Obesity, Adipokines, Inflammation, and Cardiovascular Disease
Joseph J. Lamb, MD
Introduction
As noted by Hotamisligil,1 the interactions between immune and metabolic pathways are highly ordered, evolutionary conserved, and are important for tissue and organism health. When this balance is disrupted by maladaptive gene-environment interactions, the result is the development of chronic noncommunicable diseases associated with inflammation and aging including obesity, diabetes, and cardiovascular disease. Complex signaling pathways play an integral role in mediating the interactions between systems designed to provide the best response to physiologic stresses due to environmental change, to provide the balance of nutrient intake both at a micronutrient and macronutrient and informational level, and finally to initiate the complex orchestration of inflammatory responses that lead to the most adaptive response to these environmental challenges.
A significant body of research demonstrates that nutrients and metabolites modulate the inflammatory pathways, the stress pathways, and the energy regulation pathways, resulting in either desired optimal health or the progression to the chronic noncommunicable diseases of aging. An excellent review by Saltiel and Olefsky2 supports the hypothesis that inflammation is an early response activated during adipose expansion and during the development of chronic obesity. These changes lead to an activation of the immune system and a shift to a pro-inflammatory phenotype. This pro-inflammatory phenotype is not limited to the activation of the innate adaptive immune systems but instead is hallmarked by inflammatory changes within many organ systems including the pancreas, the brain, liver, gut, and muscle. Excessive nutrient intake associated with an overall positive energy balance leads to the accumulation of excessive fat and adipocytes both in the subcutaneous and visceral spaces. The initiation of an inflammatory response is a beneficial physiologic response to the stresses that obesity places on adipocytes. However, long-term activation is maladaptive in the long term.3 As noted by Scherer et al.,4 adipocytes to be successful in their role as the major energy storing tissue in the body require an almost unlimited capacity to expand. Thus, adipocytes require the ability to remodel acutely and chronically. This remodeling can be challenged because of the overexpansion of adipocytes and the limit of oxygen due to the failure to develop adequate vascular supply. In this setting, hypoxia becomes an early determinate of the health of the expanding adipose tissue.
Inflammation is characterized by the deterioration of metabolic health that is clearly detrimental to longevity. Caloric excess disrupts healthy metabolic pathways and accelerates aging, while conversely calorie restriction has been associated with restoration of normal function, prevention of chronic diseases, and maintenance of longevity. Using a genetic mouse model lacking FABP4/5 that confers protection against metabolic diseases, Charles et al.5 demonstrated that FABP4-deficient mice exhibited preserved insulin sensitivity resulting in reduced inflammation, maintenance of adipose tissue integrity, and prevention of fatty liver disease. These mice, however, did not gain an extension of life span. The authors conclude that a complex interplay of environmental signals, divergent metabolic pathways, and functional and dysfunctional physiologic responses dictate the overall response of the organism to its environment.
Obesity and the Link to Inflammation and Cardiovascular Disease
A comparison of the anatomical changes during the evolution from a common fruit fly to the anatomy of a mammal over 600 million years demonstrates the development of unique organ systems replacing what is in the fruit fly, a nonspecialized fat body. This fat body is a vascularly supplied
collection of the adipocytes, metabolically active cells, and immune cells that would eventually specialize into specialized adipose stores, the metabolically active organs (liver and pancreas), and the innate and adaptive immune systems.6 A consequence of this shared evolutionary origin for these cell types is the conservation of paracrine (cell to cell transmission) signals (adipokines, cytokines, hormones) into autocrine hormonal signaling and inflammatory signaling (organ to organ signaling at a distance dependent on the neuroendocrine systems). These shared signaling pathways reflect an evolutionary integration of regulatory pathways governing the body’s responses to pathogens and nutrition. Zhang et al.7 postulate that the hypothalamus developed the unique ability to respond to the environment through inflammatory signaling. This pro-inflammatory response which for our ancestors was adaptive and typically activated by pathogenic exposures is now quite detrimental in the setting of repeated responses to nutrient excess provided by our diet of convenience and conspicuous consumption. Toll-like receptors (TLRs) share an important function in this signaling. TLR4-deficient mice were noted to have aggravated fasting hypoglycemia. Overstimulation of TLR4 has been associated with the development of insulin resistance. Pang8 suggests that TLR4 is thus critical for the maintenance of glucose and lipid metabolism independent of insulin signaling. Although TLR4 is classically recognized as the receptor for lipopolysaccharides, Shi et al.9 have demonstrated that the obesity-associated increase in nutritional fatty acid levels activate TLR4 signaling and adipocytes and macrophages, resulting in the induction of inflammatory signaling. They demonstrate that mice lacking TLR4 do not develop impaired insulin signaling in muscle or the development of insulin resistance with the infusion of systemic lipids.
