Objective
This study aimed to determine the usefulness of novel parameters of left ventricular (LV) endocardial area strain (AS) in assessing global LV performance in patients with repaired tetralogy of Fallot (TOF).
Methods
Thirty patients, aged 21.7 ± 5.8 years, with repaired TOF and 25 healthy subjects aged 19.9 ± 5.5 years were studied. Three-dimensional LV wall motion tracking was performed to determine global and regional peak AS, an AS-systolic dyssynchrony index (SDI), and LV volumes and ejection fraction. The AS-SDI was derived from the dispersion of time-to-minimum segmental endocardial areas, expressed as a percentage of RR interval, using a 16-segment model. The LV global performance plot was generated by plotting peak global AS against AS-SDI.
Results
The coefficients of variations for intra- and interobserver measurement of LV global AS were 6.3% and 10.6%, respectively. Compared with controls, patients had significantly lower global AS (30.5% ± 4.5% vs. 40.9% ± 2.8%, P < .001) and greater AS-SDI (10.0% ± 2.9% vs. 4.1% ± 1.4%, P < .001). Global AS ( r = 0.94, P < .001) and AS-SDI ( r = −0.76, P < .001) correlated strongly with LV ejection fraction. The global performance plot identified 87% of patients (26/30) with both reduced LV global AS and LV systolic mechanical dyssynchrony.
Conclusion
AS measurement of LV endocardium based on three-dimensional wall motion tracking is reproducible and enables convenient assessment of global LV performance using a composite representation of parameters, including global AS and an AS-derived index of mechanical dyssynchrony.
Although right ventricular (RV) dysfunction has in the past been the focus of attention in patients after repair of tetralogy of Fallot (TOF), the importance of left ventricular (LV) dysfunction as an important independent risk factor of long-term adverse outcomes is increasingly recognized. LV dysfunction, possibly related to an unfavorable ventricular–ventricular interaction, is characterized by reduced ejection fraction, impaired myocardial deformation, and systolic mechanical dyssynchrony. In patients after TOF repair, assessment of LV function using conventional M-mode echocardiography is limited by the paradoxical motion of the ventricular septum, whereas evaluation of LV myocardial strain and dyssynchrony requires separate assessments by a combination of different echocardiographic modalities, including tissue Doppler imaging, speckle tracking, and three-dimensional echocardiography.
A novel parameter of LV function, area strain (AS), based on three-dimensional wall motion tracking was recently introduced for use in the clinical arena. AS reflects deformation of the LV endocardial surface during contraction and relaxation. Because area is the product of length and width, AS can be regarded as a parameter integrating the regional consequences of deformation in longitudinal, circumferential, and radial dimensions. In addition, differences in time-to-minimum segmental area among various segments reflect synchronicity of LV contraction. Simultaneous assessment of LV global AS and an AS-derived index of dyssynchrony might allow comprehensive evaluation of global LV performance.
The present study aimed to determine the usefulness of AS parameters and a novel LV global performance plot in the assessment of LV performance in patients with repaired TOF.
Materials and Methods
Subjects
Thirty patients (18 male), aged 21.7 ± 5.8 years (range, 9.9–30.9 years), who had undergone surgical repair of TOF were recruited from the pediatric cardiac and adult congenital heart clinic. Eight of the 30 patients had been evaluated in our earlier three-dimensional echocardiographic study conducted 4 years ago. The following clinical data were collected from the case records of patients: patient demographics, age at operation, types of previous procedures, and duration of follow-up since surgical repair of TOF. Twenty-five healthy subjects (11 male), aged 19.9 ± 5.5 years (range, 11.4–31.9 years), were recruited as controls. These healthy controls were 1) subjects followed up for nonspecific chest pain and palpitation but without documented cardiac causes and 2) healthy siblings of patients. The body weight and height of all subjects were measured, and the body surface area was calculated accordingly. The institutional review board approved the study, and all the subjects and parents of minors gave informed consent.
Conventional Echocardiographic Assessment
Echocardiographic imaging was performed using the Artida Ultrasound System (Toshiba Medical Systems, Tokyo, Japan). All echocardiographic recordings were stored in an external hard disc for offline analysis. Measurements were made in three cardiac cycles, and the average was used for statistical analysis.
