Tracer
Biologic target
Blood flow
13N-ammonia
Perfusion
82Rb
Perfusion
15O-water
Perfusion
Metabolism
18F-FDG
Glucose transport, glucose metabolism
11C-palmitate
Fatty acid metabolism
11C-acetate
Oxidative metabolism (TCA cycle turnover)
Innervation
11C-hydroxyephedrine
Presynaptic catecholamine uptake
11C-epinephrine
Presynaptic catecholamine uptake and storage
Receptors
11C-CGP12177
Beta-adrenergic receptor (nonselective)
11C-MQNB
Muscarinic receptor
22.2 Non-invasive Imaging Techniques
22.2.1 Nuclear Imaging
Nuclear imaging is based on the injection of radionuclides in their native form or as a part of a chemical molecule following the tracer principle, which basically means that very minute amounts of active substances are used without disturbing vital biological processes. Thus, the body is imaged “from the inside out” using special devices which record the distribution of radioactivity from the intravenously administered radionuclides. Currently, PET and SPECT are the most translatable noninvasive molecular imaging platforms. The strengths of nuclear imaging are its high sensitivity, the continuously increasing number of clinical scanners installed and availability of a wide variety of targeted, radioactively labeled tracers in experimental and clinical applications.
22.2.1.1 PET/MicroPET Imaging
PET uses short-lived positron emitters such as oxygen-15 (15O, T1/2 = 2.1 min), nitrogen-13 (13N, T1/2 = 10 min), rubidium-82 (82Rb, T1/2 = 78 s), carbon-11 (11C, T1/2 = 20 min), gallium-68 (68Ga, T1/2 = 68 min) and fluorine-18 (18F, T1/2 = 110 min), for labelling of biomolecules in order to characterize in-vivo physiologic and biochemical processes. 15O, 13N, 11C and 18F are cyclotron produced while 82Rb is produced from a strontium-82 (82Sr) generator system and 68Ga is produced from a Germanium-68 (68Ge) generator system. The emitted positron travels a short distance in the order of millimeters (determined by its initial kinetic energy), before it meets an electron and undergoes the annihilation reaction. Upon annihilation, the mass of positron and electron is converted into two 511 keV photons that travel in approximately opposite directions. Coincidence detection of these annihilation photons followed by mathematical reconstruction of the images form the basis of PET imaging.
The advantages of PET imaging include high sensitivity and temporal resolution enabling dynamic imaging, use of radiolabeled probes with natural radioisotopes such as 11C, that do not alter chemical behavior and absolute quantification of biological processes. List mode data acquisition provides an opportunity to correct for motion during cardiac and respiratory cycles [2]. The inherent resolution of PET system is technically limited by the size of the detection crystals and fundamentally limited by physical behavior of positron decay, associated with the significant movement of positron before annihilation, and deviation from exact 180° angular separation [3]. Current state-of-the-art clinical PET scanners have a spatial resolution of 3–5 mm. A major limitation for the widespread use of PET remains the high cost, reflecting the sophisticated imaging technology and the need of a cyclotron on-site, or in close proximity of production sites for short-lived tracers and availability of expensive generators. The development of fluorinated (18F) tracers, which have a relatively long half-life, allows imaging to be performed even without access to an on-site-cyclotron.
For pre-clinical PET imaging, dedicated systems with small crystals and bore diameters between 10 and 20 cm are required to reach a reasonable spatial resolution. The first microPET scanner was developed at UCLA in 1996 and currently more than 80 preclinical PET systems are installed worldwide. Most animal PET and PET/CT scanners have a spatial resolution of 1–2 mm [1, 4].
Radiopharmaceuticals which have been applied for PET cardiac imaging in the clinical and preclinical setting are described below. In general, 18F-labeled tracers are more likely to achieve broader clinical application from a logistical standpoint, while the clinical use of 11C, 13N, 15O labeled tracers has been limited by the need of an on-site cyclotron.
