Nuclear Stress Testing
Nuclear cardiac imaging has become an integral component of the clinical practice of cardiology over the last several decades. Myocardial perfusion imaging (MPI) facilitates the detection of coronary artery disease (CAD), permits assessment of the physiologic effects of equivocal coronary artery stenoses, and assists in the identification of patients who are likely to benefit from coronary revascularization. In individuals with known or suspected CAD, MPI provides incremental prognostic information beyond that afforded by stress electrocardiography alone and can be used for individual risk stratification for future cardiac events. The fundamentals of nuclear cardiac imaging and image interpretation are discussed in Chapter 10. This chapter focuses primarily on the clinical utility of MPI for patients with suspected or established CAD and how the information derived from these nuclear imaging studies can be used to assist in the care of the patient. The contribution of nuclear imaging to the management of patients with congestive heart failure (CHF) is also briefly discussed.
MYOCARDIAL PERFUSION IMAGING
Principles of Cardiac Nuclear Stress Testing
The usual goal of MPI is to compare the pattern of tissue perfusion in the resting state to that under stress conditions, in order to detect flow-limiting coronary artery stenoses. To understand how MPI is used for this purpose, it is helpful to recall certain aspects of cardiovascular physiology. In the basal resting state, oxygen extraction from myocardial capillary blood approaches 70% and is near maximal. Thus, there is little capacity to augment tissue oxygen delivery by increasing the myocardial extraction of oxygen from the blood when the ventricular workload increases. As a result, increases in the left ventricular workload must be accompanied by nearly proportional increases in myocardial perfusion, in order to meet the oxygen demands of the tissue. Healthy coronary vessels have flow reserves between three and six, meaning that they can increase blood flows about three to six times above rest values during periods of stress.1
In most patients with CAD, autoregulatory changes in arteriolar vascular resistance are capable of maintaining normal tissue perfusion in the resting state. The left ventricular workload is low in the resting state, and the coronary circulation is usually capable of meeting basal myocardial oxygen demands. Thus, images of rest myocardial perfusion are typically normal in patients with CAD who have not had a prior infarction or an acute ischemic event. To detect an obstructive coronary stenosis, it is frequently necessary to use maneuvers that increase tissue perfusion, in order to distinguish between vessels with and without an impaired coronary flow reserve.
Detection of Coronary Stenoses
In an artery with an atherosclerotic lesion, coronary flow reserve decreases nonlinearly as the luminal narrowing increases. The ability of the coronary vessel to increase tissue perfusion during periods of stress becomes increasingly and progressively limited as the luminal stenosis becomes more pronounced.1 During stress, perfusion in myocardium supplied by a coronary vessel with a limited flow reserve will be less than that in regions subtended by healthy arteries. Measurements of stress perfusion in affected areas (in milliliters of blood flow per minute per gram of tissue) typically will exceed resting values but remain less than the values in normal myocardium. Stenoses of 50% to 60% luminal cross- sectional area or greater are usually of sufficient magnitude to impair coronary flow reserve. However, other factors, such as the presence or absence of nonlaminar flow in the vessel, the presence or absence of collateral vessels, the presence of several stenoses in series, stenosis length, eccentricity of the lumen, absolute luminal cross-sectional area, heart rate, and the pressure gradient across the stenosis, may influence the physiologic effects of a coronary stenosis on tissue perfusion.
Images depicting myocardial perfusion during stress conditions show a reduction in the relative tracer concentration, or a stress perfusion defect, in the vascular territories supplied by the arteries with flow-limiting stenoses. The hallmark of stress-induced ischemia is a reversible perfusion defect, one that is present on stress perfusion images but absent on rest perfusion images. In studies in which measurements of coronary flow reserve made with intracoronary Doppler catheters have been compared to single photon emission computed tomography (SPECT) myocardial perfusion images, a strong correlation has been noted between stenosis flow reserves <2.0 and the presence of a reversible perfusion defect. Similarly, noninvasive measurements of myocardial perfusion reserve obtained with positron emission tomography (PET) in patients with CAD suggest that reversible SPECT defects are usually associated with perfusion reserves of 1.8 or less. In general, the more severe the luminal stenosis, the greater is the likelihood that it will be associated with a stress perfusion defect.
