Fig. 48.1
Oxidative stress
Physiological levels of free radicals are essential for cell differentiation, cell growth, cell apoptosis, and immunity against invading microorganisms [21–23]. However, when these free radicals overwhelm the antioxidant defense system, oxidative stress occurs. ROS/RNS are increased during processes such as immunological reactions, inflammation, infections, and ischemia-reperfusion injury. Excess production of ROS/RNS can cause oxidative damage to DNA as well as modify proteins, carbohydrates, and lipids, resulting in cellular injury (Fig. 48.2). On the other hand, antioxidant levels can be reduced by the chronicity and magnitude of these processes and can be influenced by dietary intake of certain types of fat and antioxidant micronutrients, such as vitamin E, ascorbic acid, carotenoids, and selenium.
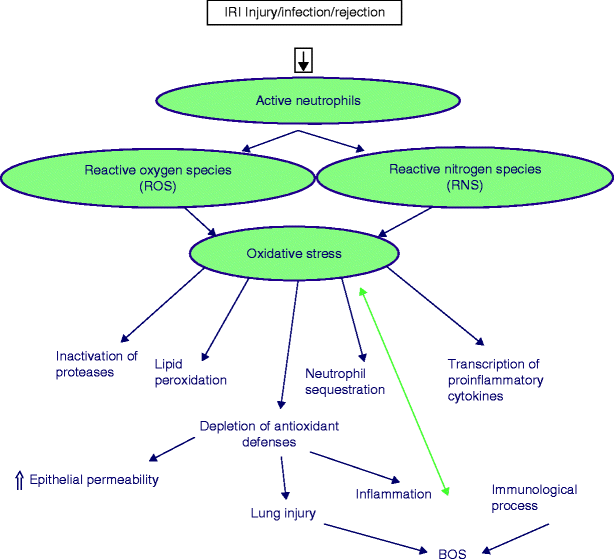
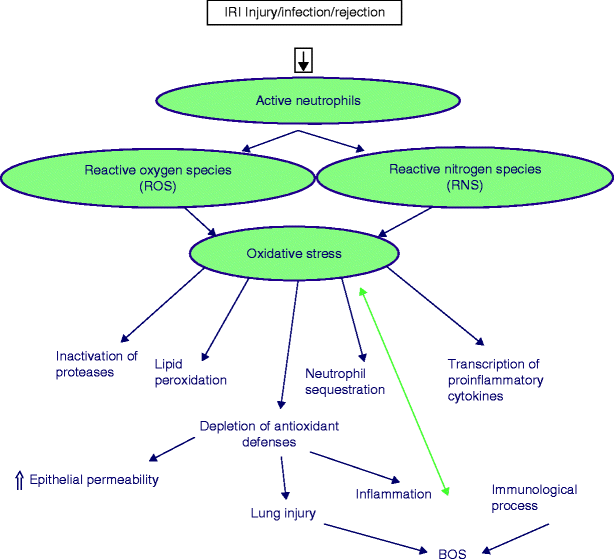
Fig. 48.2
Effects of oxidative stress
Measuring Oxidative Stress
Oxidative stress can be assessed by various laboratory analyses, for example, by measuring by-products of lipid, protein, and DNA oxidation. These include, lipid peroxidation metabolites such as plasma/tissue malondialdehyde (MDA) and 8-isoprostanes [24], protein oxidation parameters, such as protein carbonyls, total thiols, advanced oxidation protein products, and nitrotyrosine [25, 26], and measures of DNA damage, such as DNA strand breaks and guanine oxidation products (8-OHdG) [27, 28].
The antioxidant system can also be assessed by measuring antioxidant enzymes such as glutathione peroxidase (GPx), superoxide dismutase (SOD), and catalase (CAT) or by analysis of micronutrients such as antioxidant vitamin E, C, or carotenoids.
Evidence of Oxidative Stress in Lung Transplantation
Oxidative stress is a condition whereby prooxidants overwhelm the antioxidant defense system, and this may contribute to the pathogenesis of BOS by inducing increased tissue injury and inflammation.
