Lung cancer remains the leading cause of cancer death for both men and women.1 Only one-third of patients diagnosed with lung cancer are stage appropriate for surgical therapy. In addition, the rising population of elders in the United States and other Western countries has caused an increase in the number of patients with comorbidities, making primary surgical and curative therapy more complicated. In fact, a review of the California cancer registry revealed that stage I cancer patients who received no treatment have a median survival of 9 months. Eighty-nine percent of patients who were recommended for treatment but refused died within 5 years, and 78% of these patients died from cancer-specific causes. For T1 lesions, the survival rate for treated cancers was 155 months versus 26 months for untreated cancers.2 For those patients treated with conventional external radiation alone, 5-year survival is 10% to 30%.3 Hence, appropriate treatment even for patients with major comorbidities should be available.
Surgical resection remains the gold standard treatment for early-stage lung cancer. In addition, the lung is a frequent site of metastasis in that pulmonary metastases occur in 30% of all malignancies.1 However, a significant proportion of patients are unable or unwilling to undergo surgery. For these patients, ablative therapy may be an option for localized treatment. The technique of percutaneous tumor ablation has developed through the introduction of multiple modalities including microwave, cryoablation, high-intensity focused ultrasound scan, irreversible electroporation, interstitial laser, and radiofrequency ablation (RFA). The most studied and proven method among these is RFA.
Radiofrequency energy was first used in surgery with the application of cauterization in 1926, introduced by William T. Bovie, after pulsed electrical current was shown to cause coagulation in tissue, while continuous current resulted in cutting of tissue. The technique afforded precision cuts with minimal blood loss. Percutaneous RFA was first reported in 1990 for liver tumor ablation.4 Since then, the indications have expanded to other organs, such as kidney, bone, and lung. The mechanism of action and outcomes of treatment are reviewed.
The term, radiofrequency ablation, refers to the therapeutic use of electromagnetic radiation (EMR) for the selective destruction and removal of biologic lesions. Electromagnetic radiation exhibits wave-like behavior corresponding in magnitude to the light spectrum (e.g., microwaves, radio waves, infrared, visible light, ultraviolet, X-rays, gamma rays). RFA uses energy at the frequency and length of radio waves (2–300 Hz).5 A circuit is created that flows from the tip of the electrode, to a large, diffuse grounding pad placed over the patient’s thighs, to an external generator. The small cross-sectional area of the electrode creates a surrounding region of high energy flux. The molecules next to the electrode, usually water, align with the direction of the current. The rapidly alternating current causes the adjacent molecules to vibrate. Molecules farther away from the probe are affected by the other vibrating molecules. The frictional loss of energy between molecules results in a temperature increase.6 The electrode, itself, is not a source of heat, but creates the electromagnetic field that causes molecular movement, and hence heat generation.
Temperature control is an important issue in RFA. The temperature must be managed to allow energy to be conducted through the tissues. Excessive energy can cause build-up of char around the probe that insulates conduction and limits energy dissipation. With regard to the lung, the parenchyma is thermally insulated by the air-filled alveoli. Tumors within the lung will require different amounts of energy than those attached to the pleura, as the chest wall creates different resistive patterns.7
Tissue is sensitive to temperatures above 40°C.8 Protein denaturation and coagulation occur between 60°C and 100°C. The therapeutic effect of RFA diminishes when temperatures rise above 105°C, at which point the tissue starts to boil, vaporize, and carbonize. Gas formation increases tissue impedance, preventing heat expansion and limiting the ablative area. Carbonization creates insulation around the probe that prevents heat dissipation.
