Fig. 46.1
The effect of rising left atrial pressure on the development of pulmonary edema in a canine model (Reproduced from Guyton and Lindsey [1]. With kind permission of Wolters Kluwer Health publishers)
The presence of excess fluid in the alveolar spaces has several effects. It acts as a barrier to gas exchange both between the air and the alveolar membrane and within the edematous membrane cells. It also dilutes the surfactant within the alveolus with the result that surface tension is increased and greater force is required to inspire. The alveoli are thus predisposed to collapse, which further increases the work of inspiration according to Laplace’s law (in which the pressure required to inflate a sphere is inversely related to the diameter). Areas of edema in the lungs cause shunting of blood and hypoxemia, which is partially counteracted by relative vasoconstriction in the hypoxic areas. Increased capillary pressure in the lung ultimately leads to rising pulmonary artery pressure and can subsequently cause failure of the right ventricle. This pathophysiological sequence can lead to respiratory failure – impaired gas exchange resulting in hypoxemia (usually defined as PaO2 <8 kPa) – with or without hypercapnia.
46.3 ACPE: The Role of NIV
46.3.1 Continuous Positive Airway Pressure
Continuous positive airway pressure (CPAP) is the simplest form of respiratory support and has the greatest evidence base in the treatment of ACPE. CPAP provides constant pressure, typically 5–10 cmH2O, via an airtight facemask, nasal mask, or helmet throughout the respiratory cycle. The resultant positive end-expiratory pressure (PEEP) helps prevent alveolar collapse and recruits alveoli that have collapsed. The alveoli are thus maintained at a greater diameter, lung compliance is increased, and the work of breathing is reduced. Lesser shunting of blood improves oxygenation and the positive intrathoracic pressure reduces venous return, thus reducing cardiac preload. LV transmural pressure is also reduced, with an effective reduction in afterload.
The earliest studies of NIV in ACPE compared CPAP plus optimal medical therapy (OMT) with OMT alone [2–4]. CPAP was administered in the emergency room and/or intensive care unit until respiratory failure resolved, intubation was required, or the patient was unable to tolerate CPAP further. The weight of evidence demonstrated an advantage of CPAP over OMT in terms of both in-hospital mortality and the need for endotracheal intubation. CPAP plus OMT also resulted in more rapid improvement in oxygenation and respiratory rate and was safe and effective in elderly patients. A further study revealed a similar benefit of CPAP for HF with both HFREF and HFPEF [5]. The studies did not, however, demonstrate a survival advantage for CPAP over OMT at 30-day follow up.
Two larger recent studies have shed some doubt on the benefit of a routine strategy of NIV in ACPE. The 3CPO trial is the largest multicenter randomized controlled trial (1,069 patients) comparing CPAP or bi-level positive airway pressure (BiPAP) plus OMT against OMT alone [6]. Although there was a more rapid reduction in respiratory rate, heart rate, hypercapnia, and acidosis in the NIV groups, there was no benefit for NIV at 7 days for the combined endpoint of intubation or mortality (9.5 % vs 9.8 %, p = 0.87), and no mortality benefit at 30 days (15.2 % vs 16.4 %, p = 0.64). There was, however, a high frequency of crossover from OMT to NIV on clinical grounds (16 % of OMT patients; presumably those with the most severe respiratory distress) and 9 % of NIV patients crossed into the OMT arm, many of whom are likely to have had less severe respiratory compromise. The mean duration of NIV therapy of approximately 2 h is also shorter than several previous studies. A further trial randomized 124 patients to receive either CPAP plus OMT or OMT alone for ACPE in the pre-hospital setting [7]. This demonstrated no benefit for CPAP over OMT for the primary combined outcome of respiratory rate (<25/min) and oxygen saturation (>90 %) at 1 h (31.7 % vs 35.5 %, p = 0.65). There was no difference in hospital stay, intubation rates (3.3 % vs 4.8 % p = 0.52), or mortality (10 % vs 11.3 % at 30 days, p = 0.52), although the study was not powered for these outcomes.
A meta-analysis of the randomized trials, including the “negative” studies above, concluded there was an overall relative in-hospital mortality advantage for CPAP over OMT of 28 % and a relative reduction in intubation of 45 % [8]. There was no reduction in length of hospital stay.
46.3.2 BiPAP
BiPAP provides background positive pressure in the same manner as CPAP but also gives additional pressure during inspiration (inspiratory positive airway pressure). This reduces the effort required of the inspiratory muscles and may improve ventilation, with particular benefit for patients with hypercapnia and reduced respiratory drive or capability.
