Fundamentals of Radionuclide Imaging
Radionuclide imaging techniques are widely used in the evaluation of patients with known or suspected coronary artery disease (CAD). The basic principle underlying this approach is the use of radiolabeled agents or radiopharmaceuticals that are injected intravenously and enter viable cells (e.g., myocytes, autonomic neurons) or bind to cell receptors or other targets. These techniques use radiolabeled drugs or radiopharmaceuticals, which are injected intravenously and trapped in myocardial tissue or other cell types. This radioactivity within the heart decays by emitting gamma rays. The interaction between these gamma rays and the detectors in specialized scanners—single-photon emission computed tomography (SPECT) and positron emission tomography (PET)—creates a scintillation event or light output, which can be captured by digital recording equipment to form an image of the heart. Like computed tomography (CT) and magnetic resonance imaging (MRI), radionuclide images also generate tomographic (three-dimensional) views of the heart. Contemporary PET and SPECT scanners are frequently combined with a CT scanner (so-called hybrid PET/CT and SPECT/CT). CT is used primarily to guide patient positioning in the field of view and for correcting inhomogeneities in radiotracer distribution due to attenuation by soft tissues (so-called attenuation correction). However, the CT scanner is frequently used to obtain diagnostic data, including coronary artery calcium score and, occasionally, CT coronary angiography.
Protocols for Myocardial Perfusion and Viability Imaging
Imaging protocols are tailored to the individual patient based on clinical questions, patient’s risk, ability to exercise, and body mass index, among other factors. Electrocardiogram (ECG)-triggered gated rest and stress images are acquired after the intravenous injection of the radiopharmaceutical and used to define the extent and severity of myocardial ischemia and scar, as well as regional and global cardiac function and remodeling.
Selecting a Stress Protocol
The choice of exercise versus pharmacologic stress has well-defined guidelines depending upon the patient’s condition, clinical question, and safety considerations. Exercise stress is always preferred over pharmacologic stress in patients who can exercise adequately due to the wealth of additional information that is provided—exercise capacity, hemodynamic response (maximal heart rate, heart rate recovery and reserve, peak blood pressure), stress-induced symptoms, exercise-induced arrhythmias, and ST-segment response. This approach permits coupling of clinical response and stress myocardial perfusion findings.
In patients unable to exercise adequately, pharmacologic stress with vasodilators (adenosine, dipyridamole, or regadenoson) or direct chronotropic/inotropic stimulation with dobutamine is used ( Table 12.1 ). Pharmacologic stress is also preferred in patients with left bundle branch block (LBBB) or paced ventricular rhythm, as it reduces the frequency of false-positive tests related to mechanical dyssynchrony. Among the pharmacologic stress options, vasodilator stress is preferred primarily because it produces the greatest flow heterogeneity, thereby facilitating detection of regional perfusion defects. In patients with contraindications to vasodilator stress (e.g., asthma, AV block, etc.), dobutamine is a safe alternative. Finally, vasodilator stress is commonly used as an adjunct to exercise in patients unable to achieve a maximal exercise stress test.
Dipyridamole | Adenosine | Regadenoson | Dobutamine | |
---|---|---|---|---|
Effect on coronary blood flow | × 3–4 | × 3–5 | × 2–3 | × 2 |
Dose | 0.56 mg/kg | 140 u/kg per min | 0.4 mg | Initial 3 minutes infusion of 5–10 μg/kg per min, incremental increases (2–3- minute intervals) to 20, 30, and 40 μg/kg per min. Atropine can be used to increase heart rate if target not achieved |
Duration of action/half-life | 30–45 minutes | < 10–15 seconds | Three phases:
| ∼2 minutes |
Duration of infusion | 4 minutes | 4–6-minute infusion | ∼10 second | Depends on hemodynamic response |
Maximal hyperemia | 3–4 minutes after infusion | 84 seconds (average) | 1–4 minutes after injection | Peak infusion |
Timing of radionuclide injection | 3–5 minutes after infusion | At 3 minutes for a 6-minute infusion, at 2 minutes for a 4-minute infusion | ∼40 seconds after bolus injection | Goal of ≥ 85% age-predicted maximum heart rate |
Reversal of effect | Aminophylline; used commonly | Aminophylline; used rarely | Aminophylline; used occasionally | β-blocker (preferably esmolol), used uncommonly |
Imaging Protocols
A number of different protocols have been developed, validated, and tested for accuracy. Imaging protocols must be tailored to individual patients based on the clinical question, radiotracer used, and time constraints. For SPECT myocardial perfusion imaging (MPI) ( Table 12.2 ), technetium 99m-labeled ( 99m Tc-labeled) tracers are the most commonly used imaging agents because they are associated with the best image quality and the lowest radiation dose to the patient. After intravenous injection, myocardial uptake of 99m Tc-labeled tracers is rapid (1–2 minutes). After uptake, these tracers become trapped intracellularly in mitochondria and show minimal change over time. Although used commonly in the past for perfusion imaging, 201 thallium protocols are now rarely used because they are associated with a higher radiation dose to the patient.
