Author
Cohort
FO threshold
Outcome
Gillespie et al. [18]
CRRT
10%
OR death 3.02 > 10% FO
Foland et al. [19]
CRRT
10% increment
1.78 OR death for each 10% FO increase
Goldstein et al. [20]
CRRT
20%
<20% FO: 58% survival
>20% FO: 40% survival
Hayes et al. [21]
CRRT
20%
OR death 6.1 > 20% FO
Akcan-Arikan et al. [16]
PICU
15%
Longer duration of mechanical ventilation
Worse oxygenation
Valentine et al. [22]
PICU
10% on day 3
Longer duration of mechanical ventilation
Sinitsky et al. [23]
PICU
<5,<10, <15,>15 at 48 hour
Higher OI, longer duration of mechanical ventilation
Ingelse et al. [24]
PICU
N/A
Longer duration of mechanical ventilation
In PARDS, positive fluid balance is associated with a decrease in oxygenation and ventilator-free days [17]. Increased lung water retention is likely an explanation of worsening in oxygenation by causing pulmonary edema, which, in turn, may result in worsening compliance as well as predisposing to ventilator-associated conditions, such as infections. As liberal administration of fluid to patients with respiratory failure could lead to fluid accumulation with deleterious consequences on oxygenation, length of ventilation, and ICU stay, an enhanced awareness of fluid exposure is needed. Unfortunately, despite recommendations for conservative fluid strategy, intensivists still administer large amounts of fluid in pediatric acute lung injury [17]. It is not uncommon for fluid orders to be written without dedicated attention to other fluid intake driven by the obligate administration of medications, especially in the form of drips. Conversely, indiscriminate fluid restriction could also be detrimental, as it could lead to hypoglycemia, especially in younger patients with limited endogenous stores and gluconeogenesis capacity, which renders them more dependent on exogenous glucose infusion rates [27]. Strategies to better manage fluid delivery include implementation of a preemptive fluid treatment bundle to prevent fluid overload in children with PARDS and sepsis [27]. Recently, there has been a practice drift to only use isotonic fluid for maintenance fluid administration in the PICU due to observational studies reporting high prevalence of hyponatremia with hypotonic fluid use [28]. Since the most frequently used isotonic fluid, 0.9 N sodium chloride (“normal saline”), includes supraphysiological quantities of sodium and chloride, sodium overload could have unintended consequences of worsening fluid accumulation. Moreover, high chloride levels have been associated with worse AKI and worse outcomes in sepsis as well as CRRT patients, and may increase minute ventilation to compensate for nonanion gap metabolic acidoses [29, 30].
Critically ill adults with Acute Lung Injury randomized to conservative fluid strategy had better oxygenation, shorter length of mechanical ventilation, and shorter ICU stay in the National Heart, Lung, and Blood Institute (NHLBI) ARDS Network’s FACTT (Fluid and Catheter Treatment Trial) trial [31]. However, conservative fluid management may not be appropriate for all patients. The conservative strategy was associated with long-term neurologic morbidity [32]. Furthermore, two subphenotypes with treatment response to fluid restrictive fluid strategy in opposite directions are recognized in adult patients with ARDS, which suggests differing underlying pathophysiology between the subphenotype groups [33]. Subphenotype 2 was characterized by higher inflammatory biomarkers and hypotension, and a fluid-conservative strategy was associated with 40% mortality, whereas a fluid-liberal strategy was associated with 50% mortality. Subphenotype 1 was a less “inflammatory” biomarker phenotype and had 26% mortality with fluid-conservative management and 18% mortality with a fluid-liberal strategy. While it is plausible to propose that there could be similar subphenotypes in children with PARDS, there is no evidence as to which fluid administration protocol would be beneficial in a more or less “inflamed” subphenotype in pediatrics.
