© Springer International Publishing Switzerland 2015
Jadelson Andrade, Fausto Pinto and Donna Arnett (eds.)Prevention of Cardiovascular Diseases10.1007/978-3-319-22357-5_13Genetics of Cardiovascular Disease
(1)
Department of Epidemiology, School of Public Health, University of Alabama at Birmingham, Birmingham, AL, USA
(2)
Department of Epidemiology, University of Alabama at Birmingham, Birmingham, AL, USA
(3)
University of Alabama at Birmingham, Birmingham, AL, USA
(4)
Department of Epidemiology, School of Public Health, University of Alabama School of Medicine, Birmingham, AL, USA
(5)
American Heart Association, Dallas, TX, USA
Cardiovascular diseases (CVD) remain a major source of mortality and morbidity worldwide, and considerable effort has been applied to identifying modifiable environmental factors such as diet, exercise, weight control, smoking, and drug therapy that can reduce the risk of CVD. Many CVDs have a strong familial component, however, and at least some of this is attributable to genetic factors. Over the past decades, our understanding of monogenic CVD (sometimes referred to as “simple” or “Mendelian diseases,” such as familial hypercholesterolemia and hypertrophic cardiomyopathy) has increased dramatically. Monogenetic disorders are typically caused by relatively rare variants in single genes that have a large phenotypic effect in individuals and high penetrance. Consequently, these forms of CVD account for a small proportion of the total population CVD burden. In contrast, complex genetic diseases (sometimes referred to as “common” diseases, such as atherosclerosis) may be influenced by multiple (and interacting) sequence variants, epigenetics, and gene x environment interactions. The genetic component of complex diseases is possibly the aggregate of multiple small effects. The relatively small phenotypic variation (compared to monogenic traits) of intermediate CVD traits (such as hypertension) disposes individuals toward disease development. For these reasons, genetics (the study of the function of individual genes) and genomics (the study of the function of the entire genome, i.e., genes, non-protein-coding stretches of DNA, gene–gene interactions, etc.) play a potentially important part in our evolving understanding of CVDs, including risk prediction, discovery and functional explorations of susceptibility loci, and ultimately identification of new therapeutic targets.
This chapter will review the current state of CVD genetics and genomics research, with some attention given to monogenic disease but a more detailed discussion of the flourishing arena of complex CVD genomics. Given the number of studies and the rapidly evolving research front in this discipline, we cannot hope to be comprehensive. This survey, however, should supply interested readers with the fundamental terms and methods of genetic and genomics, the areas of CV health and disease that have been most robustly explored, and the existing and potential clinical implications of this work.
Monogenic Cardiovascular Traits and Diseases
Dyslipidemias
Population-based studies and large clinical trials have demonstrated that elevated low density lipoprotein cholesterol (LDL-C) and reduced high density lipoprotein cholesterol (HDL-C) are CVD risk factors [1]. Because at least half of the variation in cholesterol and other lipids can be explained by genetic factors [2], understanding the genetic pathogenesis of hypercholesterolemia and other lipid abnormalities has long been a priority. A number of cholesterol metabolism phenotypes influenced by single genes have been documented. Studies of these monogenic conditions have helped establish the connection between hypercholesterolemia and atherosclerosis and the elucidation of these regulatory pathways have been instrumental in the development of drugs used to treat dyslipidemias [3]. As a group, these monogenic diseases are referred to as autosomal dominant hypercholesterolemia (ADH). The most common and potentially severe form of ADH is familial hypercholesterolemia (FH), which results in elevated serum LDL-C concentrations. FH results from any of some 1000 known mutations in the low-density lipoprotein receptor gene (LDLR) [4, 5]. Individuals homozygous (i.e., carry two copies of the mutation) for LDLR mutations are unresponsive to dietary and drug interventions and often develop clinical CVD before reaching age 30 years [6]. Heterozygotes (i.e., those with one copy of the mutation) have somewhat less elevated LDL-C and are more responsive to treatment interventions [7]. ADH stemming from variants in other genes have also been documented. For example, some mutations in the proprotein convertase, subtilisin/kexin-type, 9 gene (PCSK9) present symptoms similar to that of heterozygous FH [8]; interestingly, other mutations in this gene can lower LDL-C and be cardioprotective [9]. The study of the functional effects of PCSK9 mutations have lead, in part, to the development of a number of therapeutic agents that inhibit PCSK9 to reduce LDL-C [10]. Population studies estimate that 5–53 % of individuals with clinical ADH phenotypes do not have mutations in any of the known ADH genes [11]. This diagnostic gap suggests other forms of ADH remain to be discovered.