The evidence base for BiPAP in ACPE is more limited than for CPAP. Trials show significant heterogeneity, but overall there appears to be a more rapid resolution of hypercapnia, respiratory rate, and hypoxemia with BiPAP than with OMT. Some (but not all) studies showed reduced intubation rates with BiPAP, but a mortality advantage has not been demonstrated. This may be due to small studies in heterogeneous populations [6, 9].
Studies comparing BiPAP to CPAP have produced inconsistent results [9]. Overall, CPAP and BiPAP produce a similar advantage in terms of improved physiological parameters over OMT, but only CPAP has evidence (albeit variable) for improved short-term survival. Improvement in hypercapnia appears to be similar for CPAP and BiPAP.
Early studies found a higher incidence of myocardial infarction in those treated with BiPAP, but subsequent research with adequate power has repudiated this [10].
In practice, CPAP is a simpler and more extensively studied form of respiratory support for ACPE and should be considered for those with persistent respiratory failure not responding to optimal medical therapy. BiPAP should be reserved for those with hypercapnia unresponsive to CPAP, or with underlying lung disease that predisposes to hypercapnia with CPAP (such as advanced chronic obstructive pulmonary disease or obesity hypoventilation syndrome, which may be associated with HFPEF). The evidence does not support the routine use of NIV for all patents presenting with ACPE, and an individualized approach based on clinical findings and arterial blood gas analysis is recommended in European HF guidelines [11].
46.4 SDB in Chronic HF: Epidemiology and Pathophysiology
Around 50 % of patients with HF suffer from SDB. This may comprise obstructive sleep apnea (OSA) or central sleep apnea (CSA), although many patients have a mixed pattern that may change over the sleep period. In OSA, there is loss of pharyngeal muscle tone leading to upper airway collapse and obstruction. This is often associated with obesity and retrognathism, and in HF rostral shift of fluid during sleep leads to edema of the pharynx, exacerbating the tendency to airway collapse. In CSA, there is an abnormality of the regulation of breathing in the respiratory centers of the brainstem. The pathophysiology in HF involves reflex hyperventilation due to activation of pulmonary J receptors by edema and pulmonary congestion, a delayed circulation time between the alveoli and brainstem, and raised chemosensitivity leading to relative hyperventilation in response to rises in arterial carbon dioxide (PaCO2). Hyperventilation leads to falls in PaCO2 to below the apneic threshold, at which point the neural drive to breathe is inadequate to stimulate respiration. The resultant hypopnea or apnea causes the PaCO2 to rise until hyperventilation again occurs and the cycle is repeated. Recurrent cyclical CSA is termed Cheyne-Stokes respiration.
Both forms of SDB are associated with recurrent arousals from sleep, episodes of hypoxemia, and enhanced sympathetic nervous system stimulation with increased catecholamine release. This predisposes to tachycardia, arrhythmia, and – particularly in the case of OSA – hypertension, which may increase the risk of stroke and myocardial infarction [12]. There is an excess of arrhythmia at night in those with OSA and increased rate of therapies from implantable cardioverter-defibrillators [13]. Daytime somnolence is a particular problem for those with OSA, and sufferers are at increased risk of road traffic accidents.
In OSA, the negative intrathoracic pressure generated by respiratory muscles attempting to inspire against a collapsed pharynx increases venous return to the heart and causes a high transcardiac gradient, increasing afterload. The septum shifts leftward and output from the failing LV is compromised. Abnormalities of endothelial function are also found in patients with OSA, including a high expression of the vasoconstrictor endothelin-1 and a blunted response to cholinergic vasodilators [14]. There is an increase in inflammatory markers such as C-reactive protein (known to be associated with vascular events) and prothrombotic factors (leading to enhanced platelet aggregation) [15].
The prevalence of CSA increases with the severity of HF and is a marker of poor prognosis. In a study of patients with moderate to severe LV dysfunction, median survival amongst those with CSA was 45 months versus 90 months for those without – a difference that persisted even when adjusted for several confounding factors [16]. Although this demonstrates association rather than causation, the recurrent hypoxemia, sympathetic stimulation, and changes in pre- and afterload associated with apnea are presumed to accelerate the vicious cycle of HF. Other risk factors associated with the development of CSA include male sex, atrial fibrillation, resting hypocapnia, and age over 60 years.