The anatomic extent of the stress perfusion defect and the magnitude of the reduction in the relative tracer activity within the defect provide objective information about the effects of a coronary stenosis on tissue perfusion during conditions of high oxygen demand. Commercially available computer programs can be used to compare the count data from a specific patient’s images to those of a normal sexmatched population, to assist in the identification and quantification of myocardial perfusion defects (see Chapter 11). The relative tracer activity concentration within the stress defect provides an indication of the severity of the ischemia. Defect extent, or the amount of the left ventricle that is affected by the perfusion abnormality, can be expressed as the number of myocardial segments with an abnormal tracer concentration, or as the proportion (percent) of all myocardial voxels that have an abnormal tracer concentration. A proximal stenosis in a major epicardial artery will generally produce a stress defect that is larger and more readily detected than one resulting from a stenosis in a distal vessel or a smaller branch artery. The anatomic location of the perfusion defect can be used to infer which of the three major coronary vessels is (are) diseased (see Chapter 11). It may also be possible to deduce whether the stenosis is proximal, mid, or distal, based on the location and anatomic extent of the defect on the stress perfusion images.
Assessment of Relative versus Absolute Myocardial Perfusion
The SPECT or PET myocardial perfusion images used in routine clinical practice for CAD detection depict relative tissue perfusion. It is assumed that at least one area of the visualized myocardium is supplied by a vessel with a normal or near-normal coronary flow reserve. However, in some patients with multivessel CAD, there may be “balanced ischemia,” a situation in which the coronary flow reserve of each of the three major coronary arteries is equally or nearly equally impaired. In balanced ischemia, the pattern of perfusion on the stress images appears relatively homogenous. A regional perfusion defect is not identified because me stress defect involves essentially all of the visualized left ventricular myocardium. This has stimulated additional clinical interest in the use of measurements of absolute myocardial blood perfusion (in milliliters of blood flow per minute per gram of tissue) and perfusion reserves from dynamic PET perfusion images. Computer programs used to calculate PET measurements of absolute myocardial perfusion and perfusion reserve are now “user friendly” and are commercially available for routine clinical use. If a patient’s perfusion reserve measurements are abnormally low, this may result either from balanced ischemia from multivessel stenosis or from global microvascular dysfunction. The prevalence of balanced ischemia in CAD patients due to multivessel stenosis is not well defined and is likely to depend on each nuclear laboratory’s specific referral pattern. In large cardiac referral centers, the prevalence of balanced ischemia is probably <5% of patients referred for nuclear stress testing, and it may be smaller in an office-based practice. Other observations from the stress test itself and either SPECT or PET perfusion imaging can alert the nuclear cardiologist to the possibility of balanced ischemia. These include the onset of anginal symptoms with stress, a significant drop in systolic blood pressure with exercise, electrocardiographic ST-segment changes in response to stress, and acute ventricular dilatation and/or new systolic dysfunction on the gated stress perfusion images. When incorporating the results of a SPECT or PET perfusion imaging study into the management of the patient, the possibility of balanced ischemia should be considered—especially if there are other clinical observations that suggest this possibility—and further diagnostic testing should be pursued accordingly.
Resting Perfusion Defects
Perfusion defects on images acquired in the resting state may arise in several different situations. When a coronary stenosis is very severe (>90% to 95% area stenosis) or there is an unstable lesion with intermittent dynamic obstruction of the lumen, a defect can sometimes be identified on resting perfusion images. In this situation, the diseased vessel may be incapable of maintaining perfusion commensurate with the tissue’s oxygen demands. A second set of perfusion images acquired at a later time may show redistribution, or “fill in” of the resting perfusion defect, as the tissue tracer concentration in this area equilibrates with that in adjacent normal myocardium. Sometimes, a supplemental dose of the perfusion tracer may be given prior to obtaining the late set of images, to assist in the “fill in” of the defect. The clinical implication of the filling in of a resting perfusion defect on redistribution or reinjection perfusion images is that the tissue is viable, and supplied by a vessel with a severe coronary stenosis.