Hypertrophic Cardiomyopathy
Hypertrophic cardiomyopathy (HCM) is characterized by left ventricular hypertrophy without dilation or any obvious systemic causes (e.g., aortic stenosis, hypertension). In individuals with HCM, the normal alignment of myocytes is disrupted and electrical functions of the heart can be impeded. HCM is the most common cause of sudden death in young athletes, and it is an important contributor to heart failure and stroke [12]. The 0.2 % prevalence of HCM in the general population is considered high for a monogenic disease [13]. Some 1400 mutations in 20 genes have been identified in HCM [14], the majority of which are in sarcomere- and myofilament-related genes. About 70 % of HCM patients have mutations in either the Β-myosin heavy chain gene (MYH7) or the myosin-binding protein C gene (MYBPC3) [12]. Although HCM traditionally falls within the realm of monogenic disorders, genetic dissection of this disease has complicated the simple-complex disease dichotomy: a number of “modifier” genetic variants have been identified (e.g., END1 [15]) that add to or interact with the implicated Mendelian variants. Given that HCM can go undiagnosed until after the occurrence of a severe clinical event, it would seem an obvious candidate for genetic testing. However, given that the number of potentially pathogenic mutations (nearly all families with HCM in their pedigree have a different mutation) and that pathogenic mutations can be identified in ≤50 % of clinically affected individuals, it is likely that HCM-causing mutations would go unrecognized in a significant number of those tested. Thus, HCM illustrates the challenges of translating even detailed genetic knowledge of a monogenic disorder into fruitful clinical tools.
Other Monogenic CVDs
ADH and HCM are not the only examples of monogenic CVDs: at least some forms of Brugada syndrome, Marfan syndrome, long QT syndrome, and other conditions can also be traced to single-gene mutations. As noted above, although population-level screening may be of questionable value for some monogenic CVDs, identifying a potentially causal mutation in the proband of a family can aid in diagnosis in other family members, identification of silent carriers, reproductive risk assessment, and, in some cases, prognosis and treatment decisions.
Complex Genetic Cardiovascular Diseases
The history of the study of common genetic diseases has been driven by evolving technologies available for measuring genetic variation. Early studies used restriction fragment length polymorphism (RFLP)/electrophoresis techniques to genotype candidate genes–genes that were, a priori, hypothesized to play a role in the disease or phenotype of interest. In contrast to these hypothesis-testing experiments, genetic linkage studies are agnostic in their assumptions and are capable of scanning the entire genome for loci that may be associated with a trait of interest. These family-based studies are capable of identifying loci not previously known or suspected to be involved in the trait or disease of interest. Although each of these methods has their merits, both proved limited in their abilities. The genotyping methods of candidate gene studies could not be efficiently applied to interrogate a large selection of variants across the genome in large study samples. The micro-satellite genotyping techniques frequently used in linkage studies often provided poor localization, that is, they could identify only a broad region of the genome where the causal variant was likely to lie. The development of genotyping microarrays (“gene chips”) capable of interrogating millions of single-nucleotide polymorphisms (SNPs) throughout the genome (with the possibility of predicting millions more through statistical imputation) merged the association methods of candidate gene studies with the agnostic, hypothesis-generating methods of whole-genome linkage methods. These gene chips gave rise to the genome-wide association study (GWAS). Importantly, as we will discuss below, the design of the gene chips used in GWAS was predicated upon the common disease/common variant hypothesis: the idea that common disease-causing variants will be found in all populations with a high burden of disease. Therefore, the variants typed by GWAS arrays are relatively common in populations, usually with minor allele frequencies ≥5 %. Because the influence of any single genetic variant on disease development may be quite small, large sample populations are necessary to find statistically significant results. For this reason, many of the most compelling findings from the GWAS era come from meta-analyses of multiple studies that have investigated the same trait in different populations that sometimes total hundreds of thousands of participants, as described below.
Coronary Artery Disease
Coronary artery disease (CAD) is a complex genetic disease with heritability (i.e., proportion of inter-individual differences in a trait that is attributable to genetic differences) of about 40 % [16]. Genetic loci associated with CAD have been identified via GWAS and GWAS consortia in recent years. For example, individually, combined, and with follow-up replication using the Metabochip genotyping array (Illumina Inc), the CARDIoGRAM [17] and C4D [18] consortia have leveraged data from over 200,000 individuals of European and South Asian ancestry to identify and replicate more than 30 novel loci for CAD susceptibility [19]. About a quarter of the variants with validated CAD associations in these studies were also significantly associated with lipid traits (e.g., LDL-C and triglycerides (TG), data from the Global Lipids Genetics Consortium) and about 10 % were also significantly associated with blood pressure (data from the International Consortium of Blood Pressure), reinforcing the causal link between these risk factors and CAD risk [19]. Although findings from these studies suggest that many CAD-associated loci are important for both sexes and across racial and groups, data for blacks and other non-Caucasian groups is limited. Studies of CAD illustrate a number of critical themes that, as will become apparent, have emerged in studies of other complex diseases and phenotypes. First, genetic associations were generally stronger for hard endpoints (e.g., MI, angiographically-diagnosed CAD) rather than intermediate phenotypes like lipids [20]. Although endpoint phenotypes may not be the best choice for all complex diseases, studies of CAD underscore the importance of judicious phenotype selection and measurement in genetic studies. Second, the effect sizes of genetic variation in CAD studies were often modest (i.e., with odds ratios typically <1.2). It appears the common variants interrogated by GWAS assays explain only a small proportion of CAD heritability.
