted building materials and furnishings.
Molds
Growth of molds in a building is directly related to dampness. If mold odors occur, whether or not there is visible mold, it is not necessary to do air sampling for mold spores. Rather, appropriate remediation to mitigate sources of water or moisture should be carried out. Remediation guidelines for moldy environments can be found in the following web page of US Department of Labor: http://www.osha.gov/dts/shib/shib101003.xhtml.
Traditional air sampling for molds is highly variable. The results from such methods may not accurately indicate either a patient’s exposure or risk of illness. Air sampling methods using culture media or sticky slides collect small amounts of air due to potential overloading of mold spores on the media or slides. Thus, they are not likely to capture the inherently large temporal and spatial variability of airborne mold concentrations unless many samples are collected at different times at the same location and also at multiple locations simultaneously. The culture method only supports growth of viable spores which may represent as little as 1% of the total mold. Despite these limitations, many environmental consultants still rely on air sampling methods as described above in investigating damp/moldy environments.
Many research groups use measurements of mold in floor, chair or other surface dust, which may better represent historical exposure to mold. Although the dust measurement does not directly represent inhalation exposure, research findings have demonstrated that dust measurements are useful in epidemiologic associations of health effects and mold in damp indoor environments. Several research groups have also used measurements of ergosterol (a principal sterol in the fungal membrane) in environmental samples for exposure assessment of mold. Although ergosterol does not provide information on mold species, it may be a useful method to assess exposure to total fungal biomass since it measures both viable and nonviable spores and fungal fragments. Additionally, measurements of (1 → 3)-β-D-glucan (a major carbohydrate constituent of fungal cell walls), extracellular polysaccharide (a carbohydrate in fungal cell walls) of Penicillium and Aspergillus species and microbial volatile organic compounds (MVOCs), and PCR (polymerase chain reaction) analysis of fungal DNA or RNA have been explored for mold exposure assessment.
Table 23.5 shows evidence of associations between physician-diagnosed asthma or respiratory symptoms and molds from recent studies. Currently, there are no standards for what level or what species of molds constitute a health risk. Thus, there are no known safe levels of exposure. For example, all molds have the potential to be allergenic, but there is a lack of knowledge on the antigens or allergens found in the vast majority of molds.
Table 23.5 Recent epidemiological studies investigating an association between microbial agents and asthma and respiratory symptoms in offices or schools
Odds ratios in bold are significant at p ≤0.05. Underlined odds ratios are marginally significant at 0.05 < p ≤ 0.10.
Abbreviations for microbial agents: a = airborne, fd = floor dust, cd = chair dust, erg = ergosterol, MVOC = microbial volatile organic compound. Abbreviations for specific fungi: hyd = hydrophilic fungi, Asp = Aspergillus spp., Zyg = Zygomycetes, Bot = Botrytis spp., Pen = Penicillium spp., Ulo = Ulocladium spp., Dre = Drechslera spp.
aPark, J.H., Cox-Ganser, J.M., Kreiss, K., White, S.K., Rao C.Y. (2008) Hydrophilic fungi and ergosterol associated with respiratory illness in a water-damaged building. Environ. Health Perspect. 116(1): 45-50.
bPark, J.H., Cox-Ganser, J., Rao, C., Kreiss, K. (2006) Fungal and endotoxin measurements in dust associated with respiratory symptoms in a water-damaged office building. Indoor Air 16(3): 192-203.
cKim, J.L., Elfman, L., Mi, Y., Wieslander, G., Smedje, G., Norback, D. (2007) Indoor molds, bacteria, microbial volatile organic compounds and plasticizers in schools – associations with asthma and respiratory symptoms in pupils. Indoor Air 17(2): 153-163.
dChao, H.J., Schwartz, J., Milton, D.K., Burge, H.A. (2003) The work environment and workers’ health in four large office buildings. Environ. Health Perspect. 111(9): 1242-1248.
eORs are for IQR (interquartile range) increase (CFU/g dust) in exposure;
fORs are for highest tertile compared with lowest tertile exposure group;
gORs are for each 102/m3 increase of bacteria or mold, and 1 μg/m3 increase of total MVOC.
