It is estimated that 5.7 million Americans adults had heart failure (HF) in 2012 with an associated cost of $30.7 billion in that year alone.1 The incidence of HF is high, with 870,000 new cases being reported annually and projections suggest a prevalence of >8 million adults in United States by 2030.1 Mortality attributed to HF in 2011 was 58,309 in the United States, and its mention on death certificates was 284,388 (1 in 9 deaths).1
Although survival of patients with diagnosed HF has improved over time, it remains a disease with poor outcome. Approximately 50% of HF patients will be deceased 5 years after diagnosis.2
Given the growing prevalence of ischemic HF (IHF) and its high mortality rate, the optimal management strategies for IHF has been the focus of research for the last several decades. Evidence, including randomized controlled trials, have accumulated and support the notion that patients with IHF may benefit from viability imaging to guide therapy and revascularization decisions.3–9 In some, but not all studies, in patients with IHF, the presence of viability, demonstrated on noninvasive images, is a strong indicator that revascularization may improve survival.7,8,10–13 This chapter will describe the concepts of viability, nuclear imaging methods of assessing viability, supportive data and finally patients who could benefit from viability assessment.
Viable, stunning, and hibernating myocardium are important concepts that are often misunderstood. Misconceptions of these three entities sometimes can lead to incorrect clinical decision making.
Dysfunctional myocardium can be dichotomized into viable or nonviable myocardium. In the latter, the tissue is replaced by irreversible fibrosis and therefore cannot be reversed nor improved with revascularization. Conversely, viable myocardium is that with variable function, metabolism, and blood flow. In cases of dysfunctional but viable myocardium due to repeated episodes or persistent abnormal coronary flow, restoring coronary flow may result in metabolic recovery and recovery of function.3,14 The main goal of viability imaging is to define the amount of viable myocardium in order to guide decision making to therapies which improve not only LV function but also clinical outcomes.4–10
Stunned myocardium refers to a post ischemic state where there is mismatch between function and flow. Although resting coronary flow has returned to normal, myocardial function remains impaired. The duration of stunning is dependent on the duration, severity, and size of the ischemic insult.15–18 In stunned myocardium, metabolic alterations prevail over structural changes. Electron microscopy of stunned myocardium shows normal or just mildly degenerated cells.14 Observed metabolic derangement includes a decrease in calcium sensitivity of myofilaments.18 Metabolic changes can be complex and change over time. A GLUT 4 translocation to the sarcolemma and an increase in glucose uptake have been observed19; so too has a decrease in glucose uptake on metabolic imaging post-STEMI revascularization.20 If the injury persists and/or in cases of repetitive stunning, myocardial changes can progress to a hibernation state (viable) or to irreversible fibrosis/scar (nonviable).3,21,22
If repeated ischemia is prevented, stunned myocardium is expected to eventually recover. As such, imaging in the post-MI period following large MIs (STEMIs or large non-STEMIs) may be misleading in that viable myocardium may recover spontaneously without needing revascularization. In part because of this (but also because of variable metabolic derangements in the stunned myocardium and potential effects of no reflow-phenomena as well as inflammation, which could each alter tracer uptake and lead to false positive or negative findings regarding viability) performing viability imaging in the post-MI period is challenging. Thus viability imaging is often avoided within 2 to 4 weeks of large myocardial infarctions (MIs).
Hibernating myocardium is thought to occur after repeated episodes of ischemia or stunning3,23 and by definition, is viable and therefore has the capacity for functional recovery after adequate revascularization.3,4,24 Myocytes in a hibernating state lose variable amounts of contractile material (sarcomeres) without significant changes in the cell volume. The absence of volume changes is an important characteristic that helps differentiate viable myocardium from atrophic degeneration.25 Cellular volume, previously occupied by myofilaments, is replaced by glycogen (Fig. 21-1).25 This adaptive downregulation response is important to prevent a “supply-demand imbalance” during a period of limited coronary flow reserve.23 Mitochondria apparatus is preserved. Functionality and oxidative metabolism remain but may be reduced and can be measured by PET with 11C-acetate (acetate is transported into the mitochondria directly via acetyl-CoA and then enters into the tricarboxylic acid cycle to produce energy).26 Similarly, myocardial glucose metabolism is preserved (via increased glycolysis to offset reduced oxidation) and can be measured by PET with 18fluorodeoxyglucose (18FDG), a glucose analog that is transported into the myocyte and converted to FDG-6-phosphate. This preservation of glucose metabolism is key and makes it possible for cardiac metabolic imaging to distinguish between viable and nonviable myocardium.