Blood Lipids, Lipoproteins, and Related Phenotypes
The monogenic lipid disorders described above account for only a small portion of the total population variation in blood lipids, and LDL-C concentrations considerably lower than those observed in some forms of ADH can still be a significant contributor to CVD, as can elevated TG and low HDL-C concentrations. Common genetic variation is believed to influence lipid trait variability. Given the explosive growth of our understanding of lipid chemistry, metabolism, and genetic underpinnings of some monogenic lipodystrophies since the middle of the last century, it’s not surprising that lipid traits have been frequently studied using candidate gene approaches. These hypothesis-driven studies were among the first to investigate the role of common variants in complex disease phenotypes. They also demonstrated the difficulty of making generalizations about genotype-phenotype associations across study populations. For example, a variant (rs670) in the promoter region of the gene (APOA1) encoding the main apolipoprotein component in HDL-C and an important actor in cholesterol excretion was associated with elevated plasma apo A-1 in Chinese male never-smokers (but not associated in male ever-smokers or females) [21]; in a European population, similar associations were found in females, but no significant associations between this variant and HDL-C were found in Canadian [22], Hispanic [23], Finnish [24], or Japanese populations [25]. Many of the common variants studied in candidate genes have been significantly associated with lipid phenotypes in GWAS, but uncertainties often remain regarding possible interacting factors and genetic heterogeneity across populations.
As of this writing, the GWAS catalog maintained by the US National Human Genome Research Institute’s Division of Genomic Medicine’s included 75 studies of more than 20 lipid-related traits with over 600 significant or suggestive genetic associations across the genome. Genes known or suspected to be involved in lipid metabolism (e.g., LDLR, genes in the apolipoprotein (APOA/APOC3) cluster on chromosome 11, and the cholesterol ester transfer protein gene (CETP)), have been reported in GWAS findings. Such confirmatory findings are important because, although the gene products may be known to play a role in lipid metabolism, identifying the variants associated with interindividual variation of a trait may provide insights into disease etiology, molecular mechanisms, and novel interventions. Many replicated GWAS findings have also pointed to novel genes not previously suspected to play a role in lipid metabolism. Lipid trait GWAS (and meta-analyses) reported that ~40–60 % of their significant associations with total cholesterol, LDL-C, HDL-C, or TG were novel [26, 27].
Hypertension
Blood pressure (BP) is a quantitative trait with 30–60 % heritability in humans [28]. As in the realm of lipid genetics, the study of the complex traits of BP and essential hypertension has often been influenced by earlier work on more severe monogenic forms of hypertension and hypotension. This earlier research identified functional mutations influencing mineralocorticoid activity, renal sodium reabsorption, and the renin-angiotensin-aldosterone system, which subsequently cause physiological imbalances presenting as abnormal BP. As with lipids, however, these rare syndromes account for only a small fraction of the population burden of hypertension, and studies of the genes associated with these Mendelian forms suggest that they contribute little to population hypertension risk [29]. Given the complexity of BP homeostasis, it is likely that genes influencing the central nervous system, endocrine system, vasculature, and blood also play a role in the phenotype. Early studies of common variants on BP-related traits were plagued by low statistical power, and heterogeneous ascertainment, comorbidities, treatment, and phenotype definitions (e.g., office BP, ambulatory BP, hypertension defined with different cutpoints, etc.) that made comparison, replication, and meta-analysis difficult. More recently, GWAS with sufficient statistical power and comparable phenotypes have found significant associations between common variants and BP-related traits. Two large GWAS consortia joined forces to form the International Consortium for Blood Pressure to perform a “mega-meta-analysis” on a discovery-phase sample of >79,000 individuals of European decent with follow up replication in >200,000 individuals from diverse ancestral populations [30]. Twenty-nine loci were associated with SBP, DBP, and hypertension. However, these statistically significant associations illustrate dramatically a conundrum that has been called the problem of “missing heritability”: the 29 BP loci discovered in the mega-meta-analysis account for less than 1 % of the trait variance, leaving unexplained the remaining 29–59 % of variation attributable to genetic factors. This is a phenomenon that has been widely reported in studies of common genetic variation (i.e., GWAS) of most presumed polygenic traits.