Existing recommendations for what constitutes low or high levels of microbial contamination are not consistent and are not based on exposure-health response relationships. Approaches recommended by the American Industrial Hygiene Association are to use the ratio of counts of indoor to outdoor fungi as a measure of possible indoor fungal contamination (ratios greater than one indicate possible problems); the use of rank order of fungal species indoors compared with outdoors to suggest indoor sources of microbial amplification. There is also a new approach to identify buildings with mold contamination, developed at the US Environmental Protection Agency, which uses PCR analysis of fungal species in dust to calculate a relative moldiness index value based on typical fungal species found in water-damaged and nonwater-damaged buildings. A recent NIOSH study of a water-damaged office building indicated that water-loving (hydrophilic) fungi in dust were associated with respiratory health effects in office workers. A list of fungal species that have been associated with damp building conditions is given in Table 23.6.
Table 23.6 Identification of the species of fungi from samples may indicate the dampness conditions of buildings (AIHA, Field Guide for the Determination of Biological Contaminants in Environmental Samples, 2005)
Chronic wet conditions | Alternating wet and dry conditions |
Acremonium strictum | Aspergillus fumigatus |
Alternaria alternata | A. ochraceus |
Aspergillus niger | Cladosporium spp. |
Botrytis cinerea | Epicoccum nigrum |
Chaetomium globosum | Fusarium oxysporum |
Doratomyces spp. | F. solani |
Gliocladium roseum | Paecilomyces variotii |
Graphium spp. | Penicillium aurantiogriseum |
Mucor racemosus | P. brevicompactum |
Phoma spp. | P. chrysogenum |
Rhizopus spp. | P. commune |
Sistotrema brinkmanii | P. viridicatum |
Stachybotrys chartarum | Scopuariopsis brevicaularis |
Trichoderma spp. | S. candida |
Verticillium spp. | Ulocladium chartarum |
Yeasts |
Bacteria
Some bacteria have been associated with damp indoor environments. These include Gram-negative bacteria, actinomycetes and nontuberculous mycobacteria. A 2006 Finnish study of visibly damaged building materials from 34 water-damaged buildings found actinomycetes in 24% of 75 samples at concentrations ranging from less than 45 to 8.0 × 106 colony forming units per gram (CFU/g) of dust and mycobacteria in 23% of 88 samples at concentrations ranging from 60 to 2.0 × 107 CFU/g. A variety of species of mycobacteria were found in the water-damaged materials and the proportion of samples positive for mycobacteria increased with increasing concentrations of fungi.
Endotoxin is the biologically active lipopolysaccharide found in cell walls of Gram-negative bacteria, and has been associated with asthma and respiratory symptoms. Endotoxin is ubiquitous because Gram-negative bacteria are present everywhere. To determine exposure to environmental endotoxin, biological activity of lipopolysaccharide, expressed in endotoxin units (EU), is measured using an extract of the blood of Limulus (the horseshoe crab). In normal conditions with no internal bacterial contamination, the indoor air level of endotoxin should not be higher than outdoors. Typically, the outdoor air endotoxin level changes significantly across seasons, showing higher levels in the growing season (around 1-3 EU/m3), due to aerosolized Gramnegative bacteria from leaves, than during winter (around 0.2 EU/m3). No health-based standard for endotoxin levels exists. However, the American Conference of Governmental Industrial Hygienists (ACGIH) (Bioaerosols: Assessment and Control) proposed that appropriate action should be taken when the level of endotoxin in the indoor environment (in the presence of respiratory symptoms) is at least 10 times higher than background outdoor levels.
Similar to mold sampling, the traditional minutes-long bacterial air sampling methods using culture media may give results that represent less than 1% of the total bacterial flora present. Thus, this method may significantly underestimate exposure to environmental bacteria. Bacterial biomass measurement using muramic acid (a sugar in the peptidoglycan layer of Gram-positive and -negative bacterial cell walls) has also been developed for exposure assessment of environmental bacteria. There are few studies which have investigated airborne bacterial levels in office and school buildings. A research study reported that the level of indoor airborne bacteria was 105 CFU/m3 in office building environments.
