The normal heart is composed of two ventricles that circulate blood through the pulmonary and systemic capillary beds in series, providing a means of delivering oxygen and nutrients to the tissues and removing carbon dioxide and metabolic waste. Deoxygenated blood is received in the right atrium and pumped from the right ventricle through the pulmonary capillary bed, where carbon dioxide is exchanged for oxygen in the alveoli. Pulmonary venous return to the left atrium is circulated through the systemic capillary bed by the left ventricle (Figure 1-1).
The term “cardiac cycle” refers to the events that occur in 1 heartbeat. By convention, the cycle is said to begin with the onset of systole. Notably, the events in the right and left heart occur in parallel, in the same sequence and at roughly the same time, although at different pressures. These events are tied to the electrical activities of the heart in a process described as electromechanical coupling. Figure 1-2 depicts the events of the cardiac cycle, including the surface electrocardiogram (ECG) and intracardiac pressures and volumes.
In diastole, the ventricles fill with blood, which occurs in two phases. In the first phase, the ventricle fills passively as the ventricular myocardium relaxes and blood is drawn into the ventricles through the atrioventricular (AV) valves as the pressure in the ventricle drops below that of the atria. Next, depolarization of the sinus node, located in the superior portion of the right atrium, initiates atrial contraction. On the surface ECG, atrial systole inscribes a P wave. As the atria contract, additional blood is pumped into the ventricles—the second phase of diastole. Meanwhile, the wave of depolarization travels along the atrial wall toward the ventricles. It ultimately reaches the AV node near the medial aspect of the tricuspid valve. The AV node funnels the impulse into the bundle of His, which rapidly conducts the impulse into the ventricles.
On the surface ECG, ventricular depolarization results in the inscription of the QRS complex. As the ventricles start to contract, the ventricular pressure begins to rise, and the AV valves close when the pressure in the ventricle exceeds that of the atria. A period called isovolumetric contraction follows, as the ventricular pressure increases without a change in volume, as both the AV valves and the semilunar valves are closed. As the pressure in the ventricles increases, it soon exceeds the aortic and pulmonary artery pressure and causes the aortic and pulmonary valves to open, ejecting blood through the great arteries. As the blood is ejected, the ventricular pressure falls. At this point, the ventricular myocardium has begun to repolarize. Ventricular repolarization results in the inscription of the T wave on the surface ECG. When the ventricular pressure drops below that of the great artery, the semilunar valve closes. As the ventricular pressure continues to fall and becomes lower than the atrial pressure, the AV valve opens. The cycle then begins anew.
Cardiac output is determined by several factors. The term stroke volume has been used to describe the amount of blood that is ejected in 1 cardiac cycle. Thus, the stroke volume multiplied by the heart rate will yield a volume per unit time. Traditionally, cardiac output is described in liters per minute. The cardiac index is the cardiac output divided by body surface area, a common way of correcting or normalizing the cardiac output for patient size, and is reported as liters per minute per meter squared. This is useful in that a normal adolescent may have a cardiac output many times that of an infant, but the cardiac index would be similar when adjusted for body size.
Starling’s law states that stroke volume will vary as a function of the amount of blood that fills the heart during diastole. The ventricular filling pressure is referred to as preload. The unfilled ventricle cannot generate any pressure because it must be filled in order to squeeze. The increased stroke volume ejected during systole in response to increased preload is a property of the myocytes themselves. Increasing preload increases stroke volume by increasing the end-diastolic volume, keeping the end-systolic volume the same. Under normal loading conditions, increases in end-diastolic volume do not result in drastically increased end-diastolic pressure; however, when stretched to near-maximum length, the myocytes reach a threshold at which they are unable to accommodate additional volume without relatively large increases in pressure. The relationship between pressure and volume is illustrated in Figure 1-3.
Diastolic dysfunction occurs when relatively normal end-diastolic volumes are associated with high end-diastolic pressures. Diastolic dysfunction is characterized by a stiff, poorly distensible ventricle. Clinically, poor left ventricular compliance results in left atrial hypertension, which results in high pressures in the pulmonary veins. This, in turn, results in elevated hydrostatic pressure in the pulmonary capillaries and subsequent pulmonary edema, tachypnea, increased work of breathing, and respiratory insufficiency or failure.