201 Thallium | 99m Technetium sestamibi | 99m Technetium tetrofosmin | |
---|---|---|---|
Source | Cyclotron | Generator | Generator |
Physical half-life | 73 hours | 6 hours | 6 hours |
Clinical application | MPI and viability | MPI | MPI |
Redistribution | Yes | No | No |
Whole-body effective dose | ∼27 mSv per rest/stress study | ∼10 mSv per rest/stress study | ∼9 mSv per rest/stress study |
Length of complete study (rest/stress imaging) | ∼4 hours | ∼2–3 hours | ∼2–3 hours |
PET MPI is an alternative to SPECT and is associated with improved diagnostic accuracy and lower radiation dose to patients due to the fact that radiotracers are typically short-lived ( Table 12.3 ). The ultra-short physical half-life of some PET radiopharmaceuticals in clinical use (e.g., 82 rubidium) is the primary reason that PET imaging is generally combined with pharmacologic stress, as opposed to exercise, as pharmacologic stress allows for faster imaging of these rapidly decaying radiopharmaceuticals. However, exercise is possible for relatively longer-lived radiotracers (e.g., 13 N-ammonia). For myocardial perfusion imaging, 82 rubidium does not require an onsite medical cyclotron (it is available from a 82 strontium/ 82 rubidium generator) and, thus, is the most commonly used radiopharmaceutical. 13 N-ammonia has better flow characteristics (higher myocardial extraction) and imaging properties than 82 rubidium but it does require access to a medical cyclotron in close proximity to the PET scanner. In comparison to SPECT, PET has improved spatial and contrast resolution and it provides absolute measures of myocardial perfusion (in mL/min per g of tissue), thereby providing a quantitative measure of regional and global coronary flow reserve that is unique. Quantitative measures of myocardial blood flow and flow reserve help improve diagnostic accuracy and risk stratification.
13 N-ammonia | 82 RUBIDIUM | 18 F-fluorodeoxyglucose | 15 O-water ∗ | |
---|---|---|---|---|
Source | Cyclotron | Generator | Cyclotron | Cyclotron |
Physical half-life | 9.96 minutes | 76 seconds | 110 minutes | 2.1 minutes |
Clinical application | MPI | MPI | Myocardial viability | MPI |
Exercise stress | Yes | No | NA | No |
Myocardial blood flow measurement (mL/min per g) | Yes | Yes | NA | Yes |
Whole-body effective dose | ∼2.96 mSv per rest/stress study | ∼3.72 mSv per rest/stress study | ∼7 mSv per study | ∼2.75 mSv per rest/stress study |
Length of complete study (rest/stress imaging) | ∼70 minutes | ∼25 minutes | ∼2 hours | ∼25 minutes |
∗ 15 O-water is not FDA approved for clinical use and is only used in research studies.
For the evaluation of myocardial viability in patients with severe left ventricular (LV) dysfunction, myocardial perfusion imaging (with SPECT or PET) is usually combined with metabolic imaging (i.e., 18 F-fluorodeoxyglucose [FDG] PET). In hospital settings lacking access to PET scanning, 201 thallium SPECT imaging is a useful alternative.