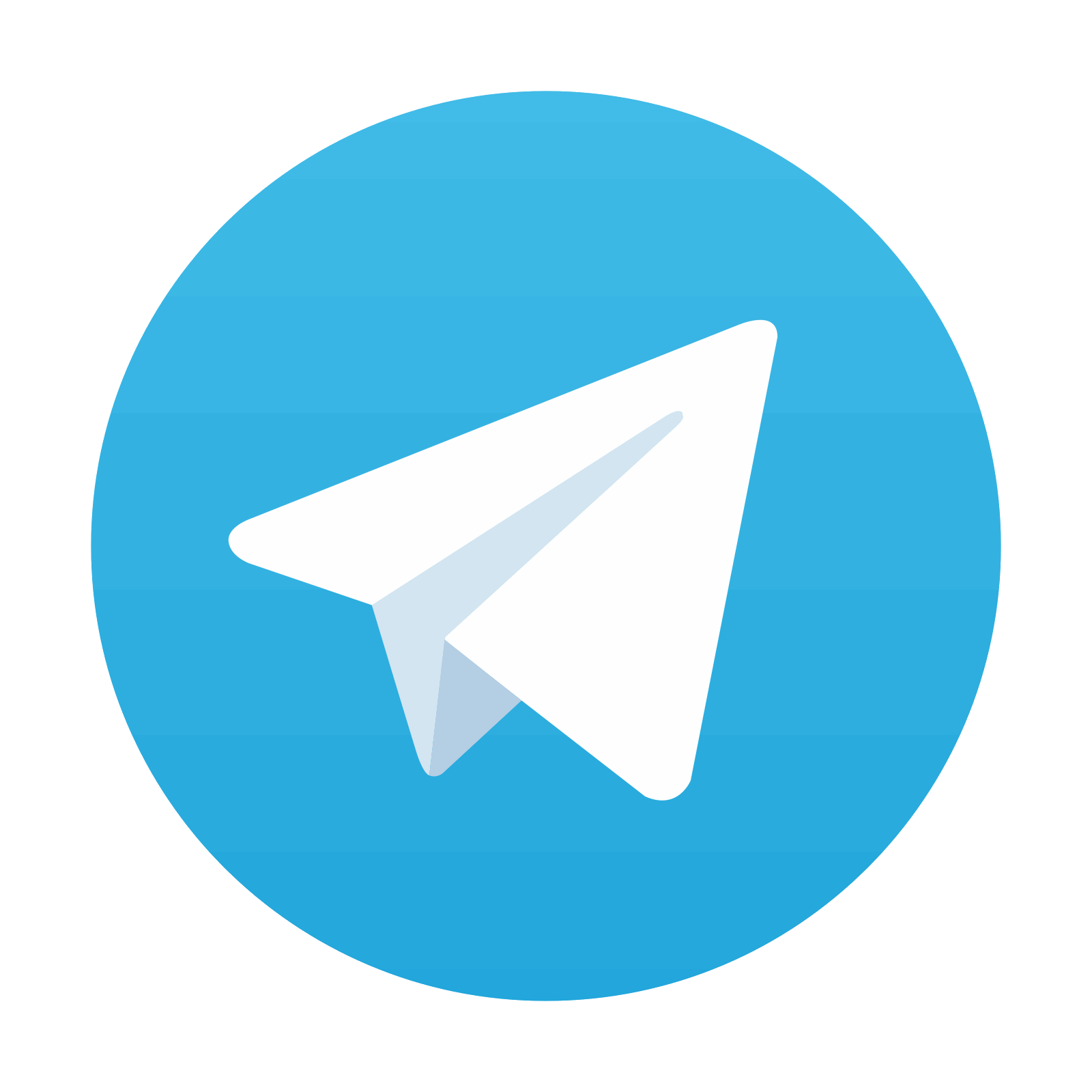
Stay updated, free articles. Join our Telegram channel

Full access? Get Clinical Tree
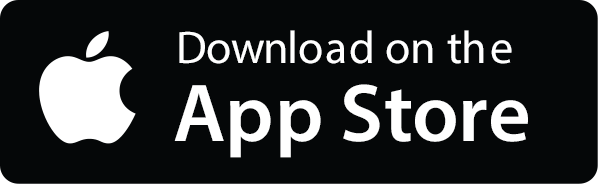
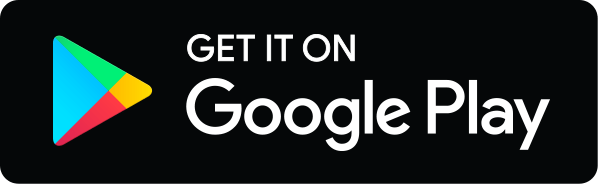