Myocardial Perfusion Agents
A number of positron emitting radiopharmaceuticals can be used as tracers of myocardial perfusion. The most common are Nitrogen-13 ammonia (13N-ammonia), Rubidium-82 (82Rb), and Oxygen-15 labeled water (15O-water). 13N-ammonia enters the myocardial cells in proportion to blood flow by diffusion across the sarcolemma, followed by metabolic conversion to 13N-glutamine by glutamine synthetase, becoming trapped within the cell. 82Rb is potassium analog, actively taken up by myocytes in proportion to blood flow via the Na+/K+-ATPase. 15O-water is a freely diffusible tracer and has been primarily utilized for absolute flow quantification research, but myocardial images are only obtained after application of kinetic modelling techniques (Table 22.1). At present, 13N-ammonia and 82Rb are the only Food and Drug Administration (FDA) approved PET tracers for the evaluation of individuals with suspected or known coronary artery disease (CAD). Myocardial perfusion PET imaging is accomplished using flow tracers 13N-ammonia and 82Rb at rest and during pharmacologic stress. For assessment of myocardial perfusion, PET is an excellent technique with sensitivity and specificity of about 90 % [5].18F-flurpiridaz is a newly developed PET myocardial perfusion agent targeted to Mitochondrial Complex –I (MC-I) in the heart [6]. It has improved biological and imaging characteristics compared with currently available perfusion imaging agents, including rapid uptake in the myocardium, prolonged retention, and superior extraction versus flow profiles. A first in man study has established the safety and dosimetry of 18F-flurpiridaz and confirmed high sustained cardiac uptake (Fig. 22.1). Subsequent studies performed in CAD patients established the dose and timing needed to detect perfusion deficits when the agent is administered under resting and stress conditions [7].
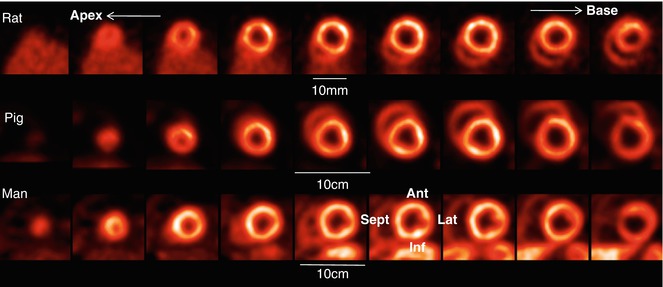
Table 22.2
SPECT radiopharmaceuticals for clinical cardiac imaging
Tracer | Biologic target |
---|---|
Blood flow | |
201Tl | Perfusion, viability |
99mTc-sestamibi | Perfusion, viability |
99mTc-tetrofosmin | Perfusion, viability |
Metabolism | |
123I- BMIPP | Fatty acid metabolism |
Innervation | |
123I-MIBG | Presynaptic catecholamine uptake |
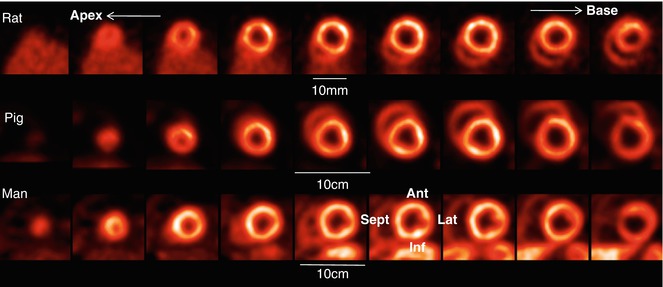
Fig. 22.1
Cardiac PET imaging of myocardial perfusion using F-18 labeled Flurpiridaz in rat, pig and man. The series of short axis images from apex to base were acquired in a dedicated animal PET/CT (rat) and a clinical PET/CT tomograph respectively. Ant anterior segment, Lat lateral segment, Inf inferior segment, Sept septum
Myocardial Metabolism Agents
The primary tracer to image glucose metabolism is fluoro-deoxyglucose (FDG) labelled with fluorine-18 (18F-FDG) (Table 22.1).18F-FDG is a glucose analog taken up by myocardial cells via two carriers, the glucose transporters 1 and 4 (GLUT1 and GLUT 4). Similar to glucose, FDG is then phosphorylated by hexokinase to 18F-FDG-6-phosphate but unlike glucose-6-phosphate, subsequent metabolism of 18F-FDG-6-phosphate is minimal so that 18F-FDG becomes essentially trapped in the myocardium. 18F-FDG PET has been extensively used to assess myocardial viability and is still considered by some investigators as the gold standard for this assessment [8]. FDG is not a specific tracer, as it is also taken by activated macrophages and can be used to image inflammation.
Two tracers, palmitic acid and acetate have been labeled with 11C. 11C-palmitic acid can assess uptake and metabolism of long-chain fatty acids (FA). Their oxidation represents the major source of cardiac energy but complex kinetics and back-diffusion of the unmetabolized tracer by the myocytes allows only semi-quantitative measurements. 11C-acetate, is a two-carbon-chain free FA which is extracted proportionally to myocardial blood flow, then rapidly enters the tricarboxylic acid cycle and is metabolized to carbon dioxide. It is considered a marker of overall oxidative metabolism without the complexity of substrate interaction between glucose and FA [9] (Table 22.1).