Figure 21-1
(A) Light micrograph of myocardium showing normal cardiomyocytes with virtually no glycogen (PAS staining in red). (B) Transmission electron micrograph of normal cardiac myocyte. (C) Representative light micrograph of biopsy sample of human hibernating myocardium. Cardiac myocytes are depleted of their contractile material and filled with glycogen (PAS-positive staining). (D) Representative transmission electron micrograph of a hibernating cardiomyocyte. Myolytic cytoplasm is devoid of sarcomeres and filled with glycogen. Magnification: a and c, ×320; b, ×7100; and d, ×7500. (Reproduced with permission from Vanoverschelde JL, Wijns W, Borgers M, et al. Chronic myocardial hibernation in humans from bedside to bench. Circulation. 1997;95(7):1961–1971.)

Several modalities are available for viability assessment: cardiac PET, single-photon emission computed tomography (SPECT), dobutamine echocardiography (ECHO), dobutamine magnetic resonance imaging (MRI), delayed-enhancement MRI (DE-MRI), delayed-enhancement computed tomography (CT),4 and myocardial contrast ECHO.27,28 Metabolic and cellular targets and findings suggestive of viability for each modality are outlined in Table 21-1. The clinically available nuclear imaging methods are summarized below.
Imaging Modalities and Mechanisms for Viability Detection | ||
---|---|---|
Imaging Modality | Method Target | Indicator of Viability |
18FDG PET | Glucose metabolism | Normal perfusion/FDG = viable (not ischemic at rest) Perfusion-Metabolism (a) mismatch = hibernation (b) match = scar |
SPECT (Tl-201) | Myocardial perfusion/Na/K ATPase activity (membrane integrity) | Uptake = viable Redistribution on delayed imaging after stress, rest or reinjection = ischemia or hibernation |
SPECT (Tc-99m-based) | Myocardial perfusion/arterioles vasodilatation Mitochondrial integrity | Uptake = viable Mismatch between rest and post nitro images = hibernating |
Dobutamine echocardiography/MRI | Contractile reserve | Improvement in wall motion with low dose dobutamine |
Delayed enhancement MRI | Fibrosis tissue | Absence or small amount of scar |
Late enhancement CT | Fibrosis tissue | Absence or small amount of scar |
Myocardial contrast echocardiography | Microvascular integrity | Homogeneous contrast intensity |
Myocardium viability imaging can be performed with 201-thallium (201Tl) or 99m-technetium (99mTc) and relies on the integrity of the sarcolemma and mitochondria, respectively.29–31 Comparisons between 201Tl SPECT, 99mTc SPECT, and 18FDG PET show that scintigraphy with 99mTc-based imaging may underestimate the amount of viable myocardium,32–36 while one direct comparison study observed that 201Tl provided comparable information with 18FDG PET.37 A meta-analysis of 40 studies (1119 patients) using 201Tl SPECT showed a mean sensitivity, specificity, predictive positive value (PPV), and negative predictive value (NPV) of 87%, 54%, 67%, and 79%, respectively.4 A meta-analysis of 25 studies (721 patients) that used 99mTc SPECT to assess viability showed sensitivity, specificity, PPV, and NPV of 83%, 65%, 74%, and 76%, respectively.4 Both were not as sensitive as 18FDG PET.4 However, other studies showed similar values between 201Tl and 99mTc-based imaging.38,39 Overall, both approaches are considered reasonable means to assess myocardial viability.8
Thallium is a potassium analog and its uptake is both passive and also active via a process requiring the normal function of the sodium-potassium ATPase pump and cellular membrane integrity.40 Since membrane integrity is a requisite for cell viability, thallium-201 uptake visualized by SPECT images is indicative of myocardium viability.