Comprehensive Evaluation of Coronary Artery Disease
Detection of Coronary Artery Stenosis
The basic principle of radionuclide MPI for detecting CAD is based on the ability of a radiotracer to identify a transient regional perfusion deficit in a myocardial region subtended by a coronary artery with a flow-limiting stenosis. A reversible myocardial perfusion defect is indicative of ischemia ( Fig. 12.1A ), whereas a fixed perfusion defect generally reflects scarred myocardium from prior infarction ( Fig. 12.1B ). Generally, myocardial perfusion defects during stress develop downstream from epicardial stenosis with 50% to 70% luminal narrowing or greater and become progressively more severe with increasing degree of stenosis. It is noteworthy that coronary stenosis of intermediate severity (e.g., 50–90%) is associated with significant variability in the resulting maximal coronary blood flow, which in turns affects the presence and/or severity of regional perfusion defects. For any degree of intermediate luminal stenosis, the observed physiologic variability is multifactorial and includes (1) geometric factors of coronary lesions not accounted for by a simple measure of minimal luminal diameter or percent stenosis, including shape, eccentricity, and length, as well as entrance and exit angles, all of which are known to modulate coronary resistance; (2) development of collateral blood flow; and (3) the presence of diffuse coronary atherosclerosis and microvascular dysfunction (combination of endothelial and smooth muscle cell dysfunction in resistive vessels, and microvascular rarefaction), all consistent findings in autopsy and intravascular ultrasound studies of patients with CAD. All these factors account for the frequent disagreements between angiographically defined CAD and its associated physiologic severity.

Quantification of Myocardial Ischemia
Regional myocardial perfusion is usually assessed by semi-quantitative visual analysis of the rest and stress images. The segmental scores are then summed into global scores that reflect the total burden of ischemia and scar in the left ventricle ( Fig. 12.2 ). Objective quantitative image analysis is a helpful tool for a more accurate and reproducible estimation of total defect size and severity and is generally used in combination with the semi-quantitative visual analysis. The semi-quantitative (visual) and quantitative scores of ischemia and scar are linearly related to the risk of adverse cardiovascular (CV) events and are useful in guiding patient management, especially the need for revascularization, and for assessing response to medical therapy. The presence of transient LV dilatation during stress imaging (so-called transient ischemic dilatation or TID) is an ancillary marker of risk that reflects extensive subendocardial ischemia and often accompanies radionuclide MPI studies with extensive and severe perfusion abnormalities ( Fig. 12.3A ). It is often an important finding, particularly when it occurs in patients with no or only mild perfusion abnormalities, suggesting the presence of more extensive balanced subendocardial ischemia. The presence of this abnormality has often been shown to be a harbinger of increased risk. Similarly, the presence of transient pulmonary radiotracer retention and right ventricular uptake during stress along with a drop in left ventricular ejection fraction (LVEF) post-stress (a sign of post-ischemic stunning) are also markers of multivessel LV ischemia ( Figs.12.3B and C ; ).



Quantification of Myocardial Blood Flow and Coronary Flow Reserve
Myocardial blood flow (in mL/min per g of myocardium) and coronary flow reserve (CFR; defined as the ratio between peak stress and rest myocardial blood flow) are important physiologic parameters that can be measured by routine post-processing of myocardial perfusion PET images. These absolute measurements of tissue perfusion are accurate and reproducible. Pathophysiologically, CFR estimates provide a measure of the integrated effects of epicardial coronary stenoses, diffuse atherosclerosis and vessel remodeling, and microvascular dysfunction on myocardial perfusion, and, as such, the value obtained is a more sensitive measure of myocardial ischemia. In the setting of increased oxygen demand, a reduced CFR can upset the supply–demand relationship and lead to myocardial ischemia, subclinical LV dysfunction (diastolic and systolic), symptoms, and death. As discussed hereafter, these measurements of CFR have important diagnostic and prognostic implications in the evaluation and management of patients with known or suspected CAD ( Fig. 12.4 ).

Assessment of Myocardial Viability
Myocardial perfusion and metabolic imaging are commonly used to evaluate the patient with ischemic LV dysfunction, especially when the question of revascularization is being considered. Radionuclide imaging provides important quantitative information, including (1) myocardial infarct size; (2) extent of stunning and hibernating myocardium; (3) magnitude of inducible myocardial ischemia; and (4) LV function and volumes (see Fig. 12.3 and Fig. 12.5 ).