A resting perfusion defect that persists on redistribution or reinjection images sometimes indicates a myocardial scar. In a scar, there is replacement of cardiac myocytes by relatively avascular fibrous tissue. Residual tissue perfusion in the segment with the scar is lower than in normal myocardium, resulting in a reduction in the relative tracer concentration on both the resting and redistribution/reinjection perfusion images. Because of the limited spatial resolution of current gamma cameras and PET tomographs, reductions in perfusion due to a nontransmural scar will be averaged over the minimum resolvable volume of the instrument (the partial volume effect). Thus, it is not possible to attribute an observed reduction in myocardial tracer activity to a scar specifically in the subendocardial, midmyocardial, or epicardial portion of the ventricular wall, as counts will be averaged over the entire thickness of the tissue. In studies of subjects with clinical myocardial infarction (MI), the extent and severity of persistent resting perfusion defects have generally paralleled the loss of viable myocytes, as measured by the size of the leak of cardiac enzymes or by the amount of tissue fibrosis on ventricular specimens.2
If a resting perfusion defect persists on redistribution or reinjection images, an alternative diagnostic possibility is myocardial hibernation, a state in which there is a sustained downregulation of tissue perfusion, metabolism, and function. Although the mechanism by which human myocardium enters into a state of “hibernation” is not well defined, accumulating evidence suggests that multiple repetitive episodes of ischemia (repetitive stunning) may eventually result in myocardial hibernation.3 Histopathologic studies of hibernating myocardium have identified structural and ultrastructural alterations in the tissue. These include a loss of myofibrillar protein, myocyte hypertrophy, accumulation of glycogen within the cytosol of the cardiac myocyte, and alterations in myocyte mitochondrial size and appearance. Modest increases in tissue collagen content have also been reported. As the name suggests, the clinical implication of hibernating myocardium is that the dysfunctional tissue is viable, and that it can be “awakened” by restoration of blood flow and benefit functionally by coronary revascularization.
In both hibernating tissue and myocardial scar, there is a persistent reduction in tissue perfusion. Because there is little or no capacity to increase tissue perfusion with stress in either situation, reductions in relative tracer concentration on resting perfusion images will also be present on stress perfusion images, and therefore appear as a fixed perfusion defect. Myocardial scar and hibernating tissue both exhibit systolic dysfunction, and may be indistinguishable from each other on conventional gated myocardial perfusion scintigraphy. Additional imaging studies are frequently needed in order to distinguish between hibernating (viable) tissue and myocardial scar. Low-dose dobutamine echocardiography, contrast magnetic resonance imaging, and glucose metabolic imaging with PET (below) are some of the methods that have been used to distinguish myocardial hibernation from scar.4
Stress Options for Myocardial Perfusion Imaging
Exercise is generally the preferred stress modality because it permits the simultaneous assessment of other parameters oi clinical interest including patient symptoms, functional capacity, vital signs, and the rate–pressure product as an indirect index of myocardial oxygen consumption. Graded exercise is most commonly performed on a treadmill, using one of several standard stress protocols (see Chapter 23). In order to optimize the examination for the detection of coronary stenoses, it is important to increase the ventricular workload high enough to elicit a significant increase in myocardial perfusion. In clinical practice, the usual goal is for the patient to achieve at least 85% of the maximum predicted heart rate (MPHR) for age. The radioactive perfusion tracer is administered intravenously about 1 to 2 minutes prior to the end of stress, to allow a long enough period of time for myocardial uptake of the imaging agent prior to the termination of exercise. SPECT is the most common imaging modality employed if a patient is undergoing exercise stress MPI, as the technetium and thallium tracers have a half-life long enough to be compatible with exercise testing protocols, as opposed to the 75-second half-life of rubidium-82, the tracer most commonly used in PET cardiac imaging. However, if the testing facility has an on-site cyclotron, nitrogen-13 labeled ammonia (half-life = 10 minutes) can be used as a PET perfusion tracer with exercise stress MPI.
In some patients, an adequate level of exercise cannot be achieved because of orthopedic limitations, peripheral vascular disease, complicating medical illnesses, or the use of medications such as beta-blockers. Stress perfusion imaging is still feasible if a pharmacologic agent can be used to increase myocardial blood flow. About 40% of the MPI tests in the United States are performed using pharmacologic stress.5 Two most commonly used pharmacologic stress agents, adenosine and dipyridamole, are potent coronary vasodilators. Another coronary vasodilator agent more recently approved for clinical use in radionuclide MPI is regadenoson, a selective A2A receptor agonist.6 These agents increase myocardial perfusion by directly dilating the coronary vasculature, thereby “uncoupling” myocardial perfusion from ventricular work. The fourth agent used for MPI is a synthetic catecholamine, dobutamine, which increases tissue perfusion primarily by increasing tissue oxygen demand through its positive inotropic and chronotropic effects. The pharmacologic agents used for stress perfusion imaging are summarized in Table 13.1.