The resistance in the systemic arteries, against which the left ventricle pumps, has been referred to as afterload. Stroke volume changes in response to alterations in afterload. Afterload can be thought of as the amount of work the heart muscle performs. As the afterload increases, the ventricle squeezes until the amount of pressure that it can generate equals the workload imposed by the vascular resistance. At a higher afterload, the stroke volume decreases because contraction stops when the pressure-generating capacity of the ventricle has been reached and the end-systolic volume is increased. Clinically, the hypertensive patient, with high systemic vascular resistance, will manifest a lower stroke volume. This would result in decreased cardiac output, as described earlier.
The ventricular myocytes shorten during contraction as a result of the actin-myosin cross-bridge formation. Under the influence of increased sympathetic tone, the pressure-generating capacity of the ventricle increases related to the availability of intracellular calcium. This results in a more vigorous squeeze and a lower end-systolic volume, increasing the stroke volume. Exercise results in a high catecholamine state, which leads to increased contractility. This augments both heart rate and stroke volume, leading to increased cardiac output in response to the increased metabolic need. Conversely, an excessively high or prolonged catecholamine state can increase the stiffness of the ventricle and ultimately reduce contractility. In addition, decreased contractility can result from metabolic derangements, such as acidosis or myocardial ischemia.
Although described in relation to electrical circuits in terms of voltage, resistance, and current, Ohm’s law applies to hydraulic circuits as well. Ohm’s law states that pressure is equal to resistance multiplied by flow. Importantly, it is not the preload, afterload, or contractility of the ventricle that determines the pressure in a vascular bed. The flow, typically described in liters per minute, and the resistance, measured in Wood units, dictates the pressure. The resistance in a vascular bed is determined by both the muscular tone in the arteries and the downstream resistance to flow through the capillary bed. For example, in pulmonary hypertension, it is not right ventricular contractility that causes the elevated pulmonary artery pressures; the elevated pulmonary vascular resistance is the primary derangement, and the elevated right ventricular pressure is a necessary compensation. In pulmonary hypertension, due to increased blood flow in the pulmonary arteries from intracardiac shunting, an increase in smooth muscle mass in the pulmonary arterial walls occurs. With prolonged exposure to high rates of flow, the increased smooth muscle mass and tone becomes irreversible, and a permanent state of pulmonary hypertension ensues. This clinical scenario occurs in the setting of a large, unrepaired ventricular septal defect (VSD) and results in Eisenmenger syndrome.
When gathering the history of a patient with suspected cardiac disease, the importance of both the antenatal and birth history should not be underestimated; any such history is invaluable. Many pregnant women undergo a screening ultrasound at around 20 weeks gestation, which may prompt a fetal echocardiogram if any concerning anomalies are detected; similarly, any history of fetal arrhythmias should be noted. Details, such as a history of taking prenatal vitamins, and any complications during the pregnancy should be investigated. Especially in the newborn period, the circumstances surrounding the birth should be elicited in detail. Information, including Apgar scores, the need for resuscitation in the delivery room, birth weight, nursery course, duration of stay, and discharge medications should all be ascertained in the course of a first office visit. Any perinatal respiratory distress, cyanosis, murmur, or difficulty feeding should be explored for possible cardiac etiologies.
Commonly, cardiac disease in the infant or child will present with respiratory symptoms, cyanosis, or shock. In the first several months of life, tachypnea and increased work of breathing can be signs of heart failure, although the mechanism is very different from that of the adult with heart failure due to ischemic cardiomyopathy. Sweating when nursing or bottle-feeding can be a sign of heart failure in infants. Cyanosis or shock should prompt rapid evaluation in the emergency room setting to rule out life-threatening problems, such as cyanotic heart disease, severe pulmonary disease, or foreign-body aspiration.
Other elements of the history that can be helpful when screening patients for potential cardiac pathology include reports of chest pain, fainting or dizzy spells, palpitations, heart murmur, or other cardiac diagnosis.
A complete understanding of the medical history informs the diagnostician and allows the necessary exchange of relevant information with other practitioners. Likewise, important clues to systemic illnesses or genetic conditions that may be associated with cardiac diseases or defects can be revealed.
The etiology of congenital heart disease continues to be a topic of much investigation. Congenital heart disease in a sibling or parent increases the chances of congenital heart disease in an individual. A family history of genetic syndromes should also be explored. Sudden death can have heritable causes, such as hypertrophic cardiomyopathy, long QT syndrome, or Marfan syndrome. An initial history should include questions about relatives who have died suddenly or unexpectedly, such as those who might have died of sudden infant death syndrome or while swimming or driving alone, especially when unexplained death occurs under age 35 years. Other family history to be ascertained includes syncope, seizures, arrhythmias, and other cardiac diseases presenting under the age of 50 years.