Both 201 thallium and, especially, 99m Tc agents provide accurate and reproducible measurements of regional and global myocardial infarct size. The use of metabolic imaging with PET has been extensively validated and is commonly used for assessing myocardial viability. 18 FDG is used to assess regional myocardial glucose utilization (an index of tissue viability) and is compared with perfusion images to define metabolic abnormalities associated with infarction and hibernation. Reduced perfusion and increased FDG uptake at rest (so-called perfusion–FDG mismatch) identifies areas of viable but hibernating myocardium, whereas regions showing both reduced perfusion and FDG uptake at rest (so-called perfusion–FDG match) are consistent with myocardial scar ( Fig. 12.6 ). These metabolic patterns have important implications for selecting patients for revascularization.

Myocardial Neuronal Function
The use of imaging probes designed to evaluate presynaptic and postsynaptic targets of the cardiac autonomic nervous system allows quantification of autonomic function and offers insights into the pathophysiology of a variety of cardiovascular disorders, including CAD. For example, data suggest that quantitative imaging of the cardiac sympathetic nervous system may help identify patients with ischemic heart failure at risk for sudden cardiac death. In experimental models of myocardial infarction (MI), the presence of functional sympathetic denervation within areas of viable myocardium identifies sites at higher risk of ventricular tachycardia inducibility. There are emerging clinical trial data suggesting that this approach may provide a useful method for identification of patients at highest risk for sudden cardiac death.
Quantification of Left Ventricular Function and Volumes
The acquisition of ECG-gated myocardial perfusion images allows quantification of regional and global systolic function and LV volumes. ECG-gated images are typically collected at rest and post-stress (SPECT) or rest and during stress (PET). Rest LVEF measurements are helpful to define the patient’s risk after MI. A drop in LVEF post- or during stress testing can be helpful to identify high-risk patients with multivessel CAD (see Fig. 12.3B ).
Patient-Centered Applications of Radionuclide Imaging in Coronary Artery Disease
To understand the efficacy and application of radionuclide MPI, it is important to first have a construct capturing its conceptual underpinnings. The use of noninvasive testing can be defined in the context of what is the underlying question to be addressed by the test. In general, this can be either defining whether obstructive CAD is present (anatomy-based endpoint) or what is the patient’s downstream risk of adverse events (risk-based endpoint). The selection of patients for testing, the metrics and potential endpoints for assessing efficacy, and the post-test treatment strategies are determined by which of these are chosen.
Appropriateness of MPI for Diagnosis of Angiographic CAD
Historically, the selection of patients for testing has been informed by determination of the patient’s pretest likelihood of CAD. This estimate can be based on a variety of nomograms and models. For purposes of simplicity, rather than considering the entirety of the demographic, clinical, and historical information available, the estimates of pretest likelihood of CAD are based on a simplification of the Diamond and Forrester approach incorporating three data elements: patient age, sex, and symptoms. On the basis of these three elements, patients are classified as having low (< 10%), intermediate (10–90%), and high (> 90%) pretest likelihood of CAD. More accurate estimates of pretest likelihood based on more robust approaches would probably enhance the value of this assessment, and a number of other validated algorithms are available, albeit derived and validated in older studies.
Given this estimate, a Bayesian approach, as first put forward by Diamond, is commonly used to identify the potential gain in diagnostic information from testing. This process considers the patient’s pretest likelihood of CAD, the diagnostic accuracy of the test (defined by sensitivity and specificity), and the result of testing to yield a post-test likelihood of CAD. Although the greatest certainty with respect to the post-test likelihood of CAD occurs at the extremes of the spectrum (e.g., a negative test in a low pretest likelihood of CAD or a positive test in a high pretest likelihood of CAD patient), the greatest gain in information (magnitude of difference between pretest and post-test likelihood of CAD) occurs in the setting of intermediate pretest likelihood of CAD. For example, a patient with a pretest likelihood of 50% (intermediate) undergoing a test with a sensitivity and specificity of 90% will have a post-test likelihood of 90% (high) after an abnormal test result but a likelihood of 10% (low) after a normal test result. Thus, patients with a low pretest likelihood of CAD should not undergo radionuclide MPI testing because a positive test will increase their likelihood to intermediate and is more likely to be a false-positive than a true-positive result. Similarly, there is an unclear gain in diagnostic information from testing a high pretest likelihood of CAD patient. However, radionuclide MPI in high likelihood patients is sometimes performed to provide risk stratification and/or identification of the culprit vessel to guide revascularization. Hence, for the identification of anatomic endpoints, radionuclide MPI should be limited to those patients at intermediate pretest likelihood of CAD (based on demographic, clinical, historical, and exercise tolerance testing [ETT] data).