TABLE
13.1 Agents for Pharmacologic Stress Testing
Adenosine is a small molecule that is produced by vascular smooth muscle and endothelial cells. Adenosine can also be generated by the extracellular dephosphorylation of adenosine triphosphate (ATP) and adenosine diphosphate (ADP). Free adenosine within the vascular space can reenter endothelial, vascular smooth muscle, or red blood cells by facilitated transport, or it can bind to specific receptors on the cell membrane. Adenosine induces coronary vasodilatation when it binds to A2A receptors on the surface of the cell. (Adenosine binds to the A1, A2B, and A3 receptors as well, explaining its accompanying effects of AV nodal blockade and bronchoconstriction.) Binding to the A2A receptor causes an increase in intracellular cyclic AMP (cAMP) concentration via a coupled G-protein system that in turn results in coronary artery dilatation. Adenosine has a very short half-life (2 to 10 seconds), because it is rapidly cleared from the vascular space by uptake into endothelial and red blood cells. In normal coronary arteries, adenosine leads to increases in blood flows that are generally three to six times those in the resting state. Adenosine is usually administered as a continuous intravenous infusion at a rate of 140 µg/kg/min over a period of 6 minutes. The radioactive perfusion radiotracer is injected at 3 minutes, midway through the adenosine infusion. Some investigators have indicated that a 4-minute period of infusion of adenosine (with tracer injection at 2 minutes) is as efficacious for the detection of CAD as the 6-minute infusion protocol.
Dipyridamole blocks the cellular reuptake of adenosine. This causes more of the endogenous adenosine within the vascular space to bind to the A2A receptors on the cell surface. Greater receptor binding by adenosine, in turn, promotes coronary vasodilatation. Dipyridamole has a significantly longer half-life than adenosine, inducing coronary vasodilatation that may persist for as long as 30 minutes following its administration. Dipyridamole is given by continuous intravenous infusion over 4 minutes, at a rate of 0.142 mg/kg/min. The radioactive perfusion tracer is injected about 4 minutes after the end of the dipyridamole infusion, to allow achievement of maximal myocardial hyperemia.
Regadenoson is a selective A2A receptor agonist that was approved by the FDA for clinical use in MPI in 2008. Its coronary hyperemic effects have an onset within 30 seconds and usually last for 2 to 5 minutes. Regadenoson is administered intravenously as a single bolus dose of 0.4 mg, followed by a 5 mL saline flush. Two randomized double-blind multicenter trials—ADVANCE-MPI 1 and 2—demonstrated the safety of this agent in 1,871 patients, as well as an efficacy similar to adenosine for the detection of reversible perfusion defects on SPECT imaging.6,7 Regadenoson has not been compared in a clinical trial with adenosine or dipyridamole as a stress agent for cardiac PET imaging.
The side effects of adenosine and dipyridamole are similar and include flushing, chest pain, dyspnea, headache, nausea, hypotension, bronchospasm, and AV block. Of note, regadenoson appears to trigger fewer adverse events and has a favorable side-effect profile as compared to adenosine—a function of its selective agonism of the A2A receptor. Side effects, especially AV block, are more commonly seen with adenosine but tend to be short-lived with this agent—dissipating within seconds of stopping the infusion. With regadenoson and dipyridamole, any side effects experienced tend to persist longer, and treatment with aminophylline (50 to 100 mg IV), a nonselective competitive antagonist, may be required. Clinical studies suggest that the side effects of all three vasodilator agents may be attenuated if the patient is capable of performing exercise in conjunction with the pharmacologic stress. Image quality may also benefit from the performance of adjunctive exercise. Contraindications to the administration of the vasodilator agents include asthma or a history of bronchospastic pulmonary disease, hypotension, unstable angina or acute MI within 2 days, high-degree AV block without a pacemaker, uncontrolled arrhythmias, and critical aortic or mitral valve stenosis. Unlike stress with exercise or IV dobutamine (below), the adequacy of the myocardial hyperemia induced by IV adenosine or dipyridamole cannot be inferred from the changes in heart rate or systemic blood pressure induced by the administration of these agents.