Poor growth can be a manifestation of cardiac pathology. Infants with congestive heart failure do not grow well, due to a diversion of metabolic resources to maintaining systemic cardiac output, rather than somatic growth.
Fever can cause tachycardia, increase the intensity of an innocent murmur, or accompany infectious or inflammatory diseases that can affect the heart, such as infectious endocarditis, rheumatic fever, or rheumatoid arthritis.
Heart rate should be noted and compared to established normal ranges for age, taking into account the patient’s emotional and physical state. Anxiety, fear, and agitation commonly cause sinus tachycardia in the examination room and prevent an accurate assessment of the resting heart rate. Resting sinus tachycardia has a long differential diagnosis that cannot be fully explored here but should raise concern for underlying disease. Supraventricular tachycardia generally occurs at heart rates greater than 150 to 180 beats per minute and is often too fast to count accurately.
Bradycardia occurs in the setting of excellent physical conditioning, as well as with eating disorders, heart block, elevated intracranial pressure, sinus node dysfunction after a history of cardiac surgery, and hypothyroidism.
Blood pressure should be measured in the seated position, with the patient’s feet on the ground, the patient’s back supported, and the patient resting quietly and calmly for 5 minutes. The use of electronic blood pressure monitoring devices is commonly used in practice, but measuring blood pressure using a manual cuff to confirm abnormal results should be performed. The blood pressure cuff should be the appropriate size for the patient’s arm, and the sphygmomanometer should be calibrated every 6 months. The blood pressure in the right upper extremity should be measured and compared to the blood pressure in either leg. The right upper extremity blood pressure will likely be equivalent to, or less than, that of the leg. However, if the right upper extremity blood pressure is greater than the blood pressure in the leg, a diagnosis of coarctation of the aorta should be entertained. Normal values for blood pressure are based on sex, age, and height percentiles and are widely published for comparison.1,2
Respiratory rate should also be noted and compared to age-related normal values. Historical data should also be taken into account when tachypnea is present, as in the case of an ongoing viral respiratory tract infection. Tachypnea, as mentioned earlier, can be a sign of congestive heart failure in infants resulting from pulmonary overcirculation and edema.
Oxygen saturation should be part of the routinely measured vital signs. In the newborn period, desaturation can be a sign of cyanotic congenital heart disease and should prompt urgent referral. Additionally, differential desaturation between the upper and lower extremities, which refers to decreased saturations in the lower extremities compared to the upper, is due to deoxygenated blood entering the descending aorta via a patent ductus arteriosus (PDA) and requires further evaluation.
The examiner should regard the patient overall, noting any indication that the patient is distressed or agitated. Separate from behavioral causes, agitation may be a sign of distress in all age groups. The nutritional status of the patient also should be assessed, as well as the interaction between patient and parent or guardian.
Dysmorphic features may signal the presence of a genetic abnormality. Associations between genetic anomalies and congenital heart disease are discussed elsewhere.
Cyanosis indicates the presence of at least 5 g/dL of deoxygenated hemoglobin, regardless of the amount of oxygenated hemoglobin. Patients with high hemoglobin concentrations need a smaller fraction of their total hemoglobin to be deoxygenated to appear cyanotic. Conversely, a patient who is anemic would require a large percentage of their total hemoglobin to be deoxygenated to have the same appearance. For example, a chronically hypoxemic patient with a total hemoglobin of 20 g/dL would require only 25% of their hemoglobin to be deoxygenated to be cyanotic, whereas an anemic patient with a hemoglobin of 7 g/dL would require greater than 50% deoxyhemoglobin to be cyanotic. Cyanosis is best evaluated in a well-lit room. The oral mucous membranes, gums, and tongue should be inspected because they will not be affected by peripheral vasoconstriction due to temperature or elevated vascular tone or be obscured by skin pigmentation.
Labored respirations and tachypnea may result from pulmonary overcirculation and pulmonary edema in the infant with a large left-to-right shunt. Pulmonary edema may also result from left heart failure or from hypoplastic structures in the left heart with inadequate systemic blood flow. Additionally, hypoxemia from severe cyanosis or acidosis can cause respiratory distress.