There has been a shift to appropriate use criteria (AUC) to define optimal patient selection for noninvasive testing. The recommendations from the 2013 multimodality AUC for the detection and risk assessment of stable ischemic heart disease indicate that in symptomatic patients with intermediate or high pretest likelihood of CAD, irrespective of resting ECG and ability to exercise, it is appropriate to use radionuclide MPI ( Fig. 12.7 ). Additionally, radionuclide MPI is also appropriate in symptomatic patients with uninterpretable resting ECG or who cannot exercise. Finally, in symptomatic patients able to exercise and with interpretable resting ECG, MPI is reported as rarely appropriate.

In the asymptomatic patient, no symptoms or ischemic equivalent ( Fig. 12.8 ), the AUC document considers patients in the context of global risk. Generally, this is defined as low (< 10% 10-year risk), intermediate (10–20% 10-year risk), or high (> 20% 10-year risk). The thresholds for the former two categories are lowered in younger patients, and CAD equivalents can also define patients at high risk. In the setting of high risk (rest ECG interpretable or not, able to exercise or not) in the absence of symptoms, radionuclide MPI was only considered as “may be appropriate.” Patients at intermediate risk with uninterpretable rest ECG or unable to exercise are given a similar “may be” rating. In patients at low risk or patients at intermediate risk who have interpretable ECG and can exercise, MPI is considered “rarely appropriate.” In patients with known CAD, radionuclide MPI is generally considered appropriate for the evaluation of new symptoms suspected of ischemia regardless of prior revascularization. Radionuclide MPI is considered rarely appropriate in patients with stable CAD without new symptoms.

Accuracy of Radionuclide MPI for the Identification of Angiographic CAD
The accuracy of diagnostic testing for the identification of obstructive CAD is expressed in terms of sensitivity, specificity, and positive and negative predictive value. The threshold of abnormality on invasive angiographic results, the accepted gold standard, is either 50% or 70% in one or more coronary arteries. Left main (LM) coronary disease is usually defined on the basis of a 50% stenosis. An extensive literature exists defining the diagnostic accuracy of radionuclide MPI. This encompasses both SPECT and PET imaging, using different techniques, and for patients representing various subgroups.
A large meta-analysis examined the diagnostic accuracy of stress SPECT MPI for detecting obstructive CAD as defined by invasive coronary angiography. This analysis examined 86 studies (10,870 patients) published between 2002 and 2009, with pooled accuracy estimates for various SPECT MPI subgroups. “Traditional” SPECT MPI (63 studies; performed without ECG gating or correction of soft tissue attenuation) had a sensitivity and specificity of 87% and 70%, respectively. The addition of ECG gating (19 studies) and attenuation correction (12 studies) increased specificity to 78% and 81%, respectively. Comparisons between 99m Tc and 201 Tl, exercise versus pharmacologic stress, qualitative versus quantitative interpretation, 50% versus 70% thresholds, or history of prior MI were all nonsignificant.
Compared with SPECT MPI, meta-analyses and a prospective European multicenter study (Evaluation of Integrated CAD Imaging in Ischemic Heart Disease—EVINCI) suggest that PET MPI has higher sensitivity and overall accuracy for detecting obstructive angiographic stenosis. Furthermore, a 2015 meta-analysis using fractional flow reserve (FFR) rather than invasive angiography as a gold standard demonstrated higher sensitivity, specificity, and negative and positive predictive value for PET over SPECT MPI. In this analysis, PET’s performance at excluding abnormal FFR was comparable to cardiac MRI and CT and superior to both SPECT and echocardiography ( Fig. 12.9 ). As discussed previously, one additional advantage of PET over SPECT is that it allows routine quantification of myocardial blood flow and coronary flow reserve. These quantitative measures of myocardial perfusion improve the sensitivity and negative predictive value of PET for ruling out high-risk angiographic CAD ( Fig. 12.10 ). Although older studies reported superior accuracies for SPECT in men compared to women, the use of PET or SPECT with newer technology has largely eliminated this difference. In general, the challenges to accurate SPECT MPI detection of ischemia occur with smaller hearts and the presence of increased tissue for photons to transverse (e.g., breast tissue in women, obese patients). In symptomatic patients without documentation of angiographic stenosis, PET MPI is helpful for identifying the presence and quantifying the severity of diffuse microvascular disease.