For adenosine, regadenoson, and dipyridamole stress, it is important that the patient refrain from the use of drugs such as aminophylline and theophylline prior to the test, as these medications are competitive antagonists of the adenosine membrane receptor.8 These medications effectively blunt the hyperemia induced by these agents and may cause a falsely negative imaging study. Caffeine and caffeine-like substances such as theobromine, whose effects are similar to those of aminophylline, should also be avoided prior to pharmacologic stress testing with vasodilators. Current joint guidelines issued by the American Society of Nuclear Cardiology (ASNC) recommend that patients refrain from the use of caffeine for at least 12 hours prior to vasodilator stress testing.9
Dobutamine is a short-lived (half-life of about 2 minutes) β1-adrenergic receptor agonist that is widely used for stress echocardiography. Unlike stress echocardiography, when dobutamine is used as the stress for nuclear perfusion imaging it is not possible to monitor ventricular function “online.” Dobutamine stress should therefore be used with caution for nuclear imaging in patients with reduced left ventricular ejection fraction (LVEF), as new regional contractile abnormalities incited by ischemia could precipitate further deterioration in ventricular function. Dobutamine can be used in patients with bronchospastic pulmonary disease, in whom there is a relative contraindication to the use of the vasodilator stress agents described above. Dobutamine increases cardiac contractility and heart rate and is contraindicated in patients with recent MI, uncontrolled hypertension, or significant cardiac arrhythmias.
Dobutamine is administered as a continuous intravenous infusion, typically starting at 5 to 10 µg/kg/min for 3 minutes and then increasing by 10 µg/kg/min every 3 minutes to a maximum dose of 40 µg/kg/min. If the patient does not achieve 85% of his or her age-related maximal predicted heart rate, he or she can be instructed to perform handgrip exercise and/or be given up to 1 mg of atropine IV to increase the heart rate. The perfusion tracer is administered intravenously 1 to 2 minutes before the end of the dobutamine infusion, to allow enough time for myocardial uptake of the imaging agent. Side effects of dobutamine include palpitations, chest pain, hypertension, hypotension, atrial fibrillation, and ventricular tachycardia. The side effects usually respond to stopping the infusion, or to the intravenous administration of a beta-blocker.
Indications for Myocardial Perfusion Imaging
The appropriate indications for MPI, as listed by the American College of Cardiology (ACC)/American Heart Association (AHA)/ASNC guidelines,10 are summarized in Table 13.2.
TABLE
13.2 Appropriate Indications for MPI
Acute Chest Pain Syndromes
Prompt identification of individuals with acute coronary syndromes (ACSs) provides the best opportunity to salvage viable myocardial tissue and save lives. In some who present to the Emergency Department with chest pain, elevated cardiac enzyme levels and an abnormal electrocardiogram (ECG) provide definitive evidence of an acute MI, and there is no need for imaging to establish the diagnosis. On the other hand, in those with chest pain that is clearly noncardiac in origin, there is little utility in pursuing an aggressive (and expensive) diagnostic imaging strategy to exclude coronary disease. There are, however, about 6 million patients who present to Emergency Departments each year in the United States with chest pain of uncertain etiology, who have normal enzyme levels and a nondiagnostic ECG. It is these patients, in whom an ACS remains a diagnostic possibility, that MPI has the highest clinical benefit.11 Although the relative proportion of individuals with an ACS in this patient group is not large, the probability of an adverse outcome in those with a true coronary event is high if the diagnosis is missed. Reported mortality in Emergency Department patients with an ACS who are mistakenly sent home is as high as 5% to 6%. On the other hand, for those without an ACS who are admitted to the hospital for observation, there are substantial costs associated with the unnecessary utilization of health care services.
Rest SPECT MPI is useful for the evaluation of patients with suspected ACSs. Rest MPI can detect a regional perfusion abnormality in the absence of acute necrosis, especially if the radioactive tracer is injected during or shortly after an episode of chest pain. Reported negative predictive values of a normal rest myocardial perfusion scan in patients with suspected ACSs are as high as 99% to 100%, and up to 97% of patients who have a negative perfusion study in this setting will remain free of cardiac events over a short-term follow-up. Two randomized, prospective studies have shown that access to acute MPI has a beneficial effect on length of stay and hospital costs. It is the position of the ASNC that the evidence supports the use of acute rest MPI for the triage of selected Emergency Department patients with suspected ACSs.11 However, rest perfusion imaging is less helpful in those with a history of prior MI because it is not possible to distinguish a resting perfusion defect due to an acute ischemic event from that of a preexisting scar.