Different protocols are described to assess viability with 201Tl SPECT. The American Society of Nuclear Cardiology (ASNC) guidelines41 describe a didactic format (Figs. 21-2 and 21-3). Although the rest-redistribution protocol can be performed (see Fig. 21-2), the most common protocol starts with a stress phase and injection of 2.5 to 3.5 mCi of 201Tl at peak stress (Fig. 21.3). After 10 to 15 minutes, the stress images are acquired with redistribution (rest) imaging acquired 2.5 to 4 hours later. Up to this point, a regular stress/rest 201 Tl protocol is described. When a persistent (fixed) defect is present and viability needs to be assessed, a late-redistribution imaging can be performed at 18 to 24 hours. 201Tl redistribution is a continual process that requires blood supply to the viable tissue and thus its uptake is also related to perfusion and the severity of coronary artery stenosis.42 Studies have shown that in late images (8–72 hours) the viable myocardium segments show thallium redistribution (reversible defects) while truly nonviable myocardium appears as a persistent (fixed) defects on the late perfusion images (no thallium uptake).42–45 To maximize the protocol, addition 1 to 2 mCi of 201Tl can be reinjected (Fig. 21-3).41
Subanalysis of a pooled meta-analysis compared 201Tl SPECT rest-redistribution to the 201Tl SPECT reinjection protocol and showed comparable sensitivities (87% for both protocols) but with higher specificity and PPV for 201Tl SPECT rest-redistribution (56% vs 50% and 71% vs. 58%, respectively) (p < 0.05 for both).4 On the other hand, head to head comparison between 24-hour redistribution with the reinjection protocol suggested that reinjection may provide better diagnostic information with a significantly greater ability to identify hibernating myocardium.46 When comparing the reinjection protocol to 18FDG PET, there was very good correlation, although 201Tl may have inferior sensitivity compared to glucose metabolism assessment with PET.4,37,47,48 The difference in sensitivity may be because perfusion/18FDG PET already considers perfusion. 18FDG then adds metabolic information when there is mismatch. Figure 21-4 shows an example of 201Tl SPECT rest-redistribution imaging.
99mTechnetium-sestamibi and 99mTc-tetrofosmin have lipophilic proprieties and enter cells passively. However, their retention by the mitochondria is an active process and depends on mitochondrial membrane integrity.49 99mTc-based radiotracers (sestamibi and tetrofosmin) have almost no redistribution when compared to 201Tl,50 and different approaches are suggested to increase sensitivity for viability detection.
While resting 99mTc-based perfusion imaging can be used alone to define viability, several studies have shown that the use of nitrates improves viability detection when compared to rest 99mTc-based imaging and correlates with improvement after revascularization.39,51–55 Although the exact mechanism is not well understood, it is proposed that it may be related to improvement in blood flow secondary to vasodilation improving blood supply to the hibernating myocardium and therefor tracer uptake.54 Studies have also demonstrated that abnormal contractility can lead to perfusion defects with SPECT perfusion imaging.56,57 Incremental value can be achieved by adding electrocardiogram (ECG) gating and dobutamine to 99mTc-based imaging, enabling the assessment of both perfusion and contractile reserve in a single study.58
Dual-isotope imaging with 99mTc at stress and 201Tl at rest is also possible. The 201Tl rest portion is used for viability interpretation with the addition of late redistribution and reinjection to increase the test sensitivity. However, this protocol is associated with a significantly higher radiation dose and may not be the ideal primary test when other diagnostic options are available.41
After choosing the image acquisition protocol according to patient characteristics and institutional availability, imaging interpretation should be performed carefully.