Pediatric acute lung injury consensus conference recommendations for fluid management in PARDS
PALICC fluid recommendations [34] | |
---|---|
Total fluid goal | We recommend that pediatric patients with PARDS should receive total fluids to maintain adequate intravascular volume, end-organ perfusion, and optimal delivery of oxygen |
Goal-directed fluid management | After initial fluid resuscitation and stabilization, we recommend goal-directed fluid management. Fluid balance should be monitored and titrated to maintain adequate intravascular volume while aiming to prevent positive fluid balance |
Fluid titration | We recommend that fluid titration be managed by a goal-directed protocol that includes total fluid intake, output, and net balance |
Diuresis and Mechanical Fluid Removal
Careful attention to fluid accumulation and early intervention with goal-directed use of diuretics may be beneficial in adults with ARDS. Diuresis can be augmented with albumin in patients with hypoalbuminemia [38, 39]. However, diuretics as currently used in the PICU might not be adequate to achieve fluid balance goals; despite considerable doses of diuretics, pediatric acute lung injury patients often fail to achieve negative fluid balance by PICU day 3 [22]. Management of hypoxemia in severe PARDS with early institution of CRRT should be considered in order to prevent fluid overload, especially in cases where there is relative oliguria, or when fluid intake (due to ongoing resuscitation, need for blood products, or large number of medications) far exceeds urine output. Unfortunately, restitution of euvolemia has not been shown to negate the negative outcome associations with fluid accumulation, suggesting that it is not the edema per se that leads to adverse outcomes but possibly a hidden confounder – such as severity of illness not captured by the scores used currently – that is responsible for the outcomes in observational studies. For instance, in pediatric Extracorporeal Membrane Oxygenation (ECMO) patients on RRT or mechanical fluid removal, reversal of fluid overload by fluid removal did not improve outcomes [40]. Alternatively, this observation might also suggest that interventions aimed at prevention of fluid accumulation may be more effective compared to fluid removal. Perhaps even basic measures such as using maximally concentrated feeds and medications early on, in addition to being mindful of the free water content of IV fluids and parenteral nutrition compared to enteral feeds, may have a greater impact on preventing fluid accumulation.
Acute Kidney Injury in PARDS
The association between AKI and lung dysfunction has been extensively reported in adults and recently in children [41–45]. Patients with AKI have longer duration of ventilation, and patients with acute respiratory failure have high rates of AKI. Organ crosstalk between kidney and lung, with spillover of cytokines and damage-associated molecular patterns into the circulation mediating inflammation in the distal organ, has been proposed as the link between AKI and propagation of lung injury. More importantly, this relationship seems bidirectional in nature. Established AKI could lead to increased pulmonary vascular permeability and leukocyte trafficking, promoting lung injury. A high incidence of AKI has been reported in adult patients with ventilator-associated pneumonia. High mean airway pressures can cause proximal tubular apoptosis under experimental conditions. Fluid overload, which frequently accompanies oliguric AKI, can lead to increased venous pressures, which in turn can lead to elevated renal venous pressure, compromising renal perfusion. This mechanism is likely to be augmented in invasively ventilated patients who are on higher mean airway pressures, such as those with severe PARDS. Prudent attention to the management of mechanical ventilation and lung-kidney interactions is required to address this deleterious organ crosstalk. What is still not clear and requires further study is whether oliguria associated with AKI leads to pulmonary fluid accumulation and hence worsening lung injury or whether prevention of AKI could modulate the inflammatory profile and prevent distant organ injury. In a prospective multicenter study, one in four ARDS patients had severe AKI on days 1–3 of PARDS. The prevalence of AKI increased when creatinine was adjusted for fluid accumulation. ICU mortality increased 8% for each 20 ml/kg increase in cumulative fluid balance in children with PARDS if they also had coexisting AKI. Interestingly, on day 3, patients with AKI had slightly higher fluid intake compared to those without AKI despite having similar output [46]. Evaluation for drivers of fluid intake could allow identification of modifiable causes – such as inadvertent liberal fluid prescription – and, thus, opportunities for improvement in management. What is more, neither AKI in its modern definition nor degrees of fluid overload are components of illness severity in currently used organ dysfunction systems. Data from ventilated critically ill adults suggest pulmonary edema/fluid accumulation contributes to up to 50% of ventilator-associated events [47]. The association among fluid overload, AKI, and pulmonary morbidity can easily be overlooked unless they are transformed into objective, quantifiable, and trackable metrics that also incorporate visual cues [48].
AKI typically occurs early in the PICU stay, with most cases developing in the first 3 days and more than 80% happening in the first week of ICU admission. Positive fluid balance also maps to this period, usually peaking around PICU day 5. Both early fluid overload and net positive fluid balance for each day are associated with increased respiratory and global morbidity [16, 22, 23]. Serum creatinine, the current functional marker of AKI, changes with the total body water in which it is dissolved, such that rapid fluid accumulation can lead to a dilutional effect on serum creatinine levels and mask AKI [49]. A recent international multicenter study of pediatric AKI has demonstrated that over 2/3rd of cases diagnosed utilizing oliguria criteria of the current consensus criteria would have been missed if only elevations in serum creatinine were taken into consideration [12]. Adding to the complexity of exploring this relationship is the lack of consensus approach to quantify fluid balance. Weights are difficult to obtain reliably in the critically ill, and as patients often lose weight during their PICU stay due to catabolism and loss of lean body mass, the degree of fluid accumulation is often underestimated.