23.2.3 Chemicals released from building materials/furnishings and growing microbes
Volatile organic compounds are defined as organic compounds with boiling points in the range of 50-250°C. In indoor environments of schools and offices, there are many sources of VOCs, such as building materials (especially in new buildings), furniture, plastics, floor wax, cleaning agents, occupants and photocopiers. There are several thousand VOCs, and over 900 VOCs have been identified in indoor environments. Acetone, aliphatic hydrocarbons (hexane, octane, decane, etc.), aromatic hydrocarbons (toluene, xylene, benzene, etc.), chlorinated solvents (methylene chloride, trichloroethane, etc.), n-butyl acetate, dichlorobenzene, 4-phenylcyclohexene and terpenes are among the most commonly found VOCs. Since measurement and identification of an individual VOC with very low concentration is expensive, total VOCs are frequently measured. There is no standard for total VOCs in the USA and Canada, nor are consensus guidelines available from the American Society of Heating, Refrigerating, and Air Conditioning Engineers (ASHRAE).
Microbial volatile organic compounds can be produced while microorganisms, especially mold, actively grow in damp building materials. In laboratory studies, more than 200 VOCs have been recognized as having microbial origins. Recently, the American Industrial Hygiene Association’s Field Guideline for the Determination of Biological Contaminants in Environmental Samples identified 14 compounds as potential MVOCs (3-methyl furan, 1-butanol, 3-methyl-1-butanol, 3-methyl-2-butanol, 2-pentanol, 2-hexanone, 2-heptanone, 3-octanone, 3-octanol, 1-octen-3-ol, 2-octen-1-ol, 2-nonanone, borneol and geosmin), which were consistently detected by more than three authors in field surveys. Unique musty, earthy, mushroom-like or mold odors in damp/mold buildings are due to several MVOCs (e.g. geosmin, 2-methyl-iso-borneol or 8-carbon MVOCs). Many researchers in epidemiological studies have successfully used mold odor as a useful predictor for adverse health effects in damp indoor environments. However, no MVOC measurements have been shown to be predictive of health risk.
Formaldehyde, a well-known irritant and sensitizer, is a colorless gas and can be recognized by pungent odor at a concentration higher than 0.2 parts per million (ppm). Building materials and furnishings (e.g. particle board, carpets and fabrics), cleaning agents and adhesives can release formaldehyde. Irritant effects have been found to occur when formaldehyde is present at levels above 0.1 ppm. Studies in schools have reported levels generally at 0.05 ppm or less, but some concentrations above the irritancy threshold were found. A study of Italian office workers with symptoms of irritation while working in newly renovated offices, found levels of formaldehyde from 0.07 to 0.4 ppm. The ACGIH classified formaldehyde as a ‘suspected human carcinogen’ with a ceiling threshold limit value of 0.3 ppm. NIOSH also identified formaldehyde as a potential occupational carcinogen and recommended a time-weighted average (up to a 10 hour workday and a 40 hour working week) exposure limit of 0.016 ppm and a 15 minute-average ceiling limit of 0.1 ppm.
Phthalates are plasticizers added to polyvinyl chloride (PVC) products to keep them pliable, as well as to a number of other products such as paints, glues, cleaners and cosmetics. They are ubiquitous in the environment. There are public concerns about the health effects of phthalates, since they may act as endocrine disruptors and can affect the reproductive system. Recent research on children in homes and schools has indicated that phthalates and other plasticizers and their breakdown products are associated with respiratory symptoms, asthma, rhinitis and eczema. Moisture can lead to increased release and breakdown of plasticizers from PVC-containing building materials such as floor tiles and carpet backing; thus increased exposure to phthalates in damp, water-damaged schools and offices may be a concern. More research is needed to understand exposure-response relationships between phthalates and respiratory health effects.
A 2000 Institute of Medicine (IOM) report on asthma and indoor air exposures found sufficient evidence of a causal relationship between the development of asthma and house dust mite, limited or suggestive evidence of an association between the development of asthma and exposure to cockroach in preschool-aged children, and insufficient evidence to make a conclusion on the development of asthma and exposure to cockroach (in people other than preschool-aged children), cat, dog or rodents. In regards to exacerbation of asthma, the IOM committee found sufficient evidence of a causal relationship with exposure to cat, cockroach and house dust mite, sufficient evidence of an association with exposure to dog, and insufficient evidence to make a conclusion regarding exposure to rodents.
