CME
Relationship of Central Pattern Generators with Parasomnias and Sleep-Related Epileptic Seizures
Keywords
• Central pattern generators • Parasomnias • Sleep-related epileptic seizures • Arousal
In 1911, Graham Brown1 was the first to show the existence of neuronal networks within the thoracic spinal cord capable of producing repetitive rhythmic hind limb movements resembling locomotion. He reported that rhythmic alternating locomotorlike motor movements were observed in antagonistic muscles in each hind limb for a short time after experimental thoracic spinal cord transection in an animal model that had previously had its dorsal sensory roots sectioned.1 These experiments unequivocally showed that neuronal aggregates in the spinal cord (deprived from sensory inputs and supraspinal influences) can generate a coordinated rhythmic motor movement.
Since then, a wealth of molecular, genetic, and neuroimaging studies have confirmed that such autonomous motor neural networks (now called central pattern generators [CPGs]) are present in all animals. CPGs are located in the midbrain, pons, and vertebrate spinal cord. They are genetically determined neural networks (circuits) that are capable of producing self-sustained patterns of innate motor behaviors critical for survival (eg, feeding, locomotion, reproduction, respiration, fleeing from danger) without sensory or motor feedback.2–4 Although they are present in all organisms (from worms to humans), CPGs display species-specific features.5
Recently, we proposed that certain motor behaviors observed in parasomnias and epileptic seizures have similar features and resemble the output of particular CPGs. A temporary loss of cerebral cortical control can occur in both sleep and certain sleep-related seizures; an arousal can then permit the emergence of stereotyped, inborn, fixed-action motor patterns that are independent of the nature of the trigger (seizure or parasomnia) and reflect activation of the same CPGs.6–9
Oromotor CPG behaviors
Brainstem masticatory CPGs are essential to generate motor patterns in cranial motoneurons of the tongue, facial, and masticatory muscles involved in swallowing, chewing, respiration, self-defense, and predatory functions.10 Masticatory CPGs can produce rhythmic licking, lapping, and chewing movements, which can be refined by sensory feedbacks from the peripheral nervous system to the brainstem, showing that CPGs are adaptable and capable of responding to different environmental situations.11–13 The basic jaw movement rhythm can be modified by proprioceptive information coming mainly from muscle spindles of masticatory muscles, which accounts for the sensory feedback and feedforward information that modulates the CPG.14–16
Masticatory brainstem CPGs can be subdivided into 2 neuronal aggregates: (1) a central timing network (CTN), which provides the timing signal for rhythmic alternation of jaw opening and closing, and (2) the CPG neuronal group, which orchestrates jaw, tongue, and facial muscle activity patterns, organizes the intracycle motor pattern, and executes the movement.17,18 Each oscillation of the CTN drives the CPG to produce the motor output for 1 movement cycle. Other brainstem masticatory CPGs (such as chewing, licking, and lapping motor movements) probably share the same CTN but the CPGs producing the motor movements may differ.17–19
Sleep bruxism (SB) is a sleep disorder that is characterized by recurring episodes of rhythmic masticatory activity of the masseter and temporalis muscles accompanied by the noise of grinding or clenching teeth.20 SB usually follows an arousal, and sometimes concludes with a swallow, and can occur in any stage of sleep but most often nonrapid eye movement (NREM) stage 1 or NREM stage 2. SB is probably an extreme audible expression of another brainstem masticatory CPG, rhythmic masticatory muscle activity.21
Sleep-related faciomandibular myoclonus (FM) is another primary sleep-related movement disorder that at first glance resembles SB but is characterized on polysomnography by spontaneous myoclonic jerks of the facial, masticatory, and sometimes sternocleidomastoid muscles during NREM sleep without the tonic electromyographic masticatory activity typical of SB.22 Sleep-related FM can cause repetitive tongue biting and bleeding, and has been mistaken for sleep-related epilepsy.23 Biting often accompanying aggression has rarely been observed to occur during epileptic seizures.24,25 Repetitive fly-biting or jaw-snapping is a frequent and characteristic feature of epileptic seizures in dogs.26 Jaw-snapping, which can lead to biting, has also been observed during both epileptic and nonepileptic events in humans and dogs.