Referral Bias and the Estimation of MPI Accuracy
The reported accuracies of noninvasive testing modalities are limited by the referral biases intrinsic to the design of most studies in this area, including the population from which patients were drawn, test selection, intersite variability in referral patterns, and especially partial verification bias. The latter refers to selective referral to the gold standard (catheterization) based on the results of the test being studied. Hence, very few patients with normal noninvasive tests will be referred to catheterization, whereas many more with abnormal tests will be referred for coronary angiography. This results in relatively fewer true- or false-negatives and more true- or false-positives, yielding a slight increase in sensitivity and a marked reduction in specificity ( Fig. 12.11 ).

Risk Stratification of CAD
Since the 1990s, risk-based approaches to testing have developed into the predominant perspective in the use of noninvasive testing. The understanding of this approach has evolved over time. First, there has been a particular focus on incrementalism in the assessment of the value of testing: that is, what is the test’s ability to add clinically meaningful information after the consideration of other previously available information? Hence, the determination of whether radionuclide MPI has prognostic value in a specific patient cohort must consider this prognostic impact within the context of clinical, historical, and demographic information, as well as the results of other previously performed testing. With respect to the latter, the results of resting ECG, baseline LV size and function, and stress ETT have been emphasized, but the results of atherosclerosis testing (e.g., coronary artery calcification [CAC], CT angiography [CTA]) are also legitimately part of the question. The more expensive imaging modalities must demonstrate the addition of unique rather than redundant clinical data to justify their use.
Just as the application of testing for the diagnosis of obstructive CAD begins with estimates of patient pretest likelihood of CAD, the first step of a risk-based approach to testing is the estimation of patient risk. Historically, the American College of Cardiology/American Heart Association (ACC/AHA) stable angina guidelines defined risk of hard cardiac events (cardiac death or nonfatal MI), with specific thresholds of low risk as less than 1%/year risk, intermediate risk as a 1% to 3%/year risk, and high risk as a greater than 3%/year risk. Conceptually, these thresholds were based on the belief that low-risk patients would be least likely to benefit from revascularization as opposed to medical therapy alone, whereas those patients at high risk would most likely benefit from both medical therapy and revascularization. The recent multimodality AUC for the detection and risk assessment of stable ischemic heart disease refers to alternate thresholds and methodologies for specific estimates of risk in asymptomatic individuals and other approaches in symptomatic patients and those with known CAD.
Clinical Risk after a Normal Radionuclide MPI
An extensive literature exists documenting the low risk of adverse events following a normal stress radionuclide MPI. In general, when examining either large patient series or specific cohorts at relatively low or intermediate overall risk, annualized event rates after a normal SPECT or PET MPI are very low, usually less than 1% annual risk of cardiac death and often less than 1% annual risk of the combined endpoint of cardiac death or nonfatal MI. In a meta-analysis of almost 40,000 patients, a normal or low-risk rest/stress SPECT MPI was associated with an annual risk of major CV events of 0.6%. Similar results have also been reported after a normal PET MPI.
The low risk associated with normal SPECT MPI has been extended to the radiation-sparing, stress-only MPI protocols as well. All-cause mortality in 8034 patients followed for a median of 4.5 years after a normal stress-only SPECT was reported to be lower than in 8820 comparative patients undergoing stress-rest protocols. These findings have been confirmed by other authors.