Three studies have reported antioxidants in lung transplant recipients and measured oxidative stress parameters. In a small cross-sectional study, Madill [29] reported that lung recipients with severe stages of BOS were more oxidatively stressed compared to those patients with a milder stages of BOS and non-BOS lung recipients. This was indicated by increased BALF levels of LPO and oxidized glutathione. However, there were no significant differences in nutritional intake of vitamin A, C, or E or plasma antioxidant vitamin levels of retinol, vitamin C, or α- and γ-tocopherol among the three groups.
Williams et al. [30] in a prospective study also reported increased BALF MDA levels in lung recipients 2 weeks and 12 months post-transplant when compared to non-transplant controls (p < 0.05). The authors reported no significant improvement in antioxidant status (serum or BALF) from 2 weeks to over 1 year post-lung transplant, indicating that lung recipients remain oxidatively stressed.
Another study [31] performed in 15 cystic fibrosis patients taking vitamin supplements, including 8,000 IU (1,200 μg) vitamin A and 300 IU (248 mg) vitamin E, assessed antioxidant vitamin levels. Serum vitamin A and vitamin E levels were determined pre-transplant and again at approximately 15 months post-transplant. Pre-transplant serum vitamin A levels were within the normal range, whereas post-transplant, they significantly increased. Similar results were seen regarding serum vitamin E levels. No measurements were conducted in BALF to assess lung antioxidant status or oxidative stress. Supplementation in these lung transplant patients with both vitamin A and E (8,000 IU, 300 IU, respectively) remained the same pre- and post-transplant to prevent vitamin deficiencies. The increase in vitamin E and A serum vitamin levels post-transplant may have been due to increased compliance or reduced oxidative stress post-transplant due to lower lung infectious rates related to CF.
In summary, only three studies have been conducted examining oxidative stress and lung transplantation. Two studies documented an increase in lipid peroxidation along with a weakened antioxidant status in lung transplants recipients not taking vitamin supplementation. The third study examining CF patients indicated that antioxidant vitamin supplementation helps maintains vitamin levels.
In addition to ischemia-reperfusion injury and inflammation associated with rejection and infection, nutritional factors may contribute to oxidative stress by either creating a prooxidant status or affecting the antioxidant defense system. Prooxidant nutritional factors include obesity and/or malnutrition, as well as intake of dietary fat, particularly polyunsaturated fatty acid (PUFA). Conversely, nutritional factors contributing to the antioxidant defense systems are antioxidant micronutrients such as vitamins E, C, and carotenoids. In addition, antioxidant enzymes and trace elements such as selenium may also play a role.
Nutritional Factors and Oxidative Stress
Obesity
We are currently encountering an obesity epidemic [32]. Recent studies have indicated that decreased energy intake resulted in decreased oxidative stress, measured by decreased plasma MDA levels in non-obese subjects compared to obese participants [33].
Obesity is associated with a heightened state of inflammation [34, 35], resulting in oxidative stress. Increased body weight is positively associated with inflammation, measured by C-reactive protein levels (a biomarker of inflammation) in obese compared to non-obese individuals [36, 37].
Obesity is also prevalent in other solid organ transplant recipients [38–41]. However, in the lung transplant population, there is minimal information on the association of obesity and the development of BOS.
Kanasky et al. [42] examined 85 post-lung transplant recipients (34 % were overweight/obese) and determined that obesity had a negative effect on post-transplant survival. The most powerful predictor of mortality was BMI, with an increased risk of death of 7 % for each 1.0 unit (kg/m2) increase. These authors also reported that there was no difference in the development of BOS and/or infection in the obese compared to the non-obese group. Another study also reported a negative impact of high pre-transplant BMI [43]. Culver examined 46 lung transplant patients with BMI >30 kg/m2 and reported a significant increase in 90-day mortality in obese lung transplant patients when compared to non-obese [OR 3.16 (CI: 1.05–9.48)]. Previously, we have reported that high body mass index (BMI) pre-transplantation was shown to increase morbidity and/or mortality within 90 days post-lung transplantation [44]. A retrospective chart review [45] of 826 lung transplant recipients from 12 international transplant centers reported that substantial weight gain occurred within the first year post-transplant. In this study, higher weight gain was associated with better subsequent survival [45].
Taken together, these studies indicate that there is a high prevalence of obesity post-lung transplantation and that this may affect survival, although the results are mixed. Only one small retrospective study looked at the relationship between obesity and BOS and did not find any increased risk of BOS in obese subjects.