Periodic limb movements in sleep (PLMS) are more common in patients with SB. Repetitive leg movements sometimes accompany episodes of SB.21 PLMS are believed to reflect disinhibition of the locomotor CPG.27 Studies in humans have shown that PLMS are associated with increased spinal cord excitability with lower thresholds and greater spatial spread of the spinal flexor reflexes.28 Rhythmic teeth grinding and leg movements have been observed in patients with anterior and mesial temporal lobe seizures (Fig. 1A).29 Seizure-related oroalimentary automatisms in focal seizures are believed to indicate involvement of the amygdalohippocampal structures by the epileptic discharge.20,21,30,31 Mild masticatory movements also occur in prolonged absence seizures (lasting >20 seconds or longer), even triggered by merely inserting something chewable in the mouth of the patient during an absence seizure.32 Rhythmic orofacial contractions and perioral myoclonia seen in some absence seizures may also represent a release of normally inhibited brainstem masticatory CPGs.33 These data suggest that the same masticatory CPGs can be activated in etiologically different pathologic conditions (SB, PLMS) and seizure types (absence and temporal lobe complex partial seizures) (see Fig. 1).

Fig. 1 (A) Polygraphic recording of a sleep-related focal epileptic seizure in a patient with left mesial temporal lobe epilepsy, characterized by prolonged rhythmic teeth grinding (note the muscular artifacts masquerading the electroencephalographic traces in the first part of the seizure) (see also Ref.29). As the seizure evolves and ends, teeth grinding progressively fades away to be followed by rhythmic alternating leg movements (magnified in the lower insert showing electromyographic activity in the left and right tibialis anterior), which persist also in the postictal phase. (B) In a different patient, detail of a polygraphic recording showing sleep-related nonepileptic rhythmic alternating leg movements (see also Ref.91). The rhythmic alternating pattern of electromyographic contractions shown in (A) and (B) is similar, suggesting activation of the same CPG.
(Courtesy of Lino Nobili, Centre of Sleep Medicine, Niguarda Hospital, Milan.)
Oroalimentary activity during sleep may be particularly prominent in individuals with sleep-related eating disorders (SRED). SRED is defined as a parasomnia with recurrent episodes of eating after arousal from nighttime sleep that have adverse consequences. Episodes are described as compulsive, involuntary, and most often patients cannot be easily awakened during them. Epidemiologic studies suggest that nocturnal eating and SRED are particularly common in patients with restless legs syndrome (RLS) and PLMS. A recent case-control study found 33% of 100 adults with RLS self-reported SRED compared with 1% of 100 matched controls randomly selected from the general population.34
Masticatory automatisms have been described during episodes of postural hypotension in a patient with multiple system atrophy,35 and in normal individuals during reflex-induced vasovagal syncope,36 further supporting the hypothesis that the same CPG can be activated in a variety of pathophysiologically and etiologically different conditions.
Emotional CPG behaviors
The expression of emotions is a universal behavior in both animals37 and humans.38 The facial expression for each emotion results from selective and distinctive inborn motor patterns, elegantly codified in humans by Ekman and Friesen.39 The ability to accurately interpret facial expressions is of primary importance for humans to interact socially with one another. Facial expressions communicate information from which one can quickly infer the state of mind of one’s peers, and adjust one’s behavior accordingly. From this point of view, the human face can be viewed as a transmitter of expression signals and the brain as a decoder of these expression signals. It follows that facial expressions and the underlying motor circuitry (regulated by brainstem CPGs that orchestrate the facial nerves and musculature) have evolved to optimize the transmission of these signals.39,40
Humans during epileptic seizures display a coherent pattern of activation of facial muscles comparable with that observed in genuine universal facial emotions in normal individuals (in addition to several nonemotional facial displays).7,41 Coherent patterns of facial emotions are particularly observed during seizures involving the medial temporal, cingulate, and orbitofrontal cortex. Using a facial action coding system (FACS), we documented that both fear and sadness are facially expressed during epileptic seizures and use the same physiologic patterns of facial muscle activation that normal individuals use to express the same emotions (Fig. 2).7,41 In another study, we found a few frontotemporal epileptic seizures that were also associated with displays of emotions and the corresponding complex behavior, suggesting the involvement of a wide physiologic network including prefrontal, frontomesial, and temporal limbic structures.42 Ictal discharges for all emotions showed a right hemisphere predominance (except for happiness, which is associated with left-sided seizures), confirming the right hemispheric specialization for emotion.
