CME
The Nuts and Bolts of Electroencephalography
Keywords
• Electroencephalography • Polysomnography • Epilepsy • Technical polysomnography • Technical electroencephalography
Electroencephalography (EEG) and polysomnography (PSG) have evolved together since their infancy. Fig. 1 shows single-channel sleep EEG samples performed by Charles Henry in 1939, in an era when EEG and PSG were identical enterprises and every recording was an adventure. Since then, the number of channels, convenience, and flexibility of recording have grown enormously but the fundamentals of detecting and interpreting electrical signals from the human body have changed far less. This article reviews some of the basics of EEG technology and discusses how an understanding of them can help inform both recording and interpretation.
Origin of the scalp EEG
For EEG signals to be detected through the skull and other intervening tissues, synchronous activity must extend over cortical areas of 10 cm2 or more, which does not mean that all of the neurons in that area are behaving synchronously; perhaps only a small fraction of them are. Ten cm2 is the cortical expanse possessed by a medium-sized rodent.1 Because a rodent brain is capable of doing many different things simultaneously, this consideration may place significant limitations on the level of detail in the information that can be extracted from scalp EEG.
Fig. 2 shows a sketch of large pyramidal cells oriented vertically through the layers of the human cerebral cortex. Excitatory neurotransmitter receptors tend to be clustered distally in the dendrites and inhibitory receptors closer to the cell body. Excitation of the dendritic membrane generates a net flow of negative charge into the extracellular space of the upper cortical layers. Inhibition of the proximal cell membrane produces a net positivity in the extracellular space at deeper layers. The resulting extracellular dipoles tend to be distributed vertically through the depth of the cortex. Multiplied across millions of neurons, this stratification of electrical charge produces the scalp EEG.
Amplification and electrical noise
A 50 μV EEG signal at the scalp is far smaller than the many sources of noise that threaten to disrupt or obliterate it and is subject to distortion at many stages of the recording process. First, the signal must be amplified many thousands of times for proper analysis; the multiplication factor is called gain. But amplification of the EEG is straightforward; more bothersome is the large range of other electrical noise (from the body and the external environment) that can swamp the EEG entirely (Fig. 3). Certain electrical activity (from muscle contraction, cardiac conduction, and eye movement) represents noise when it appears in the EEG channels but it is a desired PSG signal when recorded in the appropriate PSG channels.
Because electrical noise can be orders of magnitude larger than EEG and other neurophysiological signals (see Fig. 3), it may seem surprising that we ever see the EEG at all. The key is that enormous as the interfering signals may be, many of them are almost identical on closely adjacent portions of the body. Thus, an exact subtraction of the activity from nearby electrodes can remove the noise those electrodes have in common (common-mode signal) and record only the activity that is different at those electrodes (differential-mode signal.)
Electrodes
However, the interface between the electrolyte and the metal electrode produces a chemical half-cell potential, which is, essentially, half of an electric battery and can be much larger even than the 60-Hz noise (see Fig. 3). Avoiding possible interference requires careful design of the amplifier, use of a low-frequency filter (LF), and matching electrodes to produce minimal size and variation in the half-cell potential.
The effectiveness of the differential amplifier depends on equal impedances at the 2 hot input terminals. Large and unequal electrode impedances can allow unpredictable amounts of common-mode noise to appear at the output. Most often, the common-mode noise is a 60-Hz artifact. Occasionally it represents other activity, including EKG and pickup from the ground electrode on the forehead. Fig. 4 is a complex example of the latter. Poor electrode connections were obscured because the 60-Hz filter was turned on, but this artifact was detected because of its pattern across multiple derivations. With fewer channels it might have been extremely difficult to recognize. The best way to avoid unpredictable common-mode artifacts is not to apply the 60-Hz filters routinely but to use good technique and keep the electrode impedances low.