It is well recognized that the bulk of the fluid accumulation occurs in the first week of ICU admission. The preponderance of pediatric AKI during the initial phase of the PICU course suggests that fluid overload and AKI are closely related. In fact, the timeline of fluid overload occurrence directly overlaps with critical illness–associated pediatric AKI, which also largely happens in the first week of ICU admission. This temporal relationship has led many to surmise fluid overload to be an excretory problem and a surrogate indicator for oliguric AKI (at least relative oliguria). It is quite possible that fluid overload is a natural consequence of AKI, particularly oliguric AKI, that might be undetected, especially if urine output is not taken into consideration.
Nutrition in PARDS
Improved energy and protein adequacy, when administered via the enteral route, are associated with lower ICU mortality in PARDS [4]. Mechanisms for improved patient outcome with improved EN adequacy relate to both nutritive and non-nutritive effects of adequate EN. Direct nutritive consequences of inadequate nutrition are due to insufficient protein substrate for production of acute-phase and immune proteins, and loss of lean body mass. Utilization of lean muscle mass to recruit amino acids pool for synthesis of acute-phase and immune proteins results in loss of respiratory and cardiac muscles and difficulty with ventilator weaning. Non-nutritive benefits of EN include improved intestinal barrier function, improved immune function, and maintenance of intestinal microbiome diversity. Therefore, “optimal” nutrition can be defined differently based on the specific outcome or target. Optimal nutrition can be defined as: sufficient EN to meet metabolic demands, sufficient EN to maintain lean body mass and functional recovery, sufficient EN to maintain neurocognitive development, sufficient EN to maintain intestinal barrier functions, or adequate enteral composition to maintain microbiome diversity.
Shifts in Metabolism During Pediatric Critical Illness
An understanding of normal nutritional needs of children and the metabolic response to critical illness helps guide nutritional support recommendations in critically ill children. Critical illness induces a catabolic state with cessation of normal growth [1, 50]. Systemic metabolism shifts away from growth to production of acute-phase proteins, enzymes, and glucose [50, 51]. If this process is prolonged, there is progressive depletion of nutritional resources, which leads to muscle wasting, diminished immune function, and poor wound healing. Pediatric patients with pre-existing chronic illnesses or pre-existing malnutrition are particularly susceptible to adverse sequelae of inadequate nutrition delivery due to their limited macronutrient and micronutrient reserves [52]. In addition, many children develop undernutrition during their PICU stay. While up to 30% have pre-existing acute or chronic malnutrition at PICU admission, up to 58% are malnourished at PICU discharge [3, 6, 53]. When mechanically ventilated, both obese and underweight children are at increased risk compared to normal weight children for worse outcomes [54]. Therefore, comprehensive nutritional support strategies should include early screening and diagnosis of malnutrition (undernutrition and obesity) [55]. In the setting of PARDS, loss of lean body mass may impact respiratory muscle function and prolong ventilator dependence. In addition, therapies commonly used in PARDS patients, such as ECMO and CRRT, may increase nutrition needs or increase risk for specific nutrient deficiencies.