collection of the adipocytes, metabolically active cells, and immune cells that would eventually specialize into specialized adipose stores, the metabolically active organs (liver and pancreas), and the innate and adaptive immune systems.6 A consequence of this shared evolutionary origin for these cell types is the conservation of paracrine (cell to cell transmission) signals (adipokines, cytokines, hormones) into autocrine hormonal signaling and inflammatory signaling (organ to organ signaling at a distance dependent on the neuroendocrine systems). These shared signaling pathways reflect an evolutionary integration of regulatory pathways governing the body’s responses to pathogens and nutrition. Zhang et al.7 postulate that the hypothalamus developed the unique ability to respond to the environment through inflammatory signaling. This pro-inflammatory response which for our ancestors was adaptive and typically activated by pathogenic exposures is now quite detrimental in the setting of repeated responses to nutrient excess provided by our diet of convenience and conspicuous consumption. Toll-like receptors (TLRs) share an important function in this signaling. TLR4-deficient mice were noted to have aggravated fasting hypoglycemia. Overstimulation of TLR4 has been associated with the development of insulin resistance. Pang8 suggests that TLR4 is thus critical for the maintenance of glucose and lipid metabolism independent of insulin signaling. Although TLR4 is classically recognized as the receptor for lipopolysaccharides, Shi et al.9 have demonstrated that the obesity-associated increase in nutritional fatty acid levels activate TLR4 signaling and adipocytes and macrophages, resulting in the induction of inflammatory signaling. They demonstrate that mice lacking TLR4 do not develop impaired insulin signaling in muscle or the development of insulin resistance with the infusion of systemic lipids.
Howitz and Sinclair10 note that the pathways linking inflammation, energy utilization, and stress responses are driven by a collection of signaling molecules and kinases, with AMP kinase playing an important role in the center of the pathway, pro-inflammatory signaling influencing MTOR production, and PGC1α being the link between energy utilization and stress responses. Although the signals and mediators responsible for our autonomic responsiveness (the fight-or-flight response and the Tend-and-Befriend response), and the counterregulatory hormones necessary to maintain homeostasis of glucose and other energy-producing molecules are well understood, the regulation of energy utilization and storage in adipocytes is less well defined. It is clear, however, that adipose tissue, specifically the metabolically active visceral adipose tissue, is a central mediator of paracrine and autocrine signaling.
Prentice et al.11 note that fatty acid binding protein 4 (FABP4) functions in maintaining glucose homeostasis and is a key regulatory node facilitating communication in life’s threatening situations between energy storage systems and distant organs. However, chronic engagement of FABP4 in settings such as obesity or nutrient excess aggravates the chronic noncommunicable diseases of aging including diabetes, asthma, cancer, and atherosclerosis.
The benefits of fasting and calorie restriction have been well documented in yeast, round worms, and several mammalian models. The longevity associated with calorie restriction is mediated through sirtuin regulation.12 It has been demonstrated that calorie restriction and resulting physiologic changes are associated with activation of cellular stress response elements, improved autophagy, modification of apoptosis, and alteration in hormonal signaling.12 Sirtuin enzymes, specifically the seven mammalian ones (SIRT1-7), are integral to self-survival, in essence, as key regulators of metabolism and genomic stability.13 SIRT 1 and SIRT 6 signaling has been implicated in the balance between insulin sensitivity and resistance, resulting in the development of type 2 diabetes, fatty liver disease, and cardiovascular disease. Sirtuins regulate the production of energy matching output from resources to demands. SIRT 1 enhances fat metabolism and modulates mitochondrial respiration to optimize energy harvesting. The AMP kinase/SIRT1/PGC1α-PPAR axis and mitochondrial sirtuins are pivotal to maintaining healthy mitochondrial function. Downregulation of sirtuins with aging may explain much of the pathophysiology that is generally associated with aging.14 The nuclear sirtuins (SIRT 1, 2, 6, and 7) are all intimately associated with the regulation of inflammatory signaling pathways and play a role to reduce pro-inflammatory signaling.15
Adipose tissue with its complex interplay of hormonal and adipocytokine signaling plays a significant role in hormonal regulation of metabolic homeostasis. This regulatory function of the adipose tissue depends on its ability to secrete a large number of hormones, cytokines, extracellular matrix proteins, and growth factors influencing local and systemic metabolism. Barchetta et al.16 note that in conditions of nutrient excess leading to chronic positive energy balance, the expansion of adipose tissue with resultant hypoxia, apoptosis, and inflammatory changes leads to dysfunction and subsequent delivery of paracrine and autocrine alarm messages, resulting in metabolic derangements. Thus, this nutrient excess, with its resultant mismatch between environmental supply of energy and the requirements of cellular homeostasis, dysregulates appropriate physiologic function of adipose tissue with the subsequent development of signaling incongruent with optimal health. This dysfunction creates a milieu of angry sick fat exacerbated by the presence of lipopolysaccharide in the visceral adipose tissue that is bathed in enterohepatic circulation of the portal system. Visceral adipose tissue is thought to be more metabolically sensitive and active than subcutaneous adipose tissue.