While resting 99mTc-based perfusion imaging can be used alone to define viability, 99mTc-based SPECT viability imaging interpretation can be enhanced by including both rest and post nitrate images read simultaneously.50 Persistent (fixed) defects with absence of tracer uptake noticed on both rest and post nitrate images are unlikely to recovery with revascularization, and represent the so-called nonviable myocardium (scar). Dysfunctional segments with tracer uptake similar to other segments with normal wall thickening may recover, and segments that improve tracer uptake with nitroglycerin administration are likely to recover (viable hibernating myocardium).50,59,60 For 99mTc-based SPECT, 50% to 55% of peak activity is usually defined as the cutoff to predict functional recovery and, therefore, to determine viability61 (Table 21-2). It is important to remember that 99mTc-based SPECT can also be performed to assess ischemia. In this case, images would be acquired in rest and post stress (exercise or pharmacological stress). A moderate to severe reversible defect in this case represents ischemia and may benefit from revascularization—viability imaging would not be needed. (Stress perfusion imaging is considered in a separate chapter.) In general, viability testing should be reserved for patients where there are persistent defects on stress perfusion imaging with mild or no ischemia or in patients where stress imaging may be considered to have increased risk such as severe multivessel disease or very severe LV dysfunction.
Interpretation of 99m-Technetium SPECT | ||||
---|---|---|---|---|
Rest Perfusion | Post Nitrate Perfusion | Stress | Category | Clinical Relevance |
Preserved (normal) | Preserved | Not acquired | Normal—viable | |
Preserveda 50,61 | Not acquired | Not acquired | viable | May benefit with revascularization50,61 |
Preserved | Not acquired | Reduced | Ischemia | Ischemia—may benefit from revascularizationb |
Reduced | Preserved (50–55% of peak activity)50,61 | Not acquired | Hibernating myocardium —viable | Likely to recover with adequate revascularization51–55,59,60; may be observed after post-MI revascularization112 |
Reduced | Reduced | Not acquired | Scar—nonviable | Unlikely to recover with adequate revascularization4 |
Similar approaches may be used when 201Tl SPECT imaging is performed. In the first step of the 201Tl protocol, images are acquired at stress and after rest and then, a third optional delayed image (redistribution) is acquired if a persistent defect is present on stress and rest images.50 After acquisition of the first set of images (stress/rest), images should be reviewed. If the defect is reversible, the interpretation is that myocardium is ischemic and therefore viable (and thus may benefit from revascularization). If the defect is persistent (fixed), then a redistribution image is acquired and interpreted.50 A persistent (fixed) severe defect (<50% of peak tracer uptake) present in all three images suggests a myocardium which is unlikely to recovery after revascularization (nonviable). A reversible defect on the third set of images (late redistribution) or delayed images of a rest-redistribution protocol, with tracer uptake 55% to 60% of peak activity,50,61 indicates the presence of viable hibernating myocardium which is likely to benefit from revascularization (Table 21-3).
Interpretation of 201-Thallium SPECT | ||||
---|---|---|---|---|
Stress Perfusion | Rest (Redistribution) Perfusion | Late Redistribution Perfusion (or Post-Reinjection) | Category | Clinical Relevance |
Preserved-normal | Preserved | Not acquired | Normal—viable | |
Preserveda | Preserveda | Not acquired | viable | May benefit with revascularization |
Reduced | Preserved | Not acquired | Ischemia | Ischemia—may benefit from revascularizationc |
Reduced | Reduced | Preserved (55–60% of peak activity) Increased activity from rest | Viable (Hibernating myocardium) | Likely to recover with adequate revascularization 56-59 |
Reduced | Reduced | Reduced | Scar—nonviable | Unlikely to recover with adequate revascularization4 |
The rationale for 18FDG PET use in viability assessment is based on the fundamentals of myocardial metabolism. The cardiomyocytes can use free fatty acid (FFA), glucose, lactate, pyruvate, and ketone as energy substrates, with the first two being the most important sources of energy.62–65 Fasting, adrenergic stimulation, ischemia, or insulin can shift the preferred energy substrate toward either FFA (in the case of fasting) or glucose.66–69
During fasting, FFAs are the preferred energy substrate. FFA plasma levels are increased as a consequence of low insulin levels and increased peripheral lipolysis in adipose tissue.65,69 However, during hyperglycemic states (postprandial), the higher insulin plasma level suppresses lipolysis 70 and stimulates myocardial glucose uptake, with glucose becoming the primary substrate.71 This process is primarily mediated by the glucose transporters 1 and 4 (GLUT 1 and 4). During ischemia and high insulin metabolic state, GLUT 1 and GLUT 4 are transported from intracellular storages to the plasma membrane to increase glucose uptake by the myocyte.72,73 Similarly, under adrenergic stimulation and ischemic conditions (myocardial ischemia), the FFA oxidation process decreases or may cease and glycolysis (anaerobic glycolysis) facilitates the use of glucose as the main source of myocardial energy.66,68,71 Glucose is the primary substrate in hibernating myocardium and is the basis for the utility of 18FDG PET for viability imaging.