Whereas the risk associated with a normal MPI is generally low, this is not necessarily the case in higher-risk cohorts (e.g., diabetes, chronic kidney impairment, elderly); that is, a normal scan is not always associated with low risk ( Table 12.4 ). This may be related in part to the presence of associated comorbidities that increase clinical risk, and that this increased hazard cannot be quantified by the results of the radionuclide MPI study. Another possibility is that notwithstanding the clinical utility of SPECT MPI, it is a somewhat insensitive test to uncover diffuse atherosclerosis and/or microvascular dysfunction associated with myocardial ischemia and increased risk of adverse events. For example, quantification of global coronary flow reserve by PET—an integrated marker of epicardial stenosis, diffuse atherosclerosis, and microvascular dysfunction—was able to identify truly low-risk individuals among patients with diabetes. Indeed, diabetics without known CAD with abnormal CFR had a cardiac mortality risk similar to nondiabetics with known CAD, whereas those with relatively preserved CFR had an annual risk of less than 1% that was comparable to subjects without diabetes or CAD ( Fig. 12.12 ). Similar findings have been shown by adding a coronary artery calcium score in patients with normal MPI. Prognostic predictions in the elderly population also highlight the need for appropriate risk thresholds in formulating a risk estimate. For example, although the absolute risk after a normal SPECT MPI in a very elderly population was far greater than the conventionally accepted threshold of 1%/year, the risk of an elderly patient with a normal MPI was still lower than that of a similarly aged individual in the general US population.