Malnutrition
In addition to obesity, malnutrition can also be associated with oxidative stress because of possible micronutrient deficiencies due to inadequate intake and/or malabsorption [46]. In the pre-transplant lung literature, the effect of malnutrition and oxidative stress has been reported mainly in cystic fibrosis (CF) patients [47]. CF patients have malabsorption of fat-soluble vitamins A, E, and carotenoids [48,49]. In addition, repeated or chronic infections as well as respiratory failure can increase catabolism and anorexia, contributing to malnutrition. Furthermore, chronic inflammation generates more ROS/RNS via neutrophil and macrophage activation and ‘respiratory burst’ [50, 51]. Therefore, the effect of reduced intake and increased malabsorption of micronutrient antioxidants, combined with greater demand of antioxidants for scavenging free radicals, will enhance oxidative stress in this patient population [50].
Some studies reported on malnutrition, malabsorption, and low micronutrient levels pre-transplantation, particularly the CF population. In general, in the studies reported, oxidative stress was shown to be elevated. However, there is a paucity of studies reporting on nutritional status, dietary intake, and plasma levels of antioxidant micronutrients in post-lung transplant patients. Nutritional intake also influences oxidative stress.
Nutrition Intake
Polyunsaturated Fatty Acid (PUFA)
The type of PUFA ingested in the diet can influence oxidative stress and the demand for antioxidants. It can also influence underlying inflammation. Long-chain PUFAs are more prone to lipid peroxidation (LPO) because they have more double bonds. The greater the number of double bonds in the PUFAs, the more susceptible they are to LPO [52–54].
Hydroxyl radicals produced by activated neutrophils initiate a free radical chain reaction by attacking the double bonds of PUFA within the membrane phospholipids. Omega-6 PUFAs, such as arachidonic acid, and omega-3 PUFAs, such as eicosapentaenoic acid (EPA) and docosahexanoic acid (DHA), are prone to LPO, particularly in an environment deficient in vitamin E. Both PUFA and vitamin E are influenced by diet.
In addition, the type of PUFA influences inflammation. Omega-3 PUFAs, such as α-linolenic acid, eicosapentaenoic acid (EPA), and docosahexaenoic acid (DHA), contained in walnuts, soybeans, flax seed oil, and fish [55], have antiinflammatory effects by decreasing the production of eicosanoids and leukotrienes [56]. However, omega-6 PUFAs, such as linoleic acid found in vegetable oils and arachidonic acid found in animal fat sources, are classified as proinflammatory [57] as they produce two potent inflammatory mediators, eicosanoids and leukotrienes [56]. Therefore, the type of PUFA can influence oxidative stress depending on its predisposition to lipid peroxidation and its effect on inflammation. Both PUFA and vitamin E levels can be influenced by dietary intake.
The University of Toronto Lung Transplant Program conducts about 100 lung transplants/year, and patients with cystic fibrosis (CF) represent 25 % of this population (UHN lung transplant data statistics; December 2011). These patients generally require high-calorie and high-fat nutrition care plans to optimize their nutritional status [58], along with pancreatic enzymes and vitamin supplementation to correct for malabsorption. In addition to the underlying disease, this regimen will influence oxidative stress depending on the proportion and type of fat absorbed and the ratio of PUFA:vitamin E. High fat requirements lead to increased intake of PUFA, which leads to increased oxidative stress.
Because of its antiinflammatory effect, omega-3 PUFA is often given to cystic fibrosis (CF) patients as a supplement. Omega 3 supplements contain vitamin E to prevent LPO. In a Cochrane systematic review [59], results indicated that CF patients supplemented with ω-3 had (1) improved lung function, (2) improved clinical status, (3) reduced volume of sputum, and (4) increased essential fatty acids (efa) in neutrophil membranes. Few minor adverse effects were reported. Another study in CF patients found no change in oxidative stress with fish oil supplementation [60], suggesting that vitamin E from supplementation was sufficient to prevent lipid peroxidation. Studies on the effect of ω-3 PUFA supplementation have not been reported in the lung transplant population. Antioxidant intake and/or supplementation can also influence oxidative stress.