18FDG is a glucose analog and is used clinically to assess and quantify glucose utilization. Although 18FDG is thought of as a marker of glucose metabolism, technically speaking, it is more specifically a direct measure of exogenous glucose uptake. After 18FDG is transported across cellular membranes, it is converted to FDG-6-phosphate and becomes trapped in the myocyte where it is trapped and does not continue down the metabolic pathway.74
Viability imaging protocols using PET are usually composed of two portions: rest perfusion imaging with 13-ammonia (13N) or 82-rubidium (82Rb) and metabolic imaging with 18FDG. However, 99mTc-based SPECT and 201Tl SPECT rest perfusion images can also be used to compare with metabolic 18FDG PET images in cases where PET perfusion with 13N or 82Rb are not available (preferably with attenuation correction of the SPECT MPI).75
Patient preparation is an important component of the 18FDG PET viability protocol. In a region of reduced perfusion, areas of 18FDG uptake indicate viable myocardium, while the absence of uptake indicates nonviable myocardium.50,76–79 Thus patient preparation is very important for the success of the viability study. Options include:
Fasting: Fasting is a simple approach but can lead to inferior image quality when compared to glucose loaded.80 The rationale behind this approach is that the normal myocardium will consume FFA while ischemic myocardium (viable) will preferentially use 18FDG and appear as a “hot spot.” This approach is generally not recommended as an isolated technique.50,76 However, fasting is routinely needed as a part of the preparation before administration of glucose +/– insulin used in the three current methods (Fig. 21-5).
Glucose loading: Following a fasting period of at least 6 hours (preferable 12 hours), a glucose load is administered intravenously or orally.76 Due to its simplicity, this protocol is commonly used at many centers. By increasing glucose plasma levels, insulin release is stimulated which decreases FFA plasma levels and shifts the myocytes toward glucose (and therefore 18FDG) utilization. However, 20% to 25% of the patients with CAD may have poor image quality.50 This is most commonly problematic in diabetics who have impaired insulin release or insulin resistance. In such cases, intravenous (IV) insulin may need to be administered according to a sliding scale81 (45–60 minutes after glucose loading) repeating every 15 minutes targeting a glucose serum level between 100 and 140 mg/dL.76 FDG is administered approximately 1 hour after glucose load.
Hyperinsulinemic/euglycemic clamp: The hyperinsulinemic/euglycemic clamp protocol uses the intravenous infusion of both glucose and insulin. Blood glucose levels need to be monitored and the amount of glucose/insulin is tailored to each individual patient.50,76 Although this is a more time-consuming protocol and requires an experienced team, the image quality is superior to the standard glucose loading protocol.78,82 Some centers use this method routinely in all patients; others reserve it for all patients with diabetes.