Jugular venous distention is a rare finding in pediatrics. In the newborn, the neck is relatively short and not easily visible. In older children, even those with elevated right atrial pressures and hepatomegaly, jugular venous distention may not be evident. The hepatojugular reflex, compressing the liver during inspection of the neck veins to see if they become more prominent, should be performed with the patient in the supine position with the head elevated to 30 degrees.
Prominence of the left chest can indicate the presence of right ventricular enlargement. An absence of muscle mass in the left chest can also indicate Poland anomaly, or hypoplasia of the pectoralis minor, which can be associated with congenital cardiac defects. Pectus deformities, such as pectus excavatum and scoliosis, can be associated with Marfan syndrome. Kyphosis and scoliosis can lead to restrictive lung disease and pulmonary hypertension.
The point of maximal impulse (PMI) can often be seen in the normal child and should be located in the fourth to fifth intercostal space, at the midclavicular line. If it is displaced to the left or inferiorly, it can indicate cardiomegaly.
Peripheral edema is caused by systemic venous congestion and is a rare finding in young children. Elevated systemic venous pressure is a feature of advanced right heart dysfunction. Typically, edema in children is due to renal or hepatic disease, although patients with decompensated, postoperative single-ventricle physiology may exhibit this finding due to elevated central venous pressure.
Diaphoresis is another term for perspiration. Infants in heart failure can become diaphoretic when feeding, which is related to the increased adrenergic tone that results from chronic, low cardiac output. If related to heart failure, other signs of distress, such as tachypnea and poor growth, should accompany this. Diaphoresis secondary to environmental factors in the absence of other findings does not indicate the presence of occult heart disease. For example, sweating occurs normally during periods of high vagal tone, such as sleeping.
Prolonged hypoxemia and polycythemia result in engorgement of the capillaries beneath the nail beds in the fingers and toes. As a result, the concavity formed by the eponychium (cuticle) disappears, giving the appearance of a thick, wide distal phalanx. Clubbing also occurs secondary to disease in other organ systems; cirrhosis and cystic fibrosis are two examples.
When palpating the pediatric chest to assess for the PMI, the examiner should use the most sensitive part of the hand. The patient should be in the upright position. Many find the volar surface of the proximal fingers (metacarpal heads and proximal phalanges) to be the most sensitive. Others find that the fingertips are adequate. If the PMI is palpated in the right chest, dextrocardia should be considered.
Often, a hyperdynamic right ventricle is associated with an indistinct impulse. A hypertrophied right ventricle, associated with elevated pressure, produces a relatively short “tap” that can be appreciated at the left lower sternal border. An enlarged right ventricle due to increased volume loading, on the other hand, results in a more diffuse “lift,” or “heave,” that is gradual in onset.
As stated earlier, the examiner palpates a normal PMI at the left lower sternal border, in the fourth to fifth intercostal space, at the midclavicular line. If the left ventricle is dilated, the PMI is shifted inferiorly and leftward. A hyperdynamic PMI in the correct location may be a sign of increased cardiac output.
When the turbulent blood flow that results in a murmur can be palpated on the chest wall, this is called a thrill. Thrills can be felt in the precordial, suprasternal, and carotid areas and result from significant cardiac pathology. If a murmur is low grade but a thrill is palpated, then the finding is more likely a pulsation and not a thrill. Any child with a murmur loud enough to result in a true thrill should be referred to a cardiologist.
Peripheral pulses should be assessed for their presence, strength, and regularity. The prominence of the pulses is important. Pulses are classically graded on a scale of 0 to 4: 0 = absent pulses; 1 = barely palpable pulses; 2 = easily palpable pulses; 3 = full pulses; and 4 = bounding pulses. A pulse that feels “bounding” may be the result of a “run-off” lesion, such as aortic insufficiency or PDA. This finding results from a very wide pulse pressure; in the case of aortic insufficiency, the backflow of blood into the ventricle lowers the diastolic pressure. This has been referred to as a “water-hammer” pulse, so named for the Victorian-era toy. A diminished pulse in the setting of clinical instability can be a sign of low cardiac output, indicating that urgent resuscitation is needed.
The term pulsus paradoxus refers to an exaggeration of the normal reduction in pulse pressure seen during inspiration from conditions that limit the size of the pericardial space, such as cardiac tamponade and constrictive pericardial disease. The normal difference in systolic pressure during respiration should be less than 10 mm Hg; values greater than this should raise concern for tamponade.