Heat transmission can also be affected by the local anatomy. Approximation to a vessel 3 mm or larger can create enough heat loss from the flow of lower temperature blood to prevent adequate ablation, the so-called “heat sink” effect. This can lead to incomplete or inadequate ablation of tumors in close proximity to vessels. Animal studies of lesions close to the heart and myocardium have demonstrated RFA to be safe with no damage to the myocardium, possibly owing to the loss of heat from the higher blood flows in the heart.9 Tumors in close proximity to the heart subsequently have higher rates of recurrence with a 69.3% recurrence at 1 mm distance versus 8.6% recurrence at 9 mm.10
The typical set-up for an ablation requires a generator, a probe, and a ground. The grounding pad is used to complete the circuit from the patient to the generator. It is often large and applied to both thighs to dissipate the charge without causing local heat effects. The radiofrequency generators are capable of outputting approximately 250 W with high-frequency alternating currents ranging from 460 to 500 kHz.5 With regard to the electrodes, three commercially available devices are the most popular. The Boston Scientific LeVeen ablation system uses an impedance-based feedback system to adjust its energy output (Fig. 84-1). The distal monopolar electrodes are deployed in an umbrella fashion with diameters of 2 to 5 cm. Covidien produces the formally Valleylab Cool-tip technology. The catheter is cooled internally with running saline to slow heating of adjacent tissue, thereby reducing tissue resistance. It uses impendence monitoring to adjust its energy output, and multiple electrodes can be clustered to allow for larger ablation zones (Fig. 84-2). Angiodynamics produces the radiofrequency interstitial tissue ablation (RITA) temperature-controlled system. They offer two probes, the StarBurst which has several electrodes that expands like a Christmas tree to a maximum size of 7 cm (Fig. 84-3). Real-time temperature readings at the distal electrodes are utilized to adjust energy output. Their Talon system is similar, but uses saline infusion into the tissue at the electrode tips to reduce charring and to allow for a more uniform distribution of heat.
Figure 84-3
AngioDynamics’ radiofrequency interstitial tissue ablation (RITA) temperature-controlled system offers several probes in the StarBurst family of devices. A. The StarBurst family of devices features several electrodes that expand like a Christmas tree with an ablation size ranging from 1 cm to 7 cm depending on the probe selected. B. The StarBurst Talon system infuses saline into the tissue at the electrode tips to reduce charring and to allow for a more uniform distribution of heat. (Reproduced with permission from Angiodynamics, Latham, NY.)