Antioxidants
Observational studies examining a possible protective role of antioxidants in lung diseases have been summarized in several review articles [61–63]. Dietary studies form the bulk of these reports.
Current dietary guidelines from Health Canada Eating Well with Canada’s Food Guide recommend seven food guide servings of fruit and vegetables per day (HC Pub:4651; Cat: H164-38/1-2007E SSBN:0-662-44467-1). Consuming foods based on this recommendation will provide a diet rich in antioxidants, including vitamins C, E, and β-carotene, among others. Many dietary antioxidants work synergistically [64] to promote their protective effects as they exist in a natural environment and are biochemically balanced [65].
To our knowledge, there are no studies examining fruit and vegetable intake in lung transplant recipients. However, in the patients with lung disease, diets rich in antioxidants have shown beneficial effects on lung function described as high maximal forced expired ventilation in 1 s (FEV1). Cross-sectional studies have been conducted on 12,000 subjects with various lung etiologies, indicating that intake of fruits and vegetables improves lung function [61–63, 66]. As well, large longitudinal studies have also reported a positive association between overall dietary intake of fruits and vegetables and FEV1 [67–71]. Furthermore, fruit and vegetable consumption has shown beneficial effects on decreasing respiratory symptoms and disease severity [72]. Even low intake of fruit and vegetables compared to no consumption achieved a positive effect on lung function [61]. Similarly, one longitudinal study [67] reported an inverse association (RR = 0.73) between fruit intake and the incidence of chronic non-specific lung disease.
Studies on the intake of fruits and vegetables and the effect on oxidative stress measurements have been conducted. In a crossover study [73], increased consumption of ten servings of fruits and vegetables resulted in a significant reduction of oxidative stress measured as oxygen radical absorbance capacity (ORAC) from baseline to post-consumption [73].
Dietary studies examining the association between lung function (described as high maximal FEV1) and intake of individual antioxidants provide conflicting results. Indeed, while some cross-sectional studies found a positive association between vitamin C and lung function [74–77], along with two longitudinal studies [69, 70], others failed to find any such association [67, 78].
The evidence for intake of vitamin E is also conflicting. Cross-sectional studies [74, 75, 78] and one longitudinal study [69] report a positive association between intake of vitamin E and lung function, whereas one cross-sectional [76] and one longitudinal study report no association [79].
Although less well studied, research indicates that intake of β-carotene shows similar trends. Two cross-sectional studies [76, 80] along with a longitudinal study [69] indicate a beneficial association, whereas one cross-sectional study reports no benefit [67].
Studies involving patients with a variety of lung diseases have reported positive associations between plasma vitamin E, vitamin C, and β-carotene antioxidant levels and FEV1 [79, 81].
Supplementation studies in patients with lung diseases have reported very disappointing results. The Alpha-Tocopherol, Beta-Carotene Cancer Prevention (ATBC) study [82, 83] describes a RCT of 28,000 male smokers who were supplemented with a daily dose of 50 IU of vitamin E, 20 mg β-carotene, both, or placebo for 5–8 years. Unexpected results indicated that vitamin E supplementation, increased the risk of death from hemorrhagic stroke, and β-carotene increased lung cancer mortality and ischemic heart disease.
The CARET study [84] tested effects of combined treatment of β-carotene (30 mg/day) and retinyl palmitate (25,000 IU/day) in 18,000 men and women with a history of smoking. Results from this study found increased risk of both lung cancer and coronary artery disease in treated patients compared to controls.
Therefore, most of these epidemiological studies showed some beneficial effect from antioxidants on various respiratory diseases. However, intervention studies were disappointing. Presently, minimal studies examining antioxidant status in lung transplant patients have been undertaken. One study [85] reported low BALF ascorbic acid and glutathione levels in BOS lung recipients when compared to non-BOS recipients. The second study [30] indicated elevated plasma and BALF MDA levels and low serum and BALF ascorbic acid levels up to 12 months after lung transplantation. No dietary intake assessment was performed, and no intervention studies were conducted.
In the lung disease population, increased oxidative stress has been adequately documented in pre-transplant patients [47, 50, 86–91] as well as early post-lung transplantation in relation to ischemia-reperfusion injury (IRI). However, oxidative stress in long-term lung recipients is not well studied [29, 30].