Both thallium-201 and technetium-99m–labeled tracers have been used for rest myocardial imaging in Emergency Department patients. Thallium-201 begins redistributing shortly after its uptake by the myocardium, and images obtained later than 10 to 15 minutes following tracer injection may miss a regional perfusion abnormality. The technetium-99m-labeled tracers are preferred for imaging because they are rapidly trapped in the myocardium and permit imaging of tissue perfusion at the time of tracer injection up to 4 hours later. The sensitivity of acute rest MPI is highest if the radioactive tracer is injected during chest pain, or shortly thereafter. Ideally, the tracer should be administered within 2 hours of the episode of chest pain. In those without prior infarction, identification of a perfusion defect and/or a segmental wall motion abnormality on the rest gated SPECT perfusion images will ordinarily prompt hospital admission and an aggressive work up for an ACS. By contrast, those who have normal scans can be discharged home with a low probability of sustaining an ischemic event in the immediate future.
Current imaging guidelines indicate that rest MPI is not appropriate for patients with definite acute MI.10 However, some who have imaging because of an uncertain clinical picture at presentation will subsequently rule in for acute infarction, and the perfusion images can provide useful information about the anatomic site of injury. If the rest perfusion images are repeated prior to hospital discharge, a measure of the degree of myocardial salvage afforded by treatment can be achieved by subtracting the perfusion defect size on the resting images at discharge (final infarct size) from that on the early images (region at risk).
Risk Assessment after ST-Elevation Myocardial Infarction
Patients with acute STEMI who are suitable for primary percutaneous intervention are usually referred directly for angiography and coronary revascularization. However, current practice guidelines indicate that noninvasive risk stratification is appropriate for stable, low-risk patients (ejection fractions >40%) who have not received reperfusion therapy or who have been treated with fibrinolytic agents.12 Final infarct size, the extent of inducible ischemia, and LVEF are the key elements of risk stratification for stable patients following ST-segment elevation myocardial infarction, and gated MPI is well suited for measurement of these parameters.13
Infarct size can be determined by measuring the amount of the left ventricle with a resting perfusion defect prior to hospital discharge. Clinical studies of patients with acute infarction suggest that resting perfusion defect size is a variable that contributes independently to cardiac risk. Patients with only small fixed perfusion defects generally have a good prognosis, whereas those with resting perfusion defects involving 20% or more of the left ventricle are at higher risk for cardiac events over the ensuing 24 months. The extent of inducible ischemia can be derived from the images by careful subtraction of the size of the rest perfusion defect from that of the stress defect. Inducible ischemia, whether in the clinical infarct zone or in other vascular territories, also contributes to cardiac risk. Patient risk increases as the percent of the left ventricle with stress-induced ischemia increases, with involvement of 10% or more of the ventricle by a reversible perfusion defect placing the patient into a highrisk group. Measurements of LVEF and ventricular volumes can also readily be obtained from the gated perfusion images. In general, as the LVEF declines to <40% there is a progressive and nonlinear increase in the risk of cardiac events. Other scintigraphic observations that have been associated with increased clinical risk in the post-MI patient include transient ischemic dilatation (TID) of the left ventricle on the stress images, and increased pulmonary tracer uptake (especially with thallium scintigraphy) on the stress images.
Stress perfusion imaging with adenosine or dipyridamole can safely be performed as early as 2 days following an acute infarction,14 while present guidelines suggest that submaximal exercise stress testing not be performed before 5 days after an acute event. MPI with vasodilator stress provides incremental prognostic information beyond that afforded by conventional clinical and stress electrocardiographic variables. Vasodilator stress perfusion imaging is more sensitive for the identification of ischemia than submaximal exercise stress testing and is more useful for risk stratification, probably because it is possible to safely achieve a greater degree of hyperemia without inducing frank ischemia using vasodilator stress (Fig. 13.1). Early risk stratification facilitates identification of the high-risk patient and appropriate referral for angiography.