The distinction between visceral and subcutaneous adipose tissue is not the only macroscopically observed difference between adipose tissues. White adipose tissue (WAT) can be differentiated from brown adipose tissue (BAT) on a macroscopic and microscopic level because of the presence of an enriched mitochondrial population in BAT. WAT’s primary function is to accumulate surplus energy (predominately in the form of triglycerides), whereas BAT dissipates energy directly as heat and plays critical functions in the maintenance of temperature homeostasis.
Subcutaneous and visceral adipocytes have different progenitor cells with resultant differences in gene expression patterns. Thus, subcutaneous WAT is more responsive to the antilipolytic effects of insulin. It actually secretes more adiponectin and fewer inflammatory cytokines.17 BAT plays a critical role in the ability of hibernating mammals to sustain their physiologies throughout the winter months and to maintain their temperature regulation. BAT’s thermoregulatory properties provide a resource for infants with their high body surface area to mass ratios requiring energy support. Medina Gomez18 has suggested that strategies targeting adipocyte mitochondrial function in WAT and BAT may play a critical role in the development of therapies fighting obesity and its complications in the near future.19 Another critical function of sirtuins is the ability of SIRT 5 to influence brown adipocyte differentiation with the results of browning of WAT resulting in what may be regarded as beige adipose tissue as the WAT gains some of the responsiveness of BAT to appropriate environmental signaling.
This browning of adipose tissue requires an adaptive increase in proteasomal activity resulting in responsiveness to nutrient excess and xenohormetic messages such as fasting and cold exposures. Bartelt et al.20 demonstrated that cold adaptation induces Nrf1 in BAT and is thus crucial for maintaining endoplasmic reticulum (ER) homeostasis and integrity. In a mouse model, deletion of Nrf1 in thermogenic conditions resulted in ER stress, tissue inflammation, decreased mitochondrial functioning, and whitening of BAT.
Obesity and diabetes are certainly the consequences of disordered regulatory signaling in metabolically sensitive tissue. These metabolically sensitive tissues are certainly not limited to white and brown adipose tissues, including hepatocytes, pancreatic beta cells, and myocytes, and also including our immune signaling and our neuro tissues as our previous discussion has suggested. Corkey et al.21 suggest that our redux metabolome coordinates tissue responses by virtue of a circulating communication system. Branched chain amino acids, lactate, and free fatty acids all generate increases in mitochondrial NADH through their metabolism, influencing the oxidative reductive balance. A possible circulating master metabolic regulator, recognizing the balance between lactate and pyruvate, between beta-hydroxybutyrate and acetoacetate, between reduced and oxidized thiocytosine and the presence of reductive reactive oxygen species, coordinates signaling between the gut and the brain in terms of hunger and satiety, the balance in the liver between glycogen storage and glucose release, the balance in the adipocyte between lipolysis and lipogenesis, and the balance between insulin and glucagon secretion in pancreatic beta cells.
Interestingly, the balance between these oxidative and reductive compounds is maintained through mitochondrial function. Wallace22 in 2010 notes that “mitochondrial mutations provide heritable and stable adaptation to regional differences while mitochondrially-mediated changes in the epigenome (Nuclear DNA) permit reversible modulation of gene expression in response to fluctuations in the energy environment.” Thus, the balance in oxidative reductive reserves not only dictate the immediate short-term responsiveness but also influence the long-term responsiveness of the system allowing for transgenerational and regional adaptations to changing environmental stimuli.