Acipimox: Acipimox is a nicotinic acid derivative that inhibits peripheral lipolysis thus reducing FFA availability. This process indirectly forces myocardial utilization of glucose as preferable substrate by reducing circulating FFAs. Acipimox administration provides comparable image quality as the hyperinsulinemic/euglycemic IV clamp77,79 although nicotinic acid itself (i.e., Niacin) does not.78,82
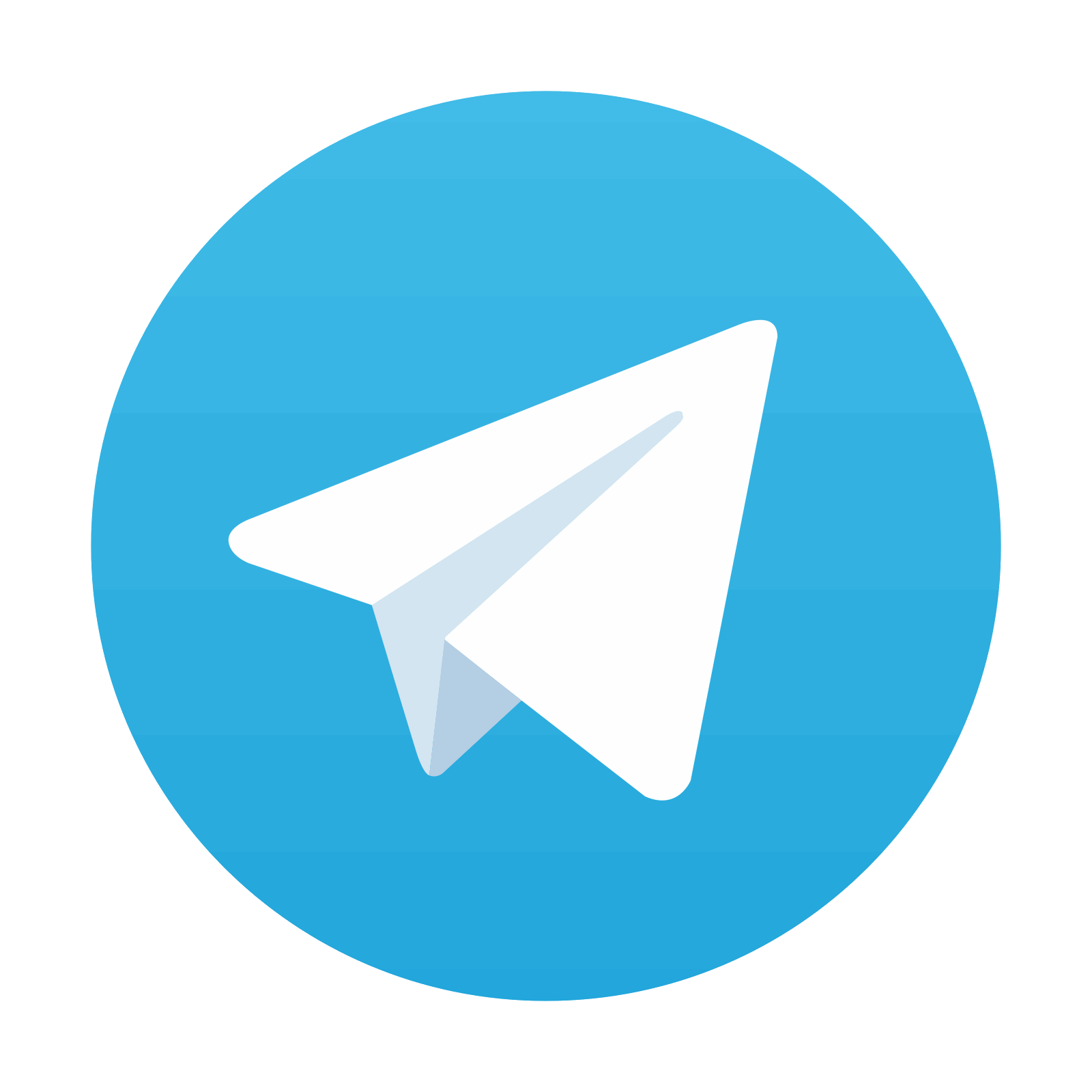
Stay updated, free articles. Join our Telegram channel

Full access? Get Clinical Tree
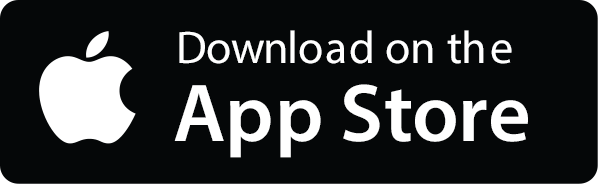
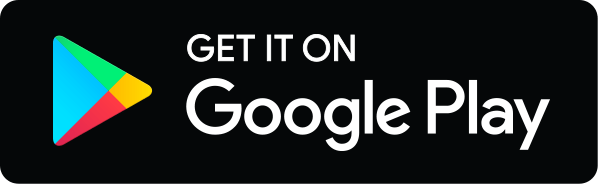