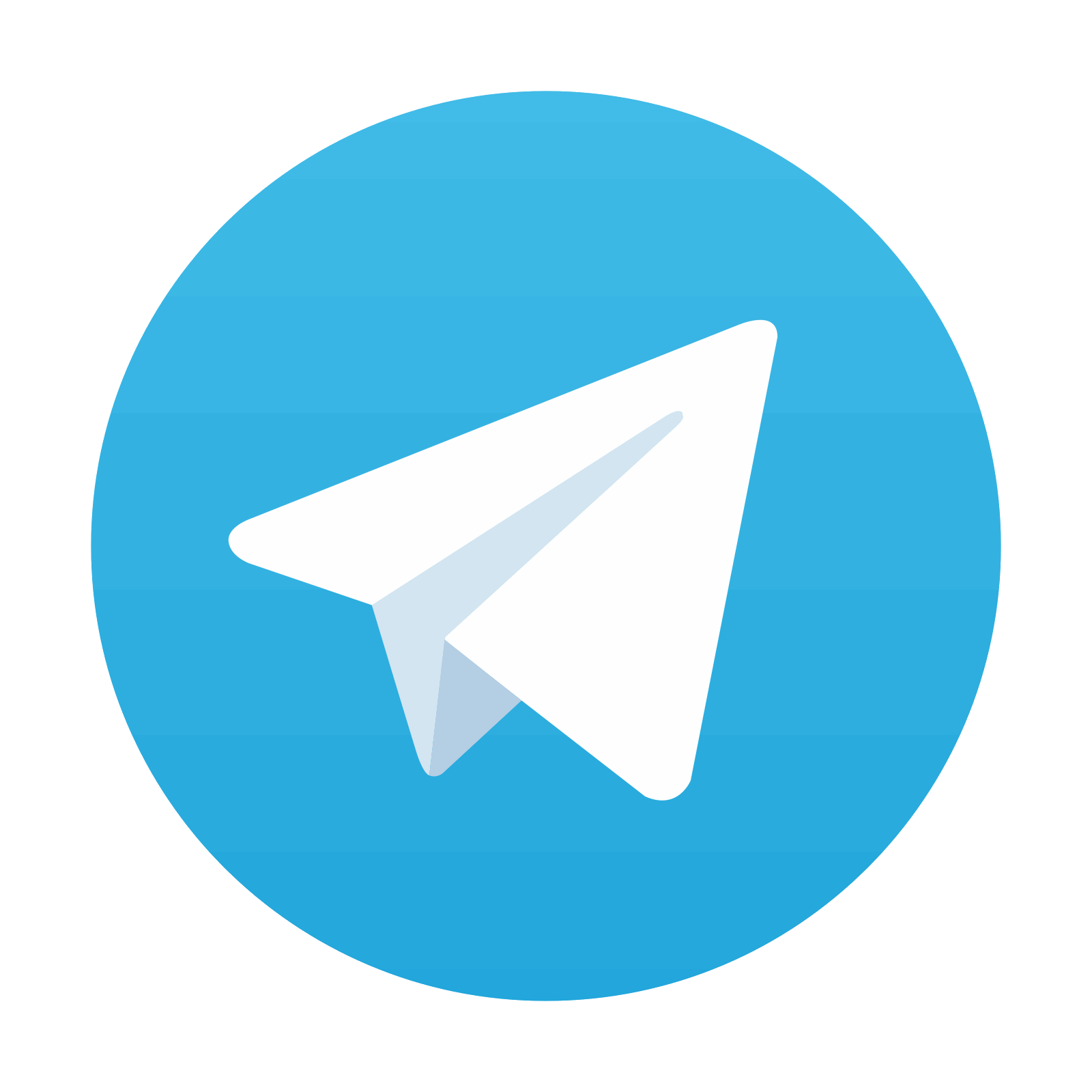
Stay updated, free articles. Join our Telegram channel

Full access? Get Clinical Tree
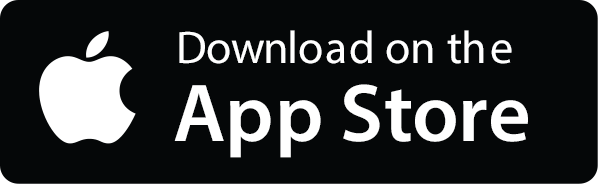
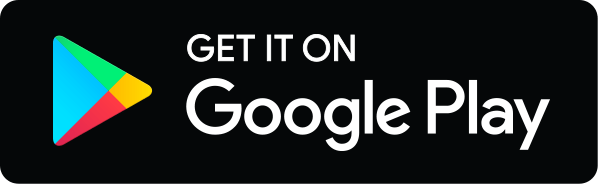