Lee et al.23 report that an early change during the consumption of a high-fat diet is the uncoupling of adipocyte respiration, leading to increased oxygen consumption in a state of relative adipocyte hypoxia. Adipose hypoxia defined as a relative oxygen deficiency is a potential contributor to adipose tissue dysfunction. Each 5 pounds of adipose tissue has a vascular supply of approximately one additional mile of capillaries, arterials, and venules, resulting in a vascular bed with increased systemic resistance and consequences for a systemic hypertension and vascular dysfunction. Lempesis24 suggests that alternations in adipose tissue oxygenation directly impact metabolic homeostasis resulting in adipocyte hypertrophy, impaired adipokine secretion, chronic inflammatory changes, and the development of insulin resistance.
Clearly in our Westernized world, there has been a significant change in the diseases that contribute most dramatically to morbidity and mortality. The burden of infectious diseases has responded to improved hygiene and the development of effective antibiotics. However, for those living in Westernized countries who could be considered to be participating the largest dietary study ever initiated, specifically the industrialization of the diet, resulting in excessive consumption of fat, cholesterol, proteins, carbohydrates, specifically refined sugar and salts as a consequence of the frequent consumption of processed and fast foods and decreased consumption of phytonutrients and fiber, the dietary pattern has created an environment where metabolic, cardiovascular, and autoimmune diseases are almost epidemic.
T cell regulation links the dietary influences of a Western diet to the development of autoimmune pathology and the possible promotion of the chronic noncommunicable diseases of aging.25 It is well recognized that inflammation is linked with the development of nonalcoholic fatty liver disease, diabetes, and endothelial dysfunction, resulting in coronary artery disease and immune dysregulation. Similar patterns of dysfunction are noted beyond the cardiovascular system. An additional consequence of these inflammatory changes is the increased permeability of the blood-brain barrier. Chronic systemic inflammation induced by obesity and type 2 diabetes promotes the blood-brain barrier breakdown with decreased removal of wastes and increased infiltration of immune cells. These changes result in the development of a pro-inflammatory pattern which has been characterized as a brain on fire with a resultant development of neuroinflammation and its connection to Alzheimer’s disease and multiple sclerosis.26 Similarly, Wang et al.27 have documented the cross talk between osteoarthritis and obesity resulting in increases in pro-inflammatory adipokines and cytokines driving the localized and systemic pro-inflammatory condition.
For quite some time, the cholesterol hypothesis has been predominant in our understanding of the pathophysiology of coronary artery disease, and certainly the continued profound primacy of the HMG-CoA reductase inhibitors (statins) as
one of the most frequently prescribed pharmaceutical agents would suggest that the association of inflammation with both coronary artery disease, obesity, and the chronic noncommunicable diseases of aging has not yet been recognized. Statins obviously possess anti-inflammatory properties, but they are recognized by the public and many prescribers as primarily cholesterol-lowering agents. Ridker et al.28 demonstrated in 2007 that LDL reduction not associated with the reduction in inflammation as marked by a reduction in HSCRP would not be associated with a reduction in cardiovascular risk. They demonstrated in the Jupiter trial that apparently healthy persons without hyperlipidemia but with HSCRP protein levels noted a significant reduction in the incidence of major cardiovascular event with rosuvastatin treatment. Winer and Winer29 have noted that chronic inflammation of visceral adipose tissue has gained acceptance as one of our lead promoters of insulin resistance in obesity. The infiltration of the adipose tissue by both B and T lymphocytes, the resultant secretion of cytokines, and macrophage modulation dictate the development of a local inflammatory response with the resultant development of insulin resistance and a systemic inflammatory pattern.29 The unique role of the visceral adipose tissue is demonstrated by Klein et al.30 who reported that liposuction did not significantly alter the insulin sensitivity of myocytes, hepatocytes, or adipose tissue despite the removal of 20 pounds of subcutaneous fat. Plasma concentrations of HSCRP, IL-6, tumor necrosis factor-alpha (TNF-α), and adiponectin were not altered and there was no significant impact upon other risk factors for coronary artery disease. Engin31 notes that novel and highly active molecules are released abundantly by adipocytes including leptin, resistin, adiponectin, and visfatin as well as some of the more classical cytokines. Cytokines that are released by inflammatory cells infiltrating