It is important to keep in mind that, in the broader context, research studies examining antioxidant supplementation in patients with a variety of disease conditions such as atherosclerosis [92] and Alzheimer’s disease [93] have also been disappointing, underlying the difficulties regarding decisions for the dosing, timing, type and combination of supplements, duration of the intervention, and outcome measures. Results from primary and secondary intervention trials with 400 IU vitamin E every other day plus 500 mg vitamin C daily have reported increased mortality in both healthy controls and patients with diseases [94–96]. The evidence is even more convincing that antioxidant supplementation with the goal of reducing the risk of cardiovascular disease is not supported by the findings from current randomized controlled studies [97, 98]. Similarly, 400 IU vitamin E every other day along with daily 500 mg vitamin C supplementation, neither alone nor together, reduced the risk of prostate or total cancers [99–101]. However, some of these studies have been criticized for not examining an oxidative stress biomarker, thus making it difficult to identify individuals who may have benefited from supplementation [102]. Therefore, based on these past studies, optimal antioxidant vitamin supplementation studies may be very challenging to design.
Another potential intervention would be a dietary modification [103]. Initiating nutrition strategies to improve the total antioxidant capacity (TAC) of lung recipients’ diets represents a possible dietary alteration. Some authors agree that improving the patients’ overall nutrition intake may prove more beneficial than antioxidant supplementation [104]. As well, some authors have reported that high TAC foods (consisting of red berries, spinach, coffee, olive oil, among others) can minimize inflammation [105–109]. More recently, Giuseppe reported that subjects consuming higher TAC foods demonstrated a significant improvement in FEV1 when compared to those with lowered intakes of TAC foods [110]. These types of diets may be more beneficial than consuming additional pills and may have other beneficial compounds that have not yet been identified [111].
However, before recommending antioxidant supplementation in lung transplant recipients, we should assess its benefit and determine what appropriate dose and combination would be most successful, as well as the duration of treatment [112, 113]. We may also want to determine if we could identify the ‘more oxidized’ lung transplant patient at an earlier stage for supplementation with extra antioxidants [114]. Furthermore, we will need to examine if supplementation leads to minimization of the inflammatory process evident in chronic rejection, a condition that significantly decreases long-term outcomes in lung transplant patients. If so, would this reduce oxidative stress and, as a result, have a clinical impact on FEV1 and the development of BOS?
Conclusion
Oxidative stress can be generated early post-lung transplantation in relation to ischemia reperfusion injury and longer term with infectious episodes and /or chronic rejection. Nutritional factors as well as nutritional intake may also impact the state of oxidative stress in this patient population. Very little has been published on nutritional factors and oxidative stress in the lung transplant population. However, preliminary research results have been most noticeably encouraging to conduct more extensive clinical studies to determine if oxidative stress post-lung transplantation leads to improved clinical outcomes. Larger and more detailed studies in lung transplantation are needed.
References
1.
2.
3.
Hertz MI. Immunosuppression for prevention of bronchiolitis obliterans. Curr Opin Transpl. 2000;5(4):402–6.CrossRef
4.
5.
6.
7.
Girgis RE, Tu I, Berry GJ. Risk factors for the development of obliterative bronchiolitis after lung transplantation. J Heart Lung Transplant. 1996;15:1200–8.PubMed
8.
9.