Dust mites thrive in warm moist places, and keeping relative humidity below 50% is a recommendation for reducing dust mites and their allergen levels. The dust-mites commonly found in indoor environments such as homes, schools and office buildings are Dermatophagoides farinae (Der f) and Dermatophagoides pteronyssinus (Der p). Their Group 1 allergens, Der f 1 and Der p 1, are often assessed for associations with allergy and asthma. There is a dose-response relationship between sensitization and exposure to dust mite allergen. Research on children has indicated that exposure to 2 μg/g or more of Der p 1 allergens in dust increases the risk of sensitization (as measured by positive skin tests or presence of IgE antibodies), and the development of asthma, although there is some evidence that sensitization can occur at lower levels. Exposure to 10 μg/g Der p 1 or more in dust increases the risk of asthma symptoms in mite-allergic people, but the relationship is not clear. Concentrations of Der f 1 and Der p 1 found in office building and school building dusts are usually lower than the levels found in dust from homes. Furthermore, the opportunity for exposure to dust-mite allergens may be lower in nonresidential buildings since allergens are contained in particles of mite feces which do not remain suspended in the air for long if disturbed. In homes, bedding is a major source of mite allergen exposure.
Cat allergens are ubiquitous, being found in homes with and without cats and in many types of public buildings, including schools and office buildings. They are sticky and are carried into buildings on clothing. There is a proposed threshold of 8 μg/g of the major cat allergen Fel d 1 for sensitization, while a level of 1 μg/g has been reported to elicit asthma symptoms in sensitized people. The allergens are carried on a range of particle sizes, but are often on small particles that can remain airborne for long periods of time after dust has been disturbed.
There are many species of cockroaches, but the German cockroach Blattella germanica (Bla g) is commonly found in homes and schools, and to a more limited extend in office buildings. Its major allergens Bla g 2 and Bla g 1 have been widely used to assess exposure to cockroaches in relation to asthma. The proposed threshold levels of cockroach exposure is 2 units per gram (U/g) allergen in dust for an increased risk of sensitization and 8 U/g of allergen in dust for asthma symptoms. Cockroach allergens are usually carried on larger particles and thus do not remain suspended in the air for long after disturbance of dust.
Most work on exposures to indoor animal allergens has been done in homes, and the levels of allergens in office buildings and schools are generally lower than residential exposures. Nevertheless, for some proportion of the samples, animal allergen levels in dust at or above threshold levels for sensitization and symptoms (proposed for residential settings) do occur in office buildings and schools. Furthermore, dust mite and cat allergens can accumulate in carpets and upholstered furniture. A 2005 review of indoor allergens in settled dust in schools concluded that from a clinical perspective the school environment is important for allergic occupants, especially if attempts have been made to reduce allergen exposures in their home environment. Examples of levels of dust mite, cat and cockroach allergens that have been found in offices and schools are given in Table 23.7.
Table 23.7 Studies investigating levels of dust mite, cat and cockroach allergens in dust in offices or schools
aThreshold level for sensitization to dust mites and the development of asthma.
bMajor risk factor for the development of acute asthma in mite-allergic individuals. cThreshold level for sensitization to cat allergen and the development of asthma. dThreshold level for symptoms.
eDetectable Der p 1 levels found in 54% of workplaces and detectable Der f1 levels found in 55% of workplaces.
fDetectable Fel d 1 levels found in 54% of workplaces.
gDetectable Der p 1 levels found in 70% of workplaces and detectable Der f1 levels found in 63% of workplaces.
hDetectable Fel d 1 levels found in 99% of workplaces.
iDetectable Bla g1 levels found in 69% of samples of dust from schools.
jSensitization threshold levels for dust mite, cat and cockroach allergens were exceeded in many schoolrooms.