obese adipose tissue include TNF-α, interleukin-6 (IL-6), monocyte chemoattractant protein 1 (MCP-1), and IL-1. These molecules, acting on immune cells, lead to local and generalized inflammation. Stimulation of the TLR-4 modulation of the phosphatidylinositol-3 kinase (PI3K), of protein kinase B (Akt) signaling, of the uncoupling of the unfolded protein response due to ER stress, and finally of activation of the c-Jun N-terminal Kinase (JNK) Activator Protein 1, an inhibitor of nuclear factor kappa-B pathways, plays an important role resulting in vascular endothelial dysfunction by modulating vascular nitric oxide and super oxide release. The development of systemic oxidative stress results in macrophage recruitment, increases in the expression of NOD-like receptor family proteins (NLRP3) in the inflammasome, and adipocyte death, which are predominant determinants in the pathogenesis of obesity-associated adipose tissue inflammation. The infiltrating macrophages produce not only cytokines but also metalloproteinases, reactive oxygen species, and chemokines. These participate in tissue remodeling, cell signaling, and the regulation of immunity with the resultant influence on adjacent tissues and organs. Guzik et al.32 note that “in blood vessels, perivascular adipose tissue and inflammation leads to vascular remodeling, super oxide production, and endothelial dysfunction with loss of nitric oxide bioavailability contributing to the development of vascular disease, atherosclerosis and plaque instability.” Erridge et al.33 noted that the postprandial endotoxemia associated with the consumption of a Westernized diet is a potential contributor to endothelial activation and the development of atherosclerosis. Pendyala et al.34 investigated the connection between a Western style diet and endotoxemia. The Western style diet, inclusive of approximately 100 g of fat intake per day, produced a 71% increase in plasma endotoxin levels, while a prudent style diet reduced the levels by 31%. Cani et al.35 demonstrated that metabolic endotoxemia initiates obesity and insulin resistance. In a fascinating study, mice were fed a high-fat diet compared with the standard chow diet with the subsequent development of obesity. Endotoxin levels were measured in the mice receiving the high-fat diet compared with a standard chow diet. A second experimental group received an infusion of endotoxin while consuming a standard chow diet. The group receiving endotoxin and the lower caloric diet had metabolic changes resulting in the development of obesity and a pattern similar to the mice fed the high-fat diet. Mehta et al.36 demonstrated that an infusion of endotoxin in humans produced insulin resistance and activation of the innate adaptive immune systems. Interestingly, the development of acute inflammation and adipose tissue changes were noted in the subcutaneous adipose tissue and not restricted to the visceral adipose tissue. The consequences of excessive nutrient intake (both dietary fats and refined carbohydrates), the increase in lipopolysaccharide absorption and increased environmental toxicities, result in a pattern of physiologic changes of which early signs are fatty acid infiltration in the liver, beta cell apoptosis and dysfunction, and the expansion of visceral adiposity. The early physiologic changes contemporaneous with the development of visceral tissue expansion suggest that obesity is not an etiologic determinant of type 2 diabetes, atherosclerosis, Alzheimer’s/dementia and the other chronic noncommunicable diseases of aging but is instead an early sign and symptom of the disordered pathophysiologies associated with our patterns of Western living.
one of the most frequently prescribed pharmaceutical agents would suggest that the association of inflammation with both coronary artery disease, obesity, and the chronic noncommunicable diseases of aging has not yet been recognized. Statins obviously possess anti-inflammatory properties, but they are recognized by the public and many prescribers as primarily cholesterol-lowering agents. Ridker et al.28 demonstrated in 2007 that LDL reduction not associated with the reduction in inflammation as marked by a reduction in HSCRP would not be associated with a reduction in cardiovascular risk. They demonstrated in the Jupiter trial that apparently healthy persons without hyperlipidemia but with HSCRP protein levels noted a significant reduction in the incidence of major cardiovascular event with rosuvastatin treatment. Winer and Winer29 have noted that chronic inflammation of visceral adipose tissue has gained acceptance as one of our lead promoters of insulin resistance in obesity. The infiltration of the adipose tissue by both B and T lymphocytes, the resultant secretion of cytokines, and macrophage modulation dictate the development of a local inflammatory response with the resultant development of insulin resistance and a systemic inflammatory pattern.29 The unique role of the visceral adipose tissue is demonstrated by Klein et al.30 who reported that liposuction did not significantly