A recent retrospective study43 reported a gender dimorphism in the ictal facial expression of emotions, which reproduces a physiologic dimorphism of human expression of emotion, reflecting either inborn or sociobehavioral differences. These investigators found no gender difference for ictal fear in children. However, more adult women reported ictal fear than adult men, and more men than women had ictal fear during childhood that disappeared during adulthood. Studies of human behavioral psychology document the prevalence of expression of rage in males and of happiness/sadness in females, relating this dimorphism to different gender social roles: competitive in males and more joining in females.
Emotional parasomnias such as sleep terrors and nightmares clearly indicate a high level of emotional arousal. In rapid eye movement sleep behavior disorder (RBD), facial expressions of emotions, mainly of negative valence, are frequently observed.44 Are facial expressions of basic emotions the same whether related to an epileptic event (limbic-hypothalamic network) or nonepileptic emotional parasomnias? Are sleep terrors and nightmares expressed by the same encoded muscle activation pattern in epilepsy as in physiologic conditions? To our knowledge, no study of facial expression during these sleep terrors or nightmares has been performed. It is relevant that decoding recognition of facial emotions is significantly impaired in epileptic patients with damage to the medial temporal lobe network occurring early in infancy.45,46
Aggressive behaviors related to epilepsy have been widely reported,47,48 and can occur in the ictal, postictal, and interictal periods.49,50 Investigation of biting behavior as a model of aggressive behavior related to epileptic seizures has been shown to occur as a reflexive behavior in the context of strong emotional arousal, fear, or