We prefer the Angiodynamics RITA system with Talon probes. Patients are worked up in the standard fashion including chest CT, PET/CT, and pulmonary function testing (PFT). Early stage or localized lesions are considered appropriate for treatment. Patients are brought into the CT scanner, placed under conscious sedation with fentanyl and Versed medication, and continuously monitored. A scout scan is performed to identify the lesion. Patients are placed in various positions to allow for the shortest route to the lesion, either supine, lateral decubitus, prone, or some variation thereof. Crossing lung fissures should be avoided to decrease the risk of pneumothorax. Local anesthesia is given on the skin and intercostal space while a straight tract is probed with the needle. Then, using CT fluoroscopic guidance, the electrode is delivered into the tumor. The tines are deployed and ablation started. Our protocol calls for a 10-minute ablation at 90°C. If pneumothorax is encountered, a pigtail catheter is placed to control the chest space. Once treatment is complete, the catheter is removed without the use of tract ablation. A CT scan is performed immediately after the procedure and again at 24 hours.
CT scanning is used to follow time-related changes post-RFA. Figure 84-4 shows the typical appearance of tissues before and after the application of RFA. In a rodent model of normal lung, the appearance of RFA treatment is that of a central density surrounded by a ground-glass opacity. This corresponds to an area of tissue necrosis centrally with a surrounding zone of ongoing necrosis and tissue damage in the ground glass area.11 In a case report of an ablation followed by resection 3 weeks later, an inner band of thermally coagulated blood, chronic hemostasis, and thrombosis with a focal area of lytic necrosis was seen. Surrounding this was an outer band of hemorrhage associated with lytic necrosis of lung tissue.12 Initially, the ablation area will increase in size, but then start to condense. A baseline CT scan is advised at 3 months. Following this, the tumor should progressively shrink.13 Cavitation of the ablated zone can occur in about one-third of all treatments.14 To detect recurrent or persistent disease, the ablation area must be evaluated for any increase in size. This can lead to some delay. Some have demonstrated that it takes about 7 months to detect an incomplete treatment.15
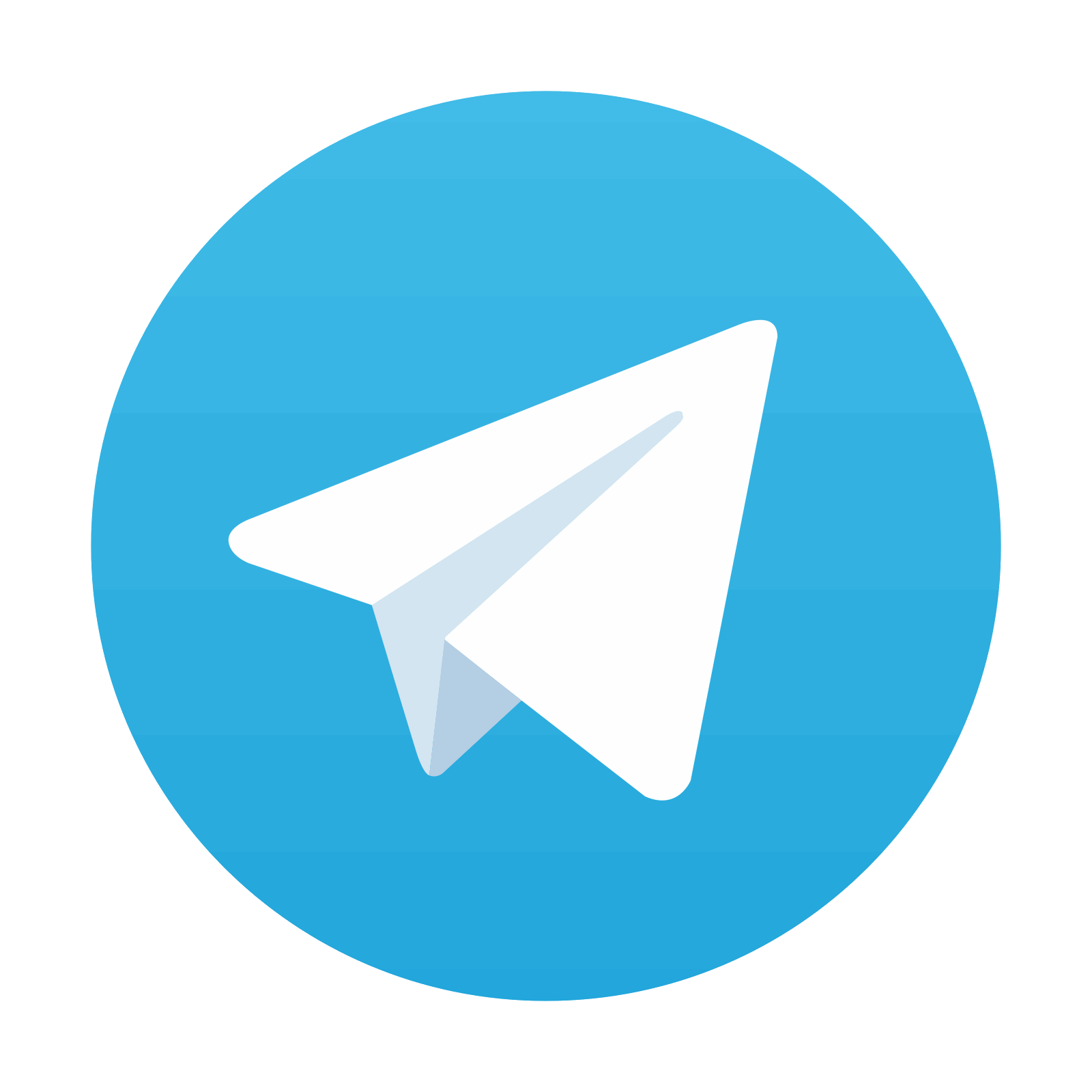
Stay updated, free articles. Join our Telegram channel

Full access? Get Clinical Tree
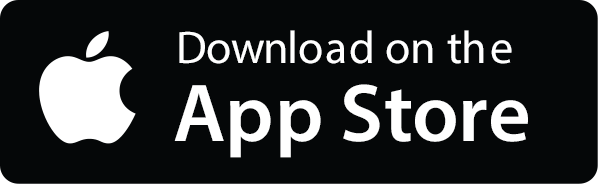
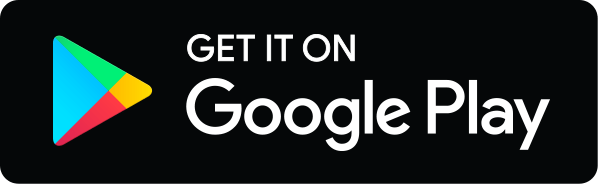