Sinus arrhythmia is the physiologic increase in heart rate during inspiration and decrease in expiration and is a response to increased preload generated when the intrathoracic pressure decreases during inspiration. This is a normal finding and should disappear with breath holding. Long pauses between pulsations or early pulsations require further investigation.
Coarctation of the aorta, a constriction that develops distal to the left subclavian artery, results in upper extremity hypertension and diminished pulses in the lower extremities. Blood pressures in all four extremities should be checked in any pediatric patient with upper extremity hypertension to rule out coarctation of the aorta. Simultaneous palpation of the right radial and either femoral pulse should be performed to assess for pulse lag and for the presence of a femoral pulse. Lag between the upper and lower extremities or absence of the femoral pulse is suggestive of a coarctation.
Cardiomegaly can be detected in severe cases by percussing the thorax. Similarly, pleural effusions or pulmonary consolidation can be identified. Percussion is used to augment the cardiac examination in determining the span of the liver. In cases where the lungs are hyperinflated, as in reactive airways disease, inferior displacement of the diaphragm can allow palpation of the liver edge below the costal margin. Percussion of a normal liver span can reassure the examiner that the liver is not enlarged, such as in congestive heart failure, but simply displaced. Enlargement of the spleen typically does not occur without enlargement of the liver; however, isolated splenomegaly can occur in endocarditis.
Auscultation of the heart in a child is often easier to do compared with adolescents or adults, because the chest wall is thinner and there is less space between the chest wall and the heart. This results in clearer auscultation and easier recognition of heart sounds. Conversely, it can be more difficult in younger patients due to poor patient cooperation and higher heart rates. Because auscultation is dependent on the patient being calm and quiet, it is recommended that the provider perform this early in the examination, to ensure improved likelihood of having an accurate and complete cardiac assessment.
A stethoscope should be comfortable for the examiner to use. Using the same stethoscope consistently will eliminate inconsistencies in the acoustic qualities of different stethoscopes. The tubing should also be less than 18 inches in length to avoid attenuation of sound transmission. The bell of the stethoscope lets in all sounds, whereas the diaphragm is a high-pass filter, letting in higher pitched sounds. Therefore, sounds heard with the bell alone are considered low pitch; those heard solely with the diaphragm are high pitched; and those heard with both are considered medium pitched. The bell should be applied lightly to the chest wall so as not to cause the skin to act as a diaphragm. The diaphragm should be applied with more pressure to eliminate apposition abnormalities. In addition, the diaphragm should not be too large for the chest, which will also cause auscultated apposition artifacts.
The first heart sound coincides with the AV valves closing and can be best heard at the left lower sternal border. Although the tricuspid and mitral valves close at this time, S1 is usually heard as a single sound. Compared with the second heart sound, S1 is typically lower pitched and longer. If there is any confusion as to which heart sound is which, palpating the carotid pulse while auscultating can be helpful; S1 precedes the pulsation, and S2 follows it.
The second heart sound results from closure of the 2 semilunar valves, the pulmonic and aortic valves. Splitting of the second heart sound, the ability to discern that there are 2 sounds as opposed to 1, occurs normally with respiratory variation. The sounds are abbreviated A2, for aortic valve closure, which is best heard in the right second intercostal space, and P2 for the sound of pulmonic valve closure, which is heard at the left second intercostal space. A2 normally occurs prior to P2. With inspiration, the downward movement of the diaphragm creates negative intrathoracic pressure. Blood is pulled from the vena cavae into the right atrium and pumped into the right ventricle. During ventricular contraction, the larger volume of blood in the right ventricle, compared with the left ventricle, takes longer to eject. This prolongation in ejection time results in delayed closure of the pulmonic valve and splitting of the second heart sound. Other alterations in intensity and timing of the second heart sounds are summarized in Table 1-1.