< div class='tao-gold-member'>
Only gold members can continue reading. Log In or Register a > to continue
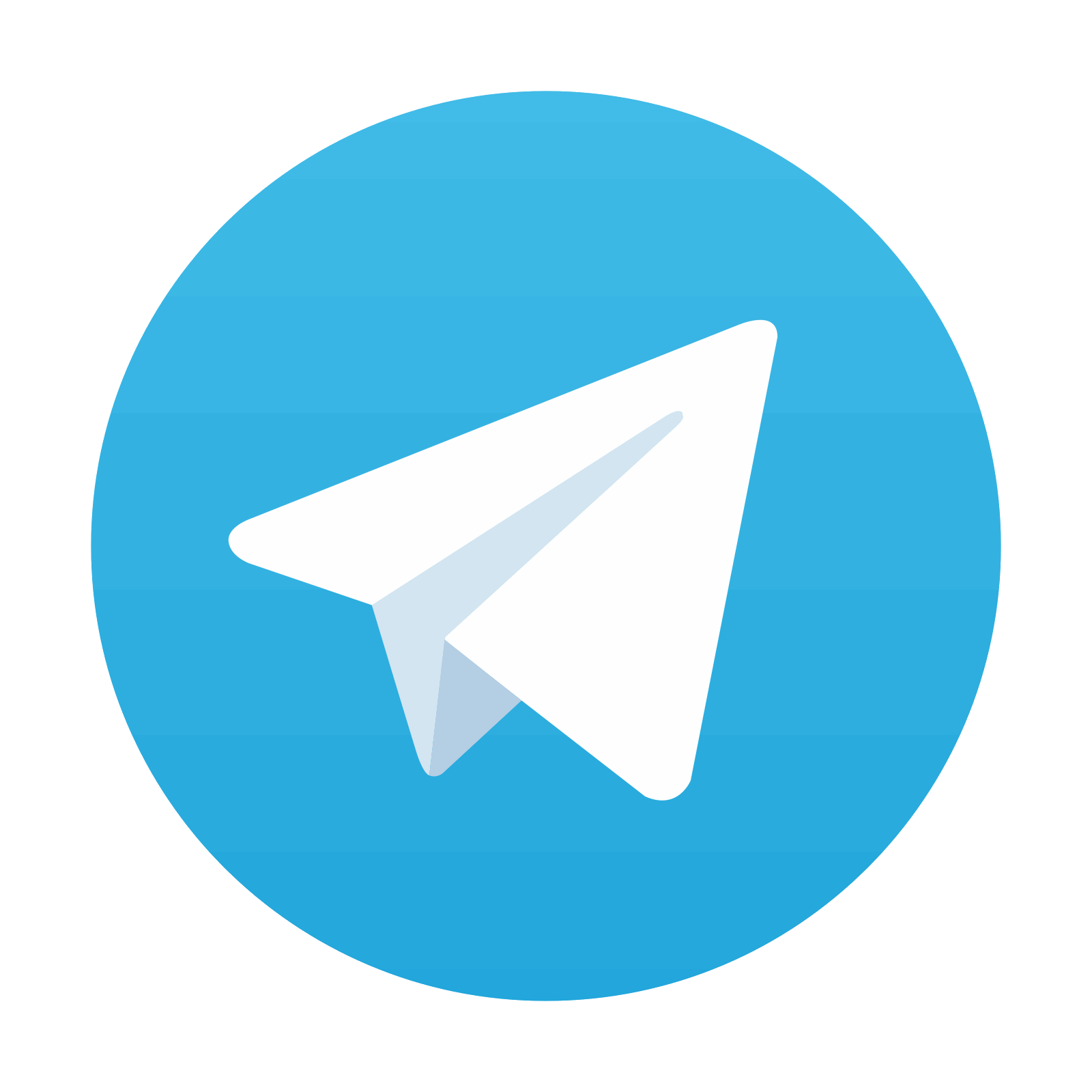
Stay updated, free articles. Join our Telegram channel

Full access? Get Clinical Tree
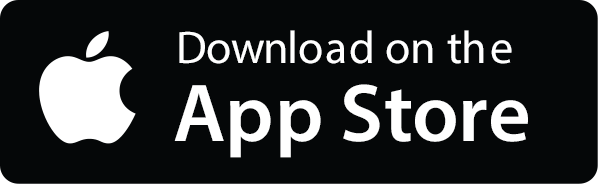
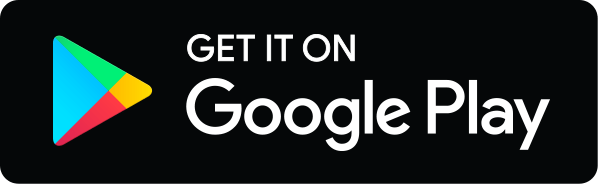