alter the insulin sensitivity of myocytes, hepatocytes, or adipose tissue despite the removal of 20 pounds of subcutaneous fat. Plasma concentrations of HSCRP, IL-6, tumor necrosis factor-alpha (TNF-α), and adiponectin were not altered and there was no significant impact upon other risk factors for coronary artery disease. Engin31 notes that novel and highly active molecules are released abundantly by adipocytes including leptin, resistin, adiponectin, and visfatin as well as some of the more classical cytokines. Cytokines that are released by inflammatory cells infiltrating obese adipose tissue include TNF-α, interleukin-6 (IL-6), monocyte chemoattractant protein 1 (MCP-1), and IL-1. These molecules, acting on immune cells, lead to local and generalized inflammation. Stimulation of the TLR-4 modulation of the phosphatidylinositol-3 kinase (PI3K), of protein kinase B (Akt) signaling, of the uncoupling of the unfolded protein response due to ER stress, and finally of activation of the c-Jun N-terminal Kinase (JNK) Activator Protein 1, an inhibitor of nuclear factor kappa-B pathways, plays an important role resulting in vascular endothelial dysfunction by modulating vascular nitric oxide and super oxide release. The development of systemic oxidative stress results in macrophage recruitment, increases in the expression of NOD-like receptor family proteins (NLRP3) in the inflammasome, and adipocyte death, which are predominant determinants in the pathogenesis of obesity-associated adipose tissue inflammation. The infiltrating macrophages produce not only cytokines but also metalloproteinases, reactive oxygen species, and chemokines. These participate in tissue remodeling, cell signaling, and the regulation of immunity with the resultant influence on adjacent tissues and organs. Guzik et al.32 note that “in blood vessels, perivascular adipose tissue and inflammation leads to vascular remodeling, super oxide production, and endothelial dysfunction with loss of nitric oxide bioavailability contributing to the development of vascular disease, atherosclerosis and plaque instability.” Erridge et al.33 noted that the postprandial endotoxemia associated with the consumption of a Westernized diet is a potential contributor to endothelial activation and the development of atherosclerosis. Pendyala et al.34 investigated the connection between a Western style diet and endotoxemia. The Western style diet, inclusive of approximately 100 g of fat intake per day, produced a 71% increase in plasma endotoxin levels, while a prudent style diet reduced the levels by 31%. Cani et al.35 demonstrated that metabolic endotoxemia initiates obesity and insulin resistance. In a fascinating study, mice were fed a high-fat diet compared with the standard chow diet with the subsequent development of obesity. Endotoxin levels were measured in the mice receiving the high-fat diet compared with a standard chow diet. A second experimental group received an infusion of endotoxin while consuming a standard chow diet. The group receiving endotoxin and the lower caloric diet had metabolic changes resulting in the development of obesity and a pattern similar to the mice fed the high-fat diet. Mehta et al.36 demonstrated that an infusion of endotoxin in humans produced insulin resistance and activation of the innate adaptive immune systems. Interestingly, the development of acute inflammation and adipose tissue changes were noted in the subcutaneous adipose tissue and not restricted to the visceral adipose tissue. The consequences of excessive nutrient intake (both dietary fats and refined carbohydrates), the increase in lipopolysaccharide absorption and increased environmental toxicities, result in a pattern of physiologic changes of which early signs are fatty acid infiltration in the liver, beta cell apoptosis and dysfunction, and the expansion of visceral adiposity. The early physiologic changes contemporaneous with the development of visceral tissue expansion suggest that obesity is not an etiologic determinant of type 2 diabetes, atherosclerosis, Alzheimer’s/dementia and the other chronic noncommunicable diseases of aging but is instead an early sign and symptom of the disordered pathophysiologies associated with our patterns of Western living.
Hormonal Signaling in Obesity: Regulation of Feeding and Satiety
A broad range of neurotransmitters and hormones influence feeding and satiety in the hypothalamus, resulting in the control of nutrient balance. A partial list of those hormones that decrease feeding (anorexigenic) include leptin, serotonin, norepinephrine (NE), cortisol-releasing hormone (CRH), insulin, CCK, peptide YY. A similar list of hormones that increase feeding (orexigenic) include neuropeptide Y (NPY), orexins A and B, endorphins, amino acids (glutamate and γ-aminobutyric acid), cortisol, ghrelin, endocannabinoids, agouti-related protein (AGRP).37 These hormones act upon two distinct types of neurons in the hypothalamus. The pro-opiomelanocortin neurons (POMC) upon anorexigenic activation decrease food intake and increase energy expenditure.