From the apical four-chamber view, the RV end-systolic and end-diastolic areas were measured, and the fractional area change was calculated accordingly. Eccentricity of the left ventricle was determined from two-dimensional images acquired from the parasternal short-axis view. The maximum distance from the endocardial surface of the mid-ventricular septum to that of the posterior-lateral LV free wall (D1) and the distance of the orthogonal axis between the endocardial surface of the anterior and inferior LV free walls (D2) were measured at both end systole and end diastole. The LV systolic eccentricity index (EI) was calculated as D2/D1 measured at end systole, and the diastolic EI was D2/D1 measured at end diastole. A larger EI signifies greater LV compression. The severity of pulmonary regurgitation was graded semiquantitatively using color Doppler imaging.
Tracking of LV Endocardium
Real-time three-dimensional echocardiographic imaging was performed from the apical view using a matrix array transducer interfaced to the Artida Ultrasound System. Full-volume acquisition, in which four adjacent subvolumes were captured over four consecutive cardiac cycles, was performed during breathhold to minimize the artifact between the subvolumes. Care was taken to include the entire LV cavity within the full-volume dataset.
The datasets were analyzed using the three-dimensional wall motion tracking software (Toshiba Medical Systems). On the basis of the selected high-quality three-dimensional dataset, a five-plane view that included an A plane (apical four-chamber, view), B plane (two-chamber view orthogonal to plane A), and three C planes (short-axis views near the apex, at mid-level, and at the base of the left ventricle) were displayed. Three markers were set on planes A and B at the apex and the two edges of the mitral valve ring. The LV endocardium was then traced automatically by the software, and the tracings were verified and adjusted manually where necessary on the basis of the five-plane view. Endocardial movements during cardiac systole and diastole were then tracked in three dimensions automatically ( Figure 1 ). On the basis of the endocardial tracking, an LV three-dimensional cast was obtained, which allowed the calculation of LV systolic and diastolic volumes and LV ejection fraction. For assessment of regional AS, the left ventricle was divided into 16 segments as defined by the American Society of Echocardiography. An AS-derived systolic dyssynchrony index (AS-SDI) was derived from the standard deviation (SD) of the 16 time-to-minimum segmental areas as a percentage of the RR interval. The LV global performance plot was generated by plotting the peak global AS based on all of the 16 segments against the AS-SDI.

Reproducibility of Global AS
Twenty subjects, 10 patients and 10 control subjects, were analyzed offline at different times for intra- and interobserver variability. Blinding was ensured by measuring the AS parameters using selected datasets on different days by the same observer (SNL) for intraobserver variability and by independent assessments by two observers (SNL and SJW) for interobserver variability.
Statistical Analysis
Data are expressed as mean ± SD. The RV areas and LV volumes were indexed by body surface area for further analysis. The absolute values of global and regional AS parameters were used for analysis to facilitate presentation and interpretation. The intra- and interobserver variabilities were reported as the coefficients of variation, calculated by dividing the SD of the differences between measurements by the mean and expressed as a percentage. Reproducibility of global AS assessment was further determined by Bland-Altman analysis of the intra- and interobserver datasets. Differences in demographic and echocardiographic parameters between patients and controls were compared using the unpaired Student t test. Pearson correlation analysis was used to assess the relationships between AS parameters and LV ejection fraction. The receiver operating characteristic (ROC) curve was used to evaluate the usefulness of AS and AS-SDI as markers for reduced LV ejection fraction <55%, which has been shown to be a predictor of major adverse outcome late after TOF repair. A two-tailed P value < .05 was considered statistically significant. All statistical analyses were performed using SPSS 16.0 (SPSS, Chicago, IL).
Results
Subjects
The 30 patients underwent surgical repair of TOF at 4.5 ± 3.7 years of age. All of the patients were asymptomatic at the time of study. Pulmonary regurgitation was moderate to severe in 17 patients, mild in 12 patients, and absent in 1 patient. The age ( P = .23) and sex distribution ( P = .25) were similar between patients and controls. Compared with controls, patients had similar body surface area (1.6 ± 0.3 m 2 vs. 1.5 ± 0.2 m 2 , P = .14), although their body weight tended to be heavier (56.1 ± 4.7 kg vs. 49.7 ± 9.0 kg, P = .06).