Nutrient Needs During Pediatric ARDS
Protein
Pediatric Acute Lung Injury Consensus Conference and Society of Critical Care Medicine/American Society for Parenteral and Enteral Nutrition recommendations for nutrition risk screening and nutrition management
Directive | PALICC [34] | SCCM/ASPEN [58] |
---|---|---|
Nutrition screening | No recommendation | Early screening of nutritional status to identify patients at high nutritional risk |
Nutrition management | ||
Nutrition plan | We recommend that pediatric patients with PARDS should receive a nutrition plan to facilitate their recovery, maintain their growth, and meet their metabolic needs | Use of predictive equations to determine energy requirements without the addition of stress factors when indirect calorimetry is not available Target energy for the first week of critical illness should be at least 2/3 of total energy requirements Minimum protein delivery is 1.5 g/kg/day |
Enteral nutrition | We recommend that EN, when tolerated, should be used in preference to parenteral nutrition | EN is the preferred route of nutrition delivery |
Goal-directed nutrition management | We recommend that EN monitoring, advancement, and maintenance should be managed by a goal-directed protocol that is collaboratively established by the interprofessional team | EN should be initiated within 24–48 hours of ICU admission, and advanced by a stepwise institutional algorithm |
Energy
Multiple retrospective studies report an association between early energy adequacy and reduced mortality in pediatric critical illness [3, 69]. However, calculation of energy requirements during pediatric critical illness is challenging. The influence of critical illness on metabolism in children is unpredictable, and energy requirements change over the course of the ICU stay [70–72]. And yet, an accurate and precise energy prescription is important because both underfeeding and overfeeding are associated with worse clinical outcomes [3, 58, 72]. Complications associated with underfeeding included delayed ventilator weaning, impaired protein synthesis, organ failure, and an increased risk of sepsis [7, 10, 73, 74]. Overfeeding is associated with delayed ventilator weaning, lipogenesis, hepatic dysfunction, hyperglycemia, increased mortality, and prolonged hospitalization. Several potential mechanisms exist for the negative impact of overfeeding on patient outcome, such as increase in carbon dioxide production, increased intolerance of EN and PN, refeeding syndrome, azotemia and metabolic acidosis from excess protein administration, hepatic steatosis from excess glucose delivery, hyperglycemia, hypertriglyceridemia, and (on the cellular level) the suppression of autophagy [72, 75–77]. Common factors, which may raise or lower total energy expenditure in PARDS, include fever, sedation, temperature support, paralytics, renal replacement therapies, ventilator support, and ECMO. The inter- and intra-individual variation in energy expenditure over the course of the ICU stay necessitates frequent reassessment and adjustment of nutritional support to avoid both under- and overfeeding.
Predictive equations to determine energy needs were developed in populations of patients and are inaccurate when applied to individual patients as they do not sufficiently account for individual variation in energy expenditure. Methods to correctly determine daily energy requirements such as indirect calorimetry (IC) are labor intensive and not available at all institutions [78]. Nor do clinical exams correctly identify patients who are hypometabolic, normometabolic, or hypermetabolic [72]. When available, IC should be used to determine energy expenditure and guide energy prescription, especially in patients at nutritional risk [58]. According to current U.S. guideline recommendations, indirect calorimetry is specifically recommended in the setting of BMI <5th percentile or > 85th percentile, a > 10% change in weight, prolonged ventilation, prolonged muscle relaxation, thermal injuries, oncologic diagnosis, or neurologic injury with dysautonomia [58, 59]. When IC is not available or impractical to perform, predictive equations such as Schofield or World Health Organization should be use without the addition of stress factors to avoid the risks of overfeeding (see Table 12.3) [58]. As most CRRT solutions contain bicarbonate, the impact of bicarbonate flux needs to be taken into consideration if IC will be used in patients on CRRT.
Energy should be provided as a mixture of carbohydrates and lipids with provision of adequate protein to preserve lean body mass. A balanced prescription of carbohydrates and lipids to provide energy in addition to appropriate protein provides sufficient macronutrients while avoiding the potential complications of excess protein, carbohydrates, or lipids. Excess carbohydrates should be avoided as they are converted to lipids with the byproduct of carbon dioxide formation, potentially prolonging mechanical ventilation [79]. Lipid turnover is increased in pediatric critical illness, and lipids are generally limited to 30–40% of calories. An inadequate lipid prescription may lead to rapid development of fatty acid deficiencies in infants and children who at baseline have limited fat stores.
Early Nutrition Support
Delivery of early EN within 48 hours of ICU admission is associated with improved tolerance of future EN and lower 90-day mortality in general critical illness, mechanically ventilated children, and in PARDS [3, 69]. Multiple retrospective studies in critically ill children demonstrate associations between reduced mortality with improved energy and protein adequacy [2, 3, 69]. In a prospective, international study of nutritional practices in 500 mechanically ventilated children, Mehta et al. found decreased odds of mortality for every tertile of increased energy delivered. This relationship was observed when increased energy was enterally, but not parenterally delivered [3]. Wong et al. examined 107 children with PARDS and identified that earlier energy and protein intake were associated with reduced ICU mortality, while protein adequacy was also associated with increased ventilator-free days [4]. Optimizing early, safe EN, rather than delivery of energy or protein via other routes, is important for improving outcomes of PARDS, and is consistent with both the 2015 PALICC Guidelines and the 2017 ASPEN/SCCM Nutrition Support Guidelines [34, 58].