anger. Biting acts were evoked (both during and after seizures) by external stimuli such as actions of people in close contact with the patient. Using stereoelectroencephalography with intracranial recording, we demonstrated that the amygdala/hippocampal region and orbitomedial prefrontal cortex were both involved when ictal biting behaviors were observed.25 These data suggest that seizure-related biting behavior could result from a loss of inhibitory control of higher centers on CPGs modulating instinctive behaviors (ie, aggressive behavior),25,51 findings that are in agreement with the views of Jasper52 on epileptic automatisms representing a paretic phenomenon (ie, the expression of loss of control).
Violent and aggressive behaviors during sleep are observed in patients with a variety of sleep disorders including NREM arousal disorders, RBD, and sleep-related epilepsies (especially nocturnal frontal lobe epilepsy [NFLE]).53 One study found a high prevalence of NREM arousal parasomnias and SB in the personal and family histories of patients with NFLE compared with normal age-matched healthy controls.54 Single-photon emission tomography studies have shown similar patterns of cingulate and cerebellar hyperperfusion during episodes of sleepwalking and epileptic paroxysmal arousals.55,56 Current evidence showing that sleepwalking, sleep terrors, and some epileptic seizures display similar ictal semiology and can be similarly provoked by sleep deprivation and arousal are in keeping with our hypothesis that both arousal disorders and NFLE share the same final common pathway (ie, activation of the same CPGs in the brainstem and spinal cord).8,9,53
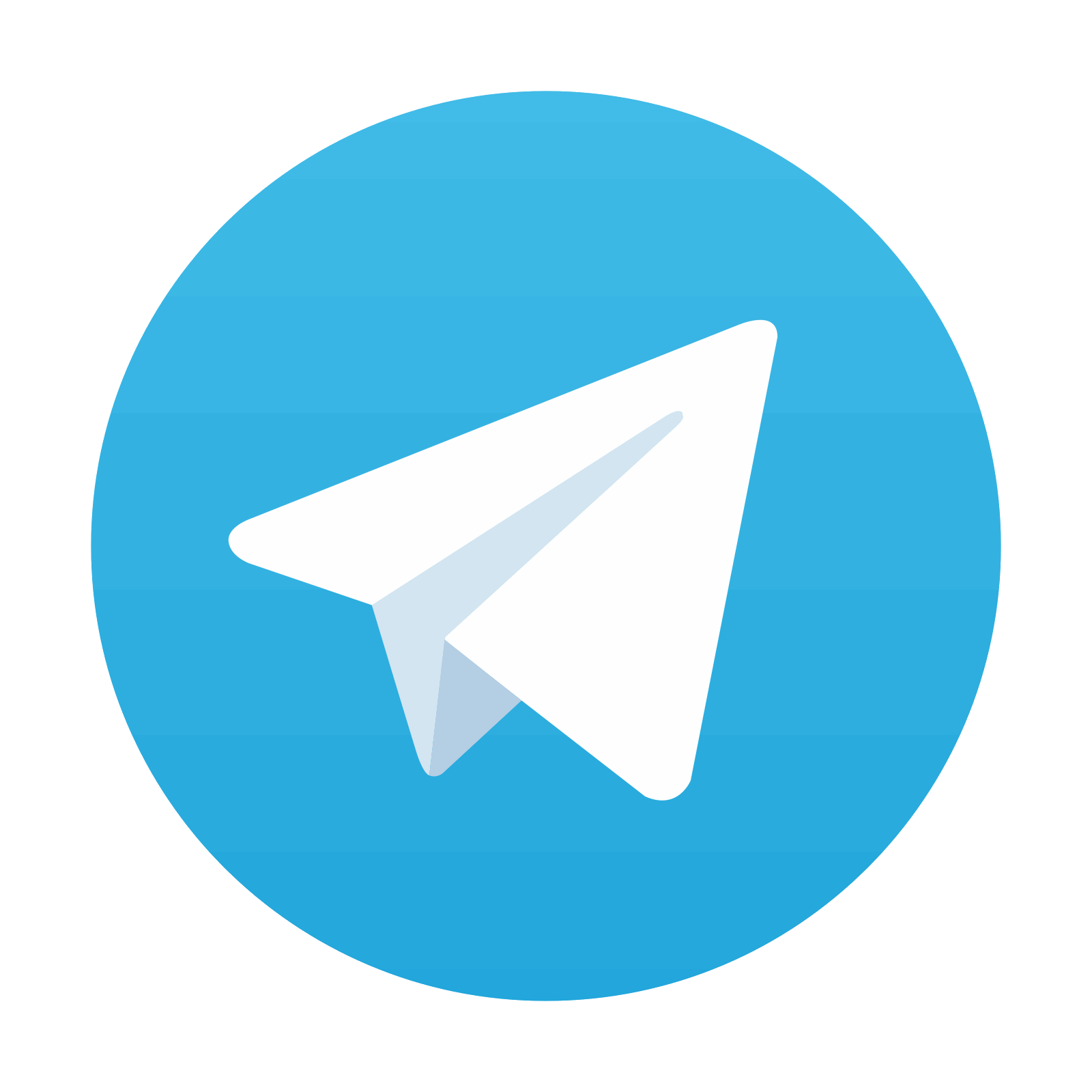
Stay updated, free articles. Join our Telegram channel

Full access? Get Clinical Tree
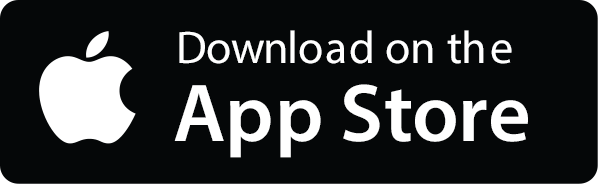
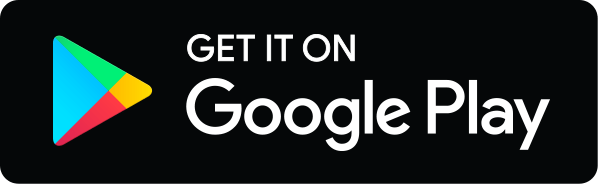