Observer Variability
The coefficients of variation for intra- and interobserver measurements of LV global AS were 6.3% and 10.6%, respectively. Bland-Altman analysis showed that there was no tendency for the difference between the two readings to vary with the magnitude of mean global AS ( Figure 2 ).
Ventricular Size and Geometry
Compared with controls, patients had significantly larger RV end-systolic area (12.0 ± 4.8 cm 2 /m 2 vs. 4.6 ± 1.3 cm 2 /m 2 , P < . 001), end-diastolic area (20.2 ± 5.3 cm 2 /m 2 vs. 9.6 ± 2.0 cm 2 /m 2 , P < . 001), LV systolic EI (1.2 ± 0.1 vs. 1.0 ± 0.0, P < . 001), LV diastolic EI (1.3 ± 0.2 vs. 1.0 ± 0.0, P < . 001), and LV end-diastolic volume (28.6 ± 6.1 mL/m 2 vs. 24.0 ± 5.5 mL/m 2 , P = .005). On the other hand, the LV end-diastolic volume (53.5 ± 9.9 ml/m 2 vs. 60.5 ± 13.5 mL/m 2 , P = .03), LV ejection fraction (46.6% ± 5.0% vs. 60.5% ± 1.7%, P < . 001), and RV fractional area change (41.4% ± 11.9% vs. 52.1% ± 10.7%, P = .001) were significantly lower in patients than in controls.
AS Parameters
Representative AS analyses of the three-dimensional datasets in a patient and a control are shown in Figure 1 . The peak global AS was significantly lower in patients than controls (30.5% ± 4.5% vs. 40.9% ± 2.8%, P < . 