Study | Modality | Endpoint | Subgroup | Event rate after normal study | Event rate after mildly abnormal study | Event rate after moderate to severely abnormal study | FU (years) |
---|---|---|---|---|---|---|---|
Hachamovitch et al. | SPECT | CD | Age 75–84 yr | 1.0%/yr (2332) | 1.7%/yr (785) | 4.9%/yr (1201) | 2.8 ± 1.7 |
Age ≥ 85 yr | 3.3%/yr (443) | 4.0%/yr (183) | 11.1%/yr (256) | ||||
Pharmacologic stress; age ≥ 75 yr | 1.9%/yr (1526) | 2.7%/yr (609) | 7.8%/yr (907) | ||||
Exercise stress; age ≥ 75 yr | 0.7%/yr (1249) | 1.0%/yr (359) | 2.7%/yr (550) | ||||
Normal rest ECG | 0.4%/yr (783) | 0.9%/yr (169) | 1.1%/yr (110) | ||||
Abnormal rest ECG | 1.7%/yr (1992) | 2.3%/yr (799) | 6.3%/yr (1347) | ||||
Prior CAD; age ≥ 75 yr | 1.8%/yr (682) | 2.3%/yr (491) | 6.6%/yr (1009) | ||||
No prior CAD; age ≥ 75 yr | 1.2%/yr (1115) | 1.8%/yr (477) | 4.3%/yr (448) | ||||
Kang et al. | SPECT | CD | BMI < 25 | 0.8%/yr (605) | 2.2%/yr (378) | 4.0%/yr (882) | 3.2 ± 2.0 |
BMI 25–29.9 | 0.4%/yr (642) | 0.8%/yr (405) | 2.9%/yr (982) | ||||
BMI ≥ 30 | 0.4%/yr (272) | 1.3%/yr (180) | 2.2%/yr (374) | ||||
Sood et al. | SPECT | CD, NFMI, UA leading to hospitalization, or late revascularization | Low DTS in women | 1.2%/yr (995) | 1.5%/yr (55) | 5.2%/yr (12) | 2.4 ± 1.2 |
Intermediate DTS in women | 1.5%/yr (1012) | 5.3%/yr (71) | 10.8%/yr (23) | ||||
Dorbala et al. | PET | CD | Consecutive series | 0.2% (664) | 1.3% (381) | 8.3% (387) | 1.7 ± 0.7 |
All cause death | 3.5% (664) | 6.1% (381) | 16.5% (387) |
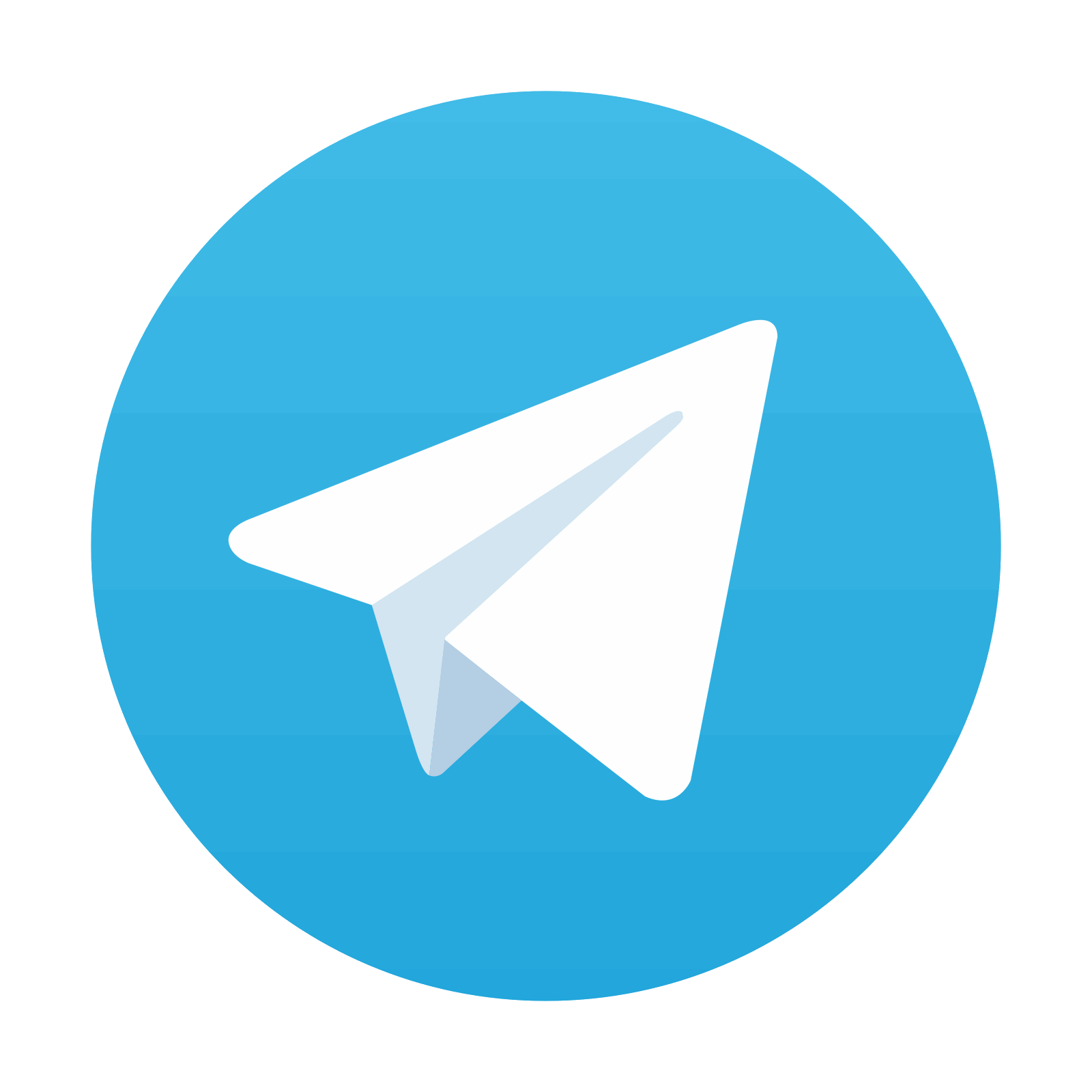
Stay updated, free articles. Join our Telegram channel

Full access? Get Clinical Tree
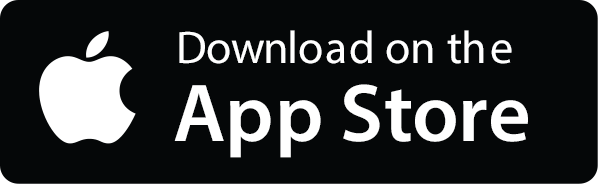
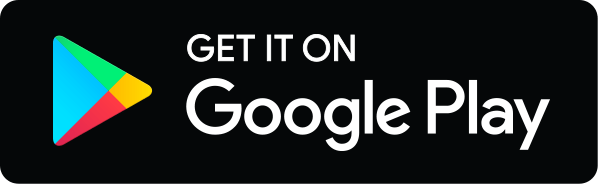