Vos R, Vanaudenaerde BM, Ottevaere A, Verleden SE, De Vleeschauwer SI, Willems-Widyastuti A, et al. Long-term azithromycin therapy for bronchiolitis obliterans syndrome: divide and conquer? J Heart Lung Transplant. 2010;29(12):1358–68.PubMedCrossRef
< div class='tao-gold-member'>
Only gold members can continue reading. Log In or Register a > to continue
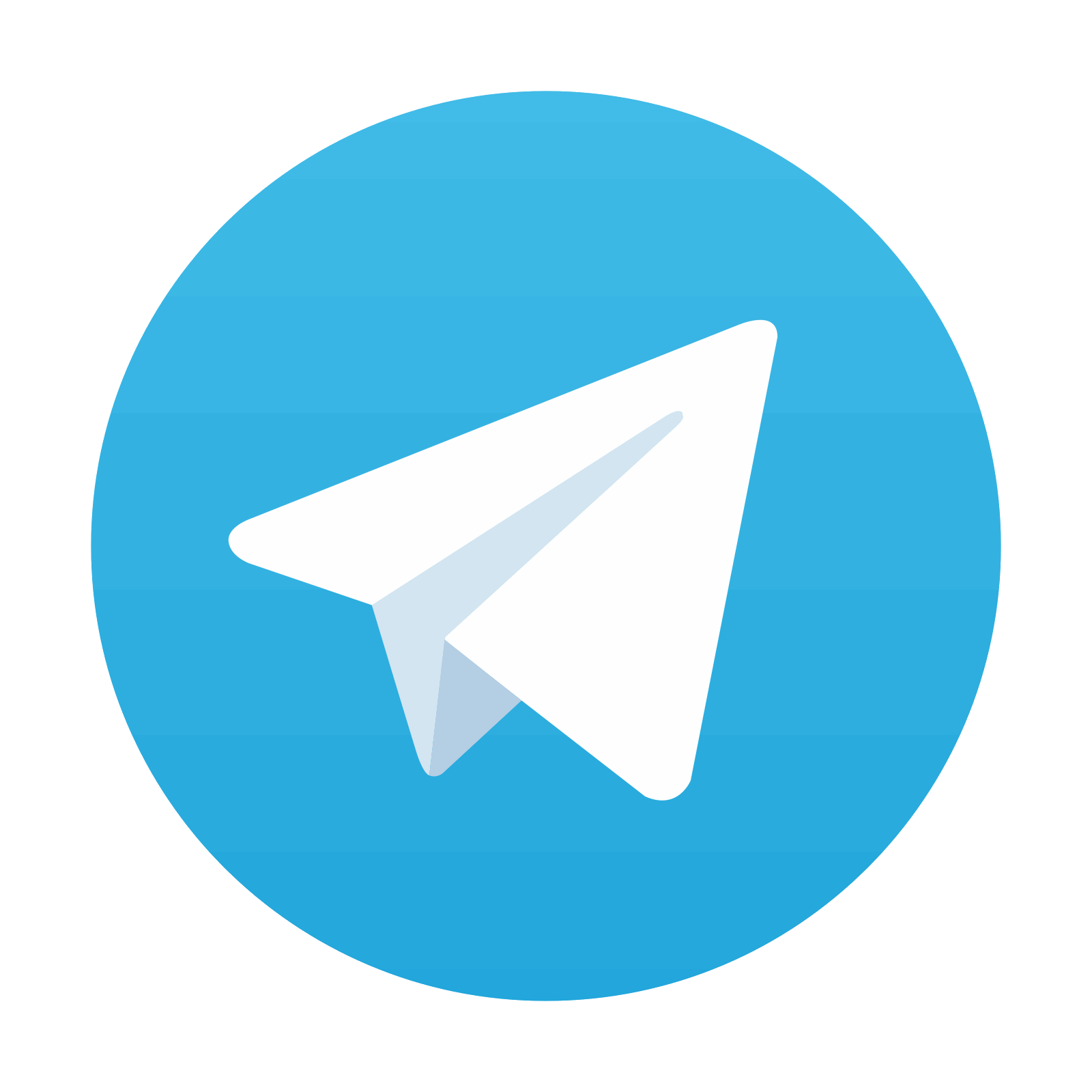
Stay updated, free articles. Join our Telegram channel

Full access? Get Clinical Tree
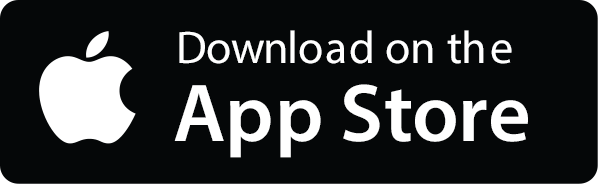
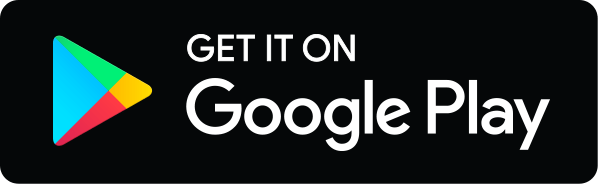