001). Table 1 summarizes the regional AS of the 16 LV segments. Of the 16 segments, 10 showed significant differences between patients and controls. The differences were most significant ( P < . 001) between septal segments of the two groups.
Patients ( n = 30) | Controls ( n = 25) | P | |
---|---|---|---|
Peak segmental AS (%) | |||
BA | 35.0 ± 10.7 | 49.6 ± 8.7 | <.001 ∗ |
BAS | 27.2 ± 10.6 | 52.8 ± 12.3 | <.001 ∗ |
BS | 35.0 ± 9.4 | 49.0 ± 11.3 | <.001 ∗ |
BI | 43.6 ± 13.2 | 44.0 ± 9.3 | .91 |
BP | 49.5 ± 9.6 | 48.9 ± 7.7 | .82 |
BL | 44.7 ± 13.2 | 48.7 ± 9.3 | .21 |
MA | 27.6 ± 10.5 | 32.7 ± 8.4 | .06 |
MAS | 24.4 ± 13.1 | 35.1 ± 8.8 | .001 ∗ |
MS | 28.0 ± 7.4 | 40.3 ± 6.2 | <.001 ∗ |
MI | 34.6 ± 9.6 | 42.8 ± 7.4 | .001 ∗ |
MP | 36.5 ± 10.1 | 41.0 ± 5.5 | .041 ∗ |
ML | 36.0 ± 11.0 | 35.0 ± 7.4 | .71 |
AA | 24.8 ± 13.2 | 32.7 ± 10.2 | .017 ∗ |
AS | 27.4 ± 12.2 | 42.4 ± 9.8 | <.001 ∗ |
AI | 30.2 ± 15.6 | 43.6 ± 11.4 | .001 ∗ |
AL | 31.0 ± 15.9 | 37.1 ± 12.7 | .13 |
Peak global AS (%) | |||
30.5 ± 4.5 | 40.9 ± 2.8 | <.001 ∗ |
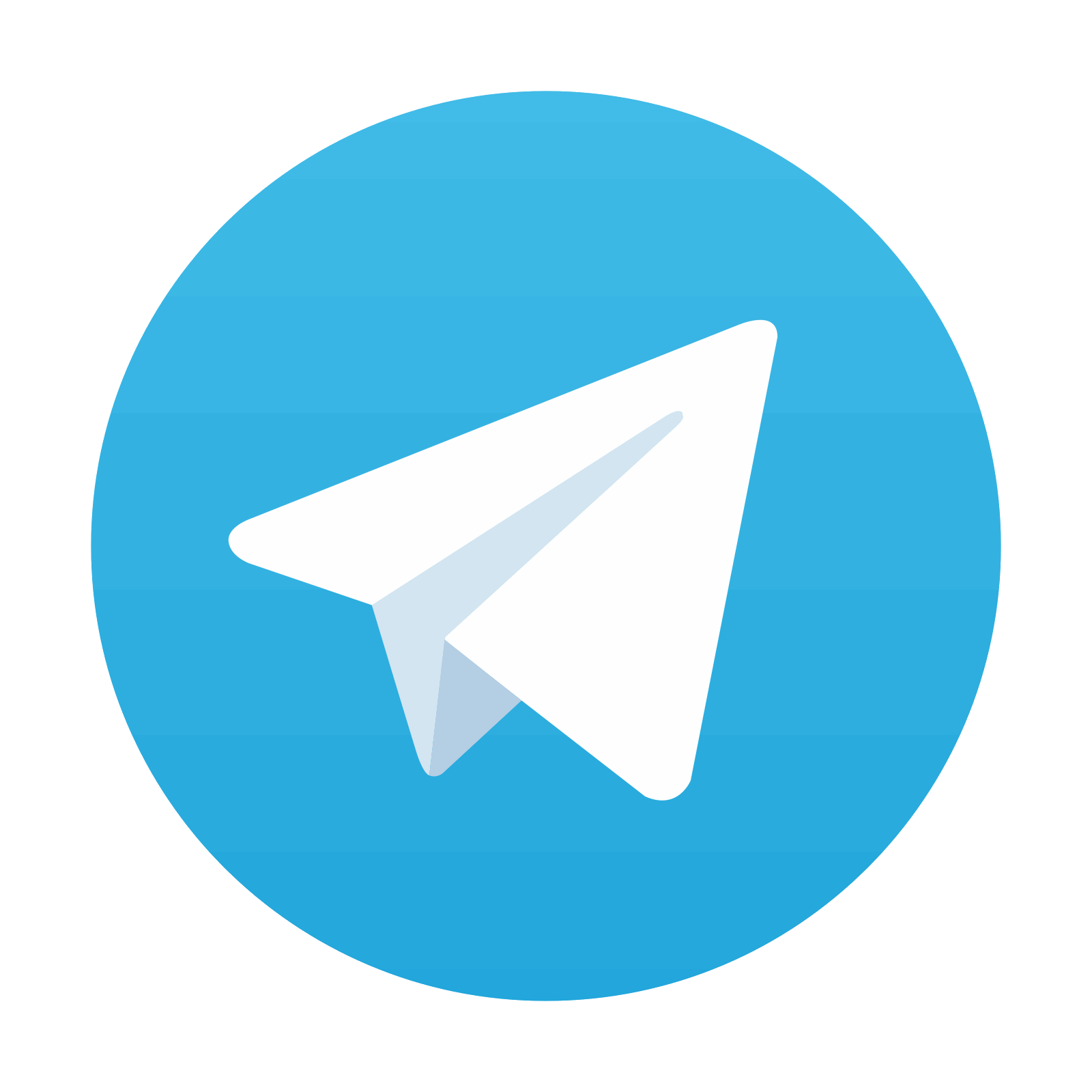
Stay updated, free articles. Join our Telegram channel

Full access? Get Clinical Tree
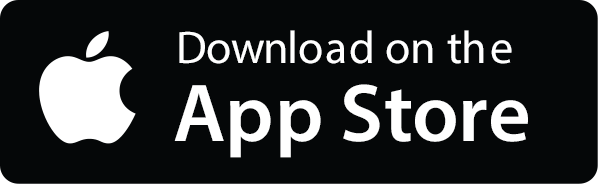
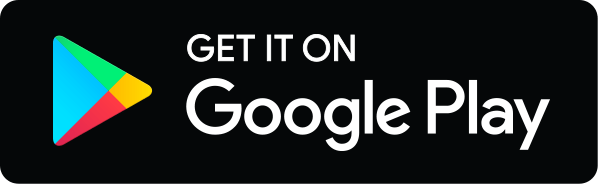