Route of Enteral Nutrition
There are limited data to guide decisions regarding gastric versus postpyloric feeding route. In a study of 74 critically ill children randomly assigned to gastric or postpyloric feeds, no difference in complications was found between study groups. Patients receiving postpyloric feeds did receive more of their daily prescribed calories [80]. Nonetheless, it is not clear if this benefit outweighs the delays to EN initiation to place and confirm position of postpyloric tubes, or interruptions to EN to replace a dislodged or occluded postpyloric tube. Nasogastric tubes are invariably more rapidly placed, and easier to replace. Data in adult ICU patients are also inconclusive regarding benefits of postpyloric tubes. A large meta-analysis of adult patients with severe traumatic brain injury demonstrated a decreased risk of pneumonitis with postpyloric feeds [81]. Other researchers found an increased risk of gastrointestinal complications when postpyloric feeds were used in septic patients or patients on epinephrine [82, 83]. No studies so far have specifically evaluated gastric versus postpyloric feeds in the setting of PARDS.
Parenteral Nutrition in PARDS
Current standard practice in the USA is to reserve PN for situations in which EN fails or is not possible. The Pediatric Early versus Late Parenteral Nutrition in Intensive Care Unit (PEPaNIC) study was an international, multicenter, randomized controlled trial comparing early versus late initiation of PN in critically ill children [84]. The PEPaNIC Trial randomized 1440 children from newborn to 17 years of age to early versus later PN supplementation. They found no difference in mortality between the study allocation groups, but an increase in hospital-acquired infections in the early PN group [85]. This study was not limited to children with PARDS, but supports recommendations that PN should only be used in PARDS patients who fail to tolerate EN or cannot be enterally fed in the first week of ICU stay. Research from neonatal intensive care supports the early use of trophic EN with PN supplementation to support bowel health and meet metabolic needs [86]. The use of combined EN and PN nutrition support, beginning with the first hours to days of life in preterm infants, has been associated with improved growth, improved neurodevelopment, improved EN tolerance, and decreased morbidity at both intermediate- (18 months) and long-term (5 year) follow-up [87–90]. It remains unclear if older or term infants with PARDS might benefit from a similar early PN strategy with regard to long-term neurocognitive outcomes. Despite the results of the PEPaNIC study, equipoise remains pertaining to the PARDS population. The optimal macronutrient dose, timing, and formulation of EN and PN support are yet to be elucidated as increasing evidence demonstrates links among the immune system, homeostasis, and nutritional intake [81, 91–94]. Questions remain whether early PN is of benefit or harm in PARDS, or if various IV lipid formulations or supplements could be of benefit. The current clinical focus is to provide sufficient energy and protein preferentially by the enteral route when safe to do so, until further studies are completed.
Failure to Receive Enteral Nutrition
Median delivery of enteral nutrition is 40–75% of goal during the first week of critical illness [3, 5–10]. Reasons for failure to receive EN are broadly categorized as medical contraindication, prescriber discomfort, and frequent interruption [7, 9, 95, 96]. Noninvasive ventilation (NIV) is increasingly used as a first-line respiratory support modality in PARDS, and is associated with worse nutritional adequacy [97]. We do not know if perceived potential benefits to NIV, used to avoid invasive mechanical ventilation, are outweighed by risks of underfeeding. Cited relative contraindications to EN often include a need for volume restriction, hemodynamic instability, and ill-defined feeding intolerance. Hemodynamically unstable adults requiring vasopressors have lower mortality when receiving early EN [98]. Large database studies do not demonstrate increased adverse outcomes in children fed enterally while on vasoactive infusions; however, in small studies, children with hemodynamic instability who develop complications of EN have worse outcomes than children who were never enterally fed [82, 99]. Therefore, provider reluctance to initiate or advance EN in the setting of hemodynamic instability may be justified [82]. Research is needed to better identify patients who will and will not develop complications from EN when on vasoactive infusions.