NPY/AGRP neurons upon orexigenic activation increase food intake and decrease energy expenditures. POMC neurons secrete α-melanocyte-stimulating hormones (MSH), and defective signaling is associated with the extreme obesity. Mutations of the melanocortin receptors specifically MCR-4 is possibly the most commonly known monogenic cause of human obesity accounting for 5% to 6% of early onset of severe obesity in children.38,39 Oral receptors including taste receptors mediate food intake as demonstrated by experiments where patent esophageal fistulas leading to the loss of ingested food decrease feeding behaviors.40 Orexigenic hormone signaling, including ghrelin, increased feeding. Blood ghrelin levels increase during fasting, peak just before eating, and fall rapidly after a meal. Ghrelin is secreted by the stomach. Decreases in blood glucose concentration causes hunger and increases feeding behavior. Similar changes in amino acids and free fatty acids result in the development of hunger as well. Interestingly, the stimulation of POMC neurons by synergistic insulin and leptin signaling leads to the browning of WAT. Stimulation of AGRP neurons by ghrelin, cortisol, and endocannabinoids suppresses WAT browning.41
NPY/AGRP neurons upon orexigenic activation increase food intake and decrease energy expenditures. POMC neurons secrete α-melanocyte-stimulating hormones (MSH), and defective signaling is associated with the extreme obesity. Mutations of the melanocortin receptors specifically MCR-4 is possibly the most commonly known monogenic cause of human obesity accounting for 5% to 6% of early onset of severe obesity in children.38,39 Oral receptors including taste receptors mediate food intake as demonstrated by experiments where patent esophageal fistulas leading to the loss of ingested food decrease feeding behaviors.40 Orexigenic hormone signaling, including ghrelin, increased feeding. Blood ghrelin levels increase during fasting, peak just before eating, and fall rapidly after a meal. Ghrelin is secreted by the stomach. Decreases in blood glucose concentration causes hunger and increases feeding behavior. Similar changes in amino acids and free fatty acids result in the development of hunger as well. Interestingly, the stimulation of POMC neurons by synergistic insulin and leptin signaling leads to the browning of WAT. Stimulation of AGRP neurons by ghrelin, cortisol, and endocannabinoids suppresses WAT browning.41
Hormonal Signaling in Obesity: Insulin Signaling
It is generally accepted that the interaction of environmental signals, genetic influences, and food consumption leads to the development of increased visceral adiposity and the reduction in adiponectin levels. These changes result in the development of insulin resistance manifested by hyperlipidemia, diabetes mellitus, hypertension, and the subsequent development of atherosclerosis.42 Dr Barbara Corkey was awarded the Banting Award for excellence