FIGURE 13.1 Annual rates of mortality/recurrent MI in patients with initial uncomplicated MI, according to the results of either dipyridamole (DP) or submaximal exercise (EX) myocardial perfusion scintigraphy. SSS, summed stress perfusion defect score; SDS, summed difference between stress and rest segmental scores; SRS, summed rest perfusion defect score. Low SSS and SRS values were defined by scores of 0-4; intermediate (Intermed) values by scores of 5-8; high values by scores >8. Low SDS values were defined by scores of 0-2, intermediate values by 3-7, and high values by scores >7. Higher perfusion defect scores were associated with higher event rates, and dipyridamole stress imaging provided better risk stratification than submaximal exercise perfusion scintigraphy. (From Brown KA, Heller GV, Landin RS, et al. Early dipyridamole 99mTc-sestamibi single photon emission computed tomographic imaging 2 to 4 days after acute myocardial infarction predicts in-hospital and postdischarge cardiac events. Comparison with submaximal exercise imaging. Circulation. 1999;100:2060–2066, with permission.)
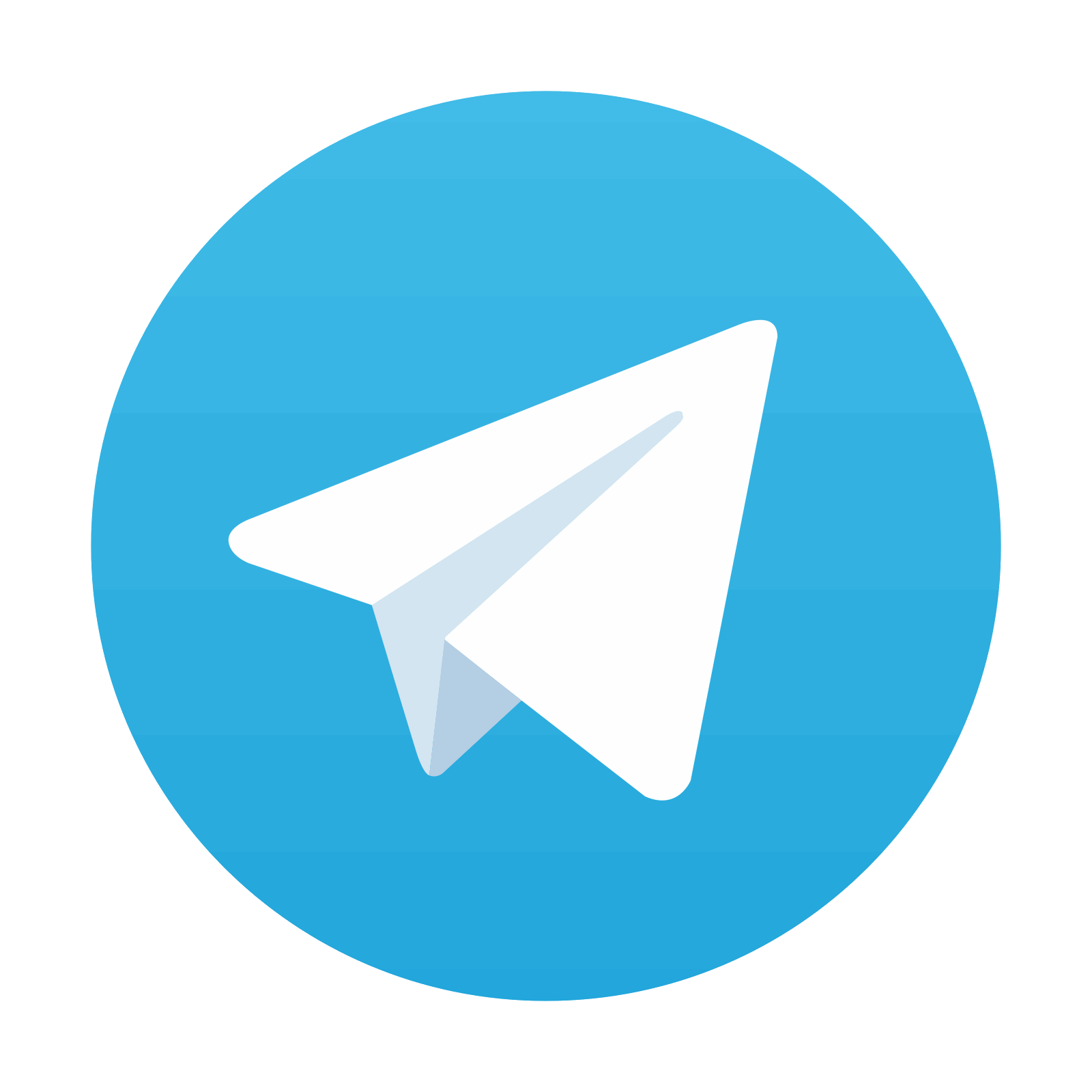