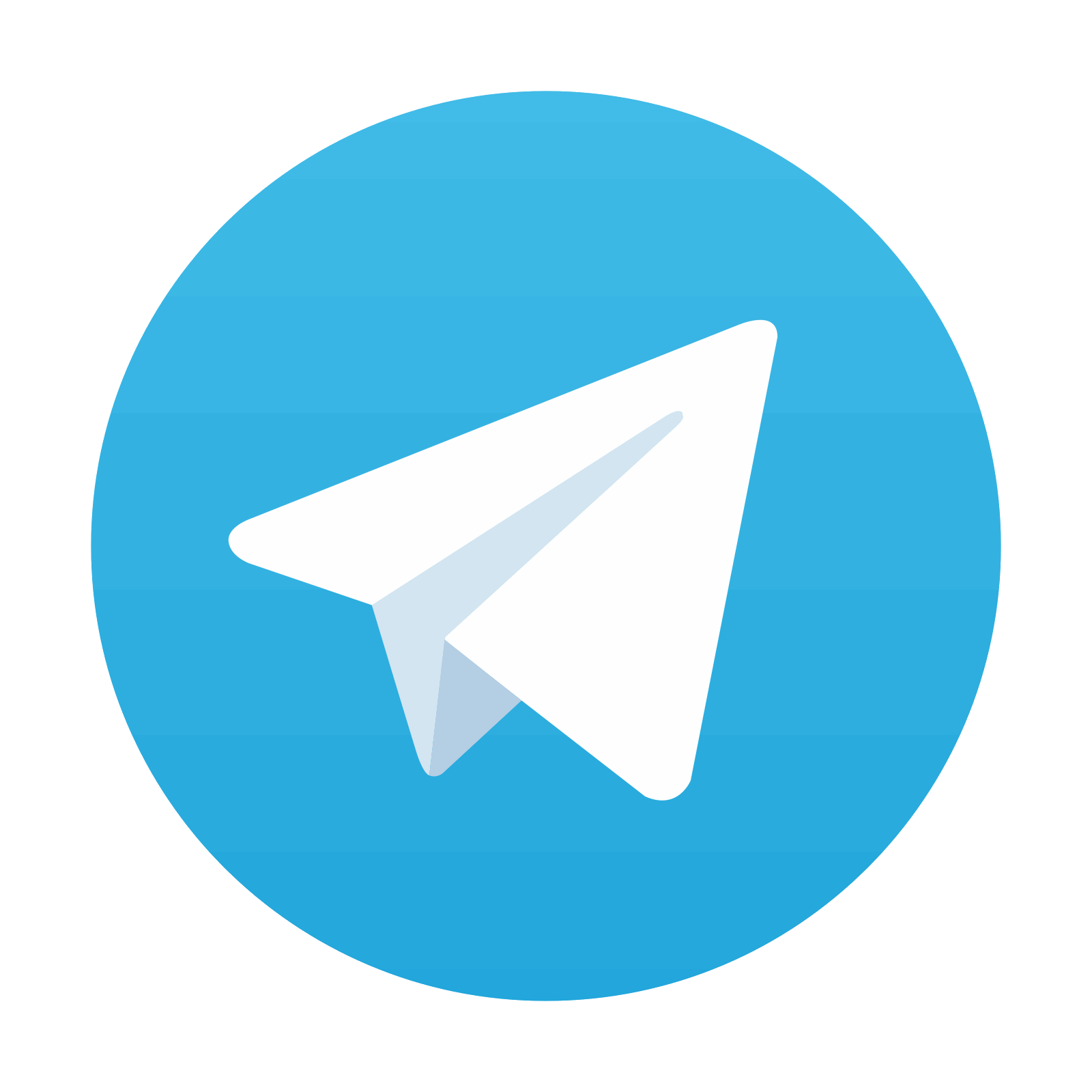
Stay updated, free articles. Join our Telegram channel

Full access? Get Clinical Tree
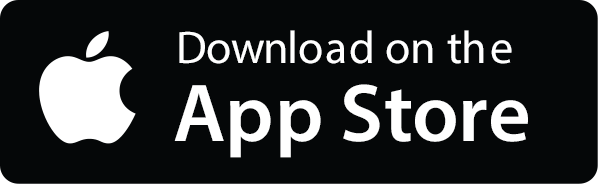
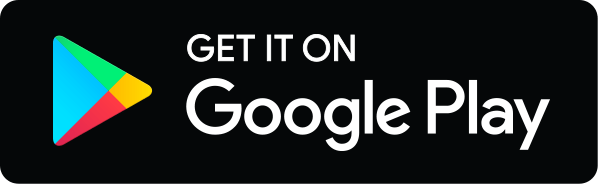