in diabetic research in 2011; in her Banting Lecture, she proposed that an early etiology of insulin resistance is the hyperinsulinemia, which is a consequence of the increased beta cell secretion of insulin. She identified that mono-oleoylglycerols, iron, and saccharin are all common dietary ingredients that are capable of producing this hyperinsulinemia.43 The hypersecretion of insulin due to mitochondrial toxicity of the beta cells leads to increased circulating insulin levels and may be associated with the downregulation of insulin receptors with the consequence of the development of insulin resistance separate from the role played by glucose and lipid toxicity. It has been noted by Schauer et al.44 that obese patients with diabetes respond differently to gastric bypass surgery versus intensive medical therapy. They noted that the use of antihypertensive, antidiabetic, and lipid modulating drugs decrease significantly after surgical treatment but increased in patients receiving medical therapies only. The homeostatic model of assessment for insulin resistance score improved significantly only in the bariatric surgery group. It is interesting to note that insulin-requiring diabetics undergoing bariatric surgery have an almost immediate reduction in the amount of insulin required during the postoperative period. Individuals who are NPO for other major abdominal surgeries, including cholecystectomies and colectomies, do not have a reduction in their requirements for insulin. This reduction in insulin dosing takes place well before associated weight loss. This effect is seen in individuals undergoing the Roux-en-y procedure and is not seen in patients with gastric reduction or gastric sleeves. The Roux-en-y procedure results in anatomical changes that result in the food stream bypassing the duodenum and the proximal jejunum. G-protein-coupled receptor signaling is modified by the changes in the food stream present after the Roux-en-y procedure. G-coupled protein receptors and kinase signaling are closely linked with the regulation of energy utilization as well as inflammatory signaling. Stimulation of the G-coupled protein receptor 120 leads to secretion of glucagon-like peptide-1 (GLP-1). Food has a dramatic influence on GLP-1 secretion. GLP-1 increases insulin sensitivity and reduces inflammation. Lockie et al.45 note that activation of central nervous system GLP-1 receptors and additional g-coupled protein receptors actually stimulate brown adipose activity through the sympathetic nervous system. Stimulation of g-coupled protein receptor by omega-3 fatty acids (DHA) lead to GLP-1 secretion with improved glucose stimulated insulin secretion and reductions in inflammation and insulin resistance.46
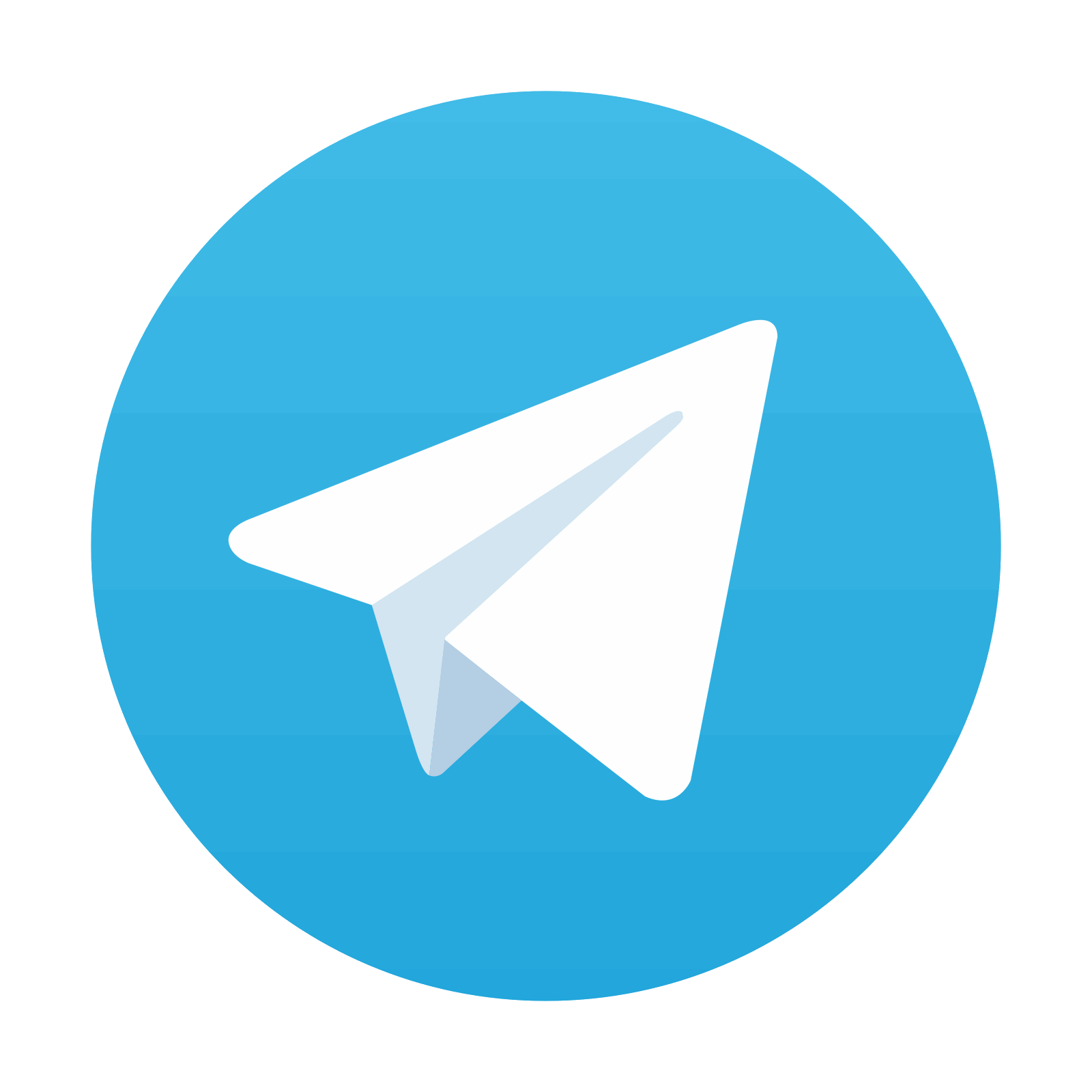
Stay updated, free articles. Join our Telegram channel

Full access? Get Clinical Tree
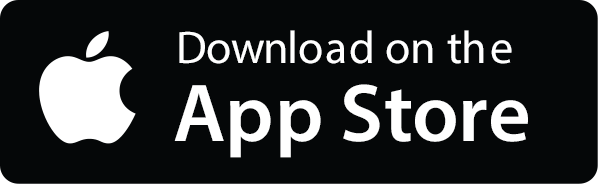
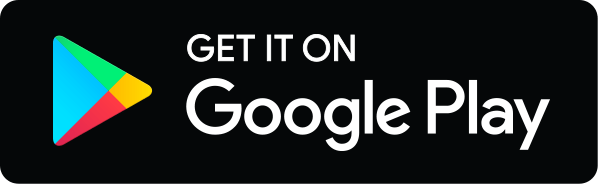