Finding | Structural Causes | Functional Causes |
---|---|---|
Widely split |
|
|
Narrowly split |
|
|
Single |
|
|
Paradoxically split |
|
|
Increased intensity |
| |
Decreased intensity |
|
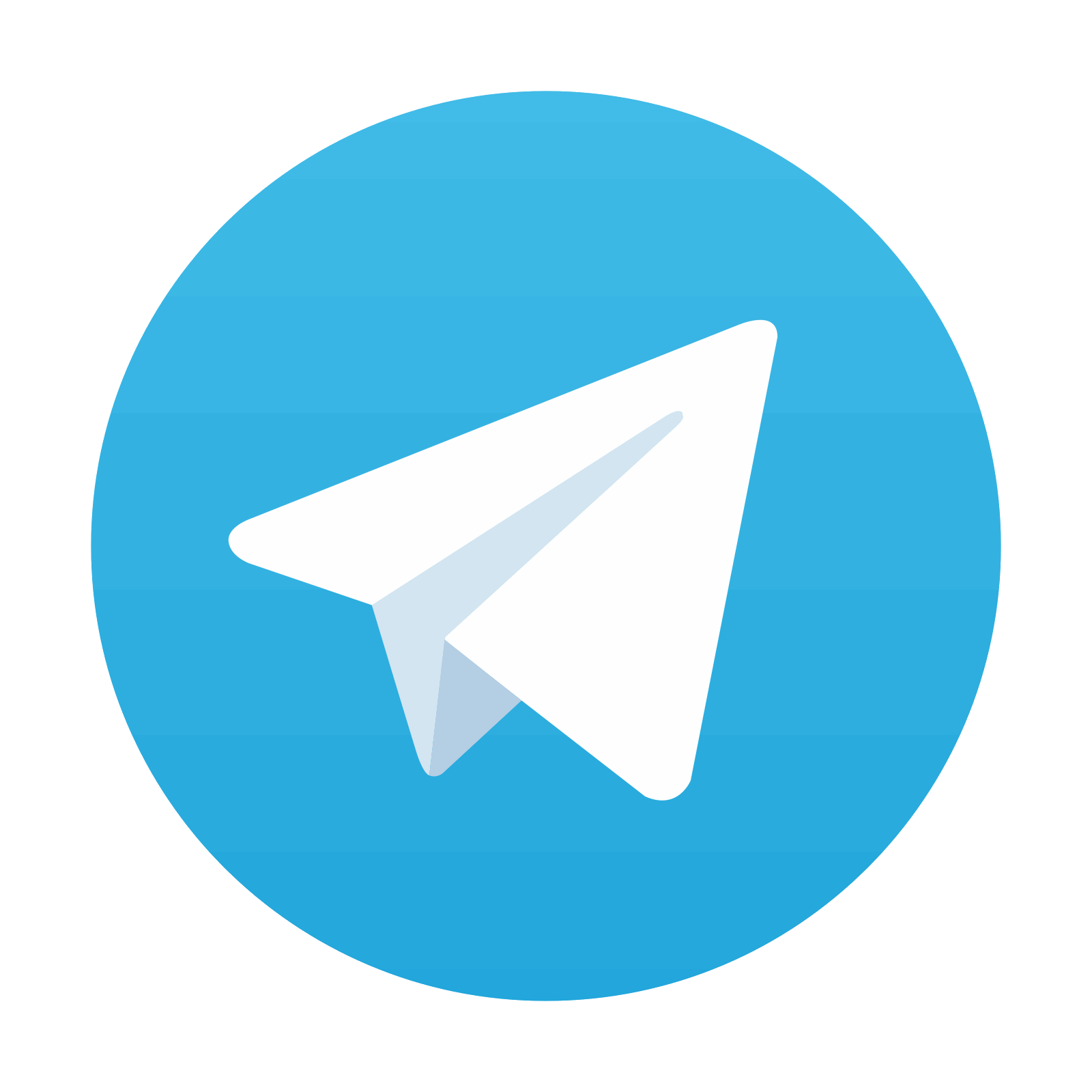
Stay updated, free articles. Join our Telegram channel

Full access? Get Clinical Tree
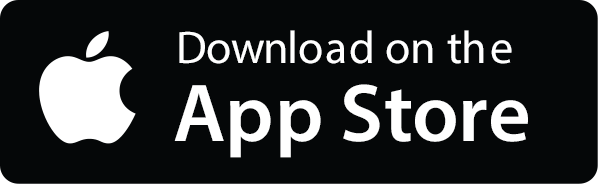
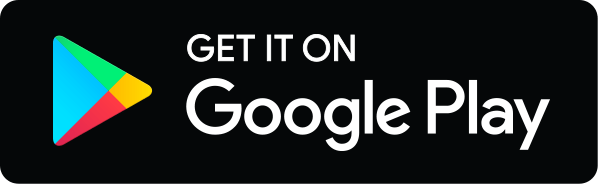