Feeding intolerance is the most frequent cause of interruption to EN and occurs in 45–57% of critically ill children [9, 100]. There is immense variability in clinician assessment of feeding intolerance and frequently used clinical criteria such as emesis, bowel sounds, abdominal exam, gastric residual volumes, diarrhea, and lactate levels are either imprecise, subjective, or have poor interrater reliability [9, 95, 101, 102]. The definition of feeding intolerance is widely variable between providers and centers, and no standardized definition or “score” is validated. Delayed gastric emptying occurs in up to 50% of critically ill children, yet remains underrecognized as a clinical concern in the PICU [103]. Poor intestinal motility is often multifactorial in nature, due to opioid drips and other elements of critical illness. Only erythromycin and metoclopramide are FDA-approved as promotility agents to treat gastric and intestinal dysmotility during pediatric critical illness and have variable success at improving poor motility in the PICU. Newer promotility agents such as cholecystokinin receptor antagonists, ghrelin, and methylnaltrexone, in the setting of opioid-induced dysmotility, are under evaluation in pediatric critical illness [104, 105]. Early initiation of a bowel regimen to prevent constipation and subsequent feeding intolerance should be prescribed and the patient monitored for diarrhea and constipation, especially for children on opioid drips. Poor intestinal motility, delayed gastric emptying, and lack of an appropriate bowel regimen may delay achievement of goal EN.
Objective biomarker-based tools for the safe initiation and advancement of EN would decrease barriers to nutritional support and improve delivery of EN, but no such tools currently exist. Ideally, biomarkers to guide EN advancement would predict which patients would experience complications of EN in the first week of ICU stay, and identify patients who might benefit from PN to achieve macronutrient goals. Procedures are also a frequent cause of interruption of nutritional support [9]. Noninvasive ventilation and intensive therapies such as ECMO are associated with failure to achieve goal EN [106, 107]. One strategy successfully employed in adult ICUs to improve nutritional adequacy is a strategy of volume-based daily enteral feed goals rather than an hourly feed goal [108]. Volume-based orders prescribe a daily volume goal for EN, typically delivered over 18–20 hours rather than the traditional 24-hour delivery. This strategy automatically accommodates usual feed interruptions for procedures, so that total volume of prescribed EN is delivered when possible throughout the day. No matter the method, presence of a multidisciplinary team focused on nutrition support in the PICU is an important part of a successful nutrition program. Implementation of an early EN guideline improved percent of goal energy and protein achieved in multiple retrospective studies, likely due to perceived emphasis on nutrition in a particular PICU and change in clinician behaviors for prescribing nutrition [109, 110].
The Gut as the Motor for ARDS
Gastrointestinal Tract and Microbiome Influences Immune and Lung Function in ARDS
The gastrointestinal tract is a primary lymphoid organ, housing 70% of all of the body’s immune cells [111–114]. The intestinal microbiota plays an important role not only in the development of the immune system in infancy, but also in shaping systemic and pulmonary immune responses during critical illness [115, 116]. Diet is a potent determinant of intestinal microbiome diversity and of intestinal barrier function, and in murine models is more important than genetic background in predicting microbial community composition [117]. Carmody et al. found that rapid changes in diet resulted in rapid shifts in microbial composition [117]. In health, the gut microbiome helps to regulate lung immunity and host defenses through several mechanisms. Intestinal commensal (beneficial) bacteria act to directly counteract proinflammatory bacteria, decrease the overall inflammatory “tone,” and preserve intestinal epithelial barrier function, preventing the translocation of inflammation-inducing bacterial components [115, 116, 118, 119]. Dysbiosis, the imbalance in the gut microbiome characterized by increased relative abundance of pathobionts and decreased relative abundance of commensal bacteria, occurs in ARDS, and is further exacerbated by treatment factors such as antibiotic use, altered intestinal pH, and prolonged critical illness [115, 120, 121]. In pediatric critical illness, this dysbiosis is characterized by increased relative abundance of pathobionts such as Enterococcus and Staphylococcus, and decreased relative abundance of beneficial commensal organisms such as Ruminococcus and Faecalibacterium [120, 122]. In addition, virulence of Gram-negative pathobionts is enhanced when nutrient deprivation occurs during systemic stress or opioid exposure, a common clinical scenario in PARDS patients [123]. Through effects on the intestinal microbiome and the intestinal epithelial barrier, specific nutrients and pre- or probiotics may impact lung and systemic proinflammatory tone and neutrophil accumulation in the setting of PARDS [124–126]. Nonnutritive benefits of enteral feeding include reduction in proinflammatory signaling to the lung, an important potential therapeutic target in PARDS [111, 127, 128]. An area of emerging research surrounds the role of nutritional support and the intestinal microbiota in shaping systemic and pulmonary inflammation and immune responses in ARDS.
Immunonutrition
Despite initial encouraging results from small single-center studies of individual pharmaconutrients, multiple larger trials of combination pharmaconutrients designed to modulate the inflammatory response in critically ill adults have not shown benefits or have shown harm [129, 130]. Combination therapies have included: various antioxidants, arginine, glutamine, metoclopramide, ω-3 polyunsaturated fatty acids (PUFA’s), zinc, and selenium [131–137]. The Randomized Comparative Effectiveness Pediatric Critical Illness Stress-Induced Immune Suppression (CRISIS) Prevention Trial evaluated daily enteral zinc, selenium, glutamine, and IV metoclopramide in critically ill children. The primary outcome was the incidence of nosocomial infections. The trial was stopped early due to futility, although secondary analysis of the dataset does suggests further research is needed in patients with baseline immune dysfunction [134].
Research continues to evaluate ω-3 PUFAs and their downstream mediators as potential pharmaconutrient targets in PARDS. Rationale behind ω-3 PUFAs is that they would directly compete with ω-6 PUFAs, and act to decrease the synthesis of proinflammatory eicosanoids, increase production of anti-inflammatory lipid mediators such as resolvins and protectins, decrease chemotaxis, decrease Reactive Oxygen Species (ROS) and proinflammatory cytokines, and decrease leukocyte binding and activation through decreased expression of adhesion molecules [138]. It is not clear if improving these intermediate biochemical targets would result in improved clinical outcomes, and this would possibly depend on appropriate identification of PARDS subphenotypes that were more proinflammatory and therefore more likely to benefit from an anti-inflammatory treatment strategy.
Multiple studies identify an association between decreased vitamin D levels and increased risk of ARDS, although the mechanistic underpinnings of this association are not currently understood [139–141]. Vitamin D deficiency is associated with impaired pulmonary function and increased incidence of viral and bacterial infections, relevant to ARDS [142–144]. Vitamin D deficiency status may prove to be an important element of a PARDS subphenotype that predicts treatment responses. Vitamin D is known to modulate macrophage, lymphocyte, and epithelial cell functions and is therefore a rational target for further study in ARDS pathophysiology [140, 145]. It unknown if treatment with vitamin D supplementation has any role in PARDS management. Multiple nutrient deficiencies are known to occur during critical illness, but it is unclear if these truly reflect deficiency states, or reflect adaptation or redistribution as a response to critical illness. Further clinical trials are needed to assess the individual contributions of specific micronutrient replacement and nutritional modulation of the immune system to understand if there is a role for these therapies in PARDS.
Summary
The balanced management of nutritional status, fluid overload, and AKI presents unique challenges when caring for children with PARDS. Careful multidisciplinary team-based care is necessary to prescribe guideline-recommended minimum macronutrient needs to preserve lean body mass and respiratory muscle function, to avoid fluid overload, and coordinate care for AKI. As we improve our understanding of patient phenotypes and crosstalk among the lung, kidney, and gastrointestinal tract, highly personalized nutritional and fluid management strategies will likely emerge based on patient severity of illness, premorbid nutritional risk, the inflammatory phenotype in ARDS, measured energy expenditure, active monitoring of lean body mass, and the composition of patient intestinal microbiome. A select group of high-risk patients are likely to especially benefit from a tightly titrated nutritional, fluid, and kidney resuscitation plan, which may include early mechanical fluid removal. Long-stay patients; patients with PARDS, sepsis, or burns; and children with pre-existing severe malnutrition are most likely to benefit from a personalized nutrition and fluid approach, which takes into consideration the interrelatedness of these organ systems.

Full access? Get Clinical Tree
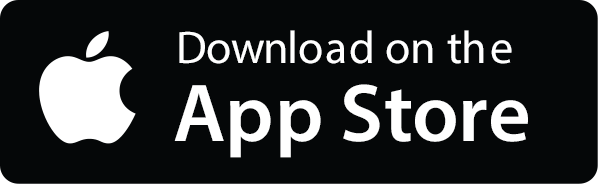
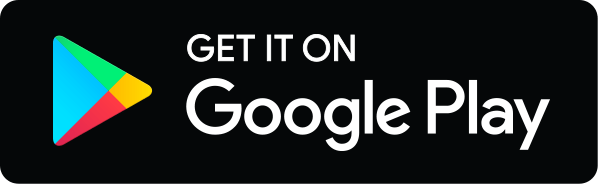