Myocardial Innervation Agents
The main tracers to image myocardial innervation are hydroxyephedrine (HED) and epinephrine (EPI) labeled with 11C. HED is a false transmitter analog of norepinephrine and highly resistant to intraneuronal metabolic degradation. HED is considered a marker of uptake-1 mechanism, which is the reuptake for storage or catabolic disposal of norepinephrine into the presynaptic terminal by an energy dependent transporter. EPI is a true catecholamine that, in contrast to HED, is sensitive to metabolic degradation under normal conditions and has also gained interest as a potential neuronal imaging tracer (Fig. 22.2). 11C-EPI labeled compounds are not FDA approved, and it is unlikely that they will not receive a broader clinical application due to their short half-life and need for an on-site cyclotron. New innervation tracers, such as LMI1195, a benzylguanidine derivative similar to metaiodobenzylguanidine (MIBG), but labeled with 18F, are under investigation with the potential for a more widespread use from a logistical standpoint [10]. Post-synaptic receptor density can be assessed with a radiolabeled b-receptor antagonist CGP12177 and MQNB, a muscarinic receptor agonist, both labeled with 11C (Table 22.1).
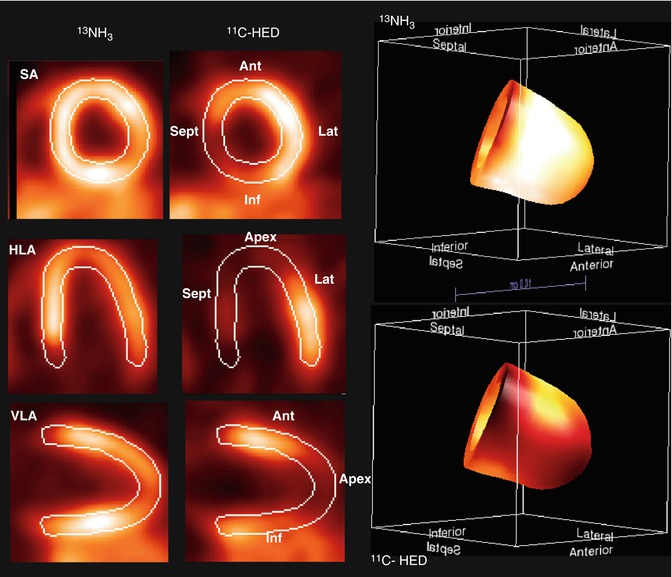
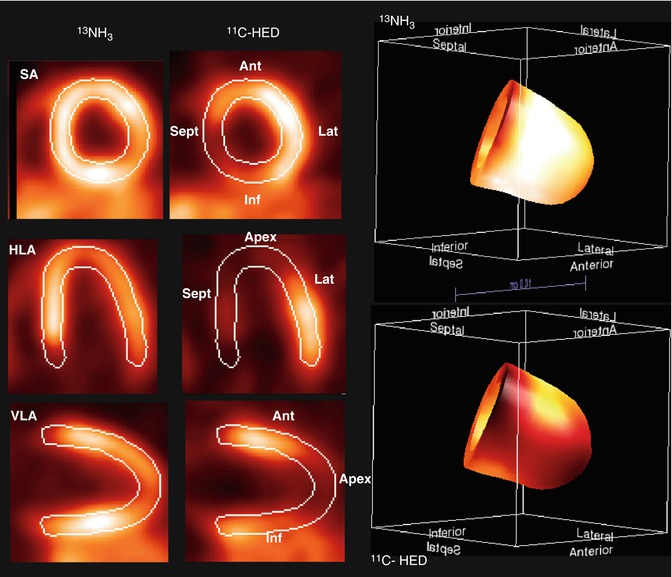
Fig. 22.2
Example of innervation imaging (performed with 11C-HED) with demonstration of its relation to perfusion in a patient imaged after revascularization post MI. There is reduced 11C-HED uptake in the anteroapical region as well as in the septum and most of the inferior wall indicating denervation in these areas. Perfusion as depicted by NH3 uptake is normal in those areas and the rest of the LV myocardium. SA short axis, VLA vertical long axis, HLA horizontal axis, Ant anterior segment, Lat lateral segment, Inf inferior segment, Sept septum
Further Molecular Probes
Beyond the tracers described above, a number of other agents have been tested mainly at a preclinical level and demonstrate potential for clinical translation. 18F-galacto–arginine-glycine-aspartate (RGD) binds to αvβ3integrin receptors that are expressed on the endothelial surface during angiogenesis. Another option for angiogenesis imaging is to use the recently developed 68Ga labelled RGD agent (68Ga-NOTA-RGD) [11]. 68Ga labeled tracers are highly appealing for imaging particularly for centers with no direct access to cyclotron, because 68Ga is produced from a commercially available 68Ge generator system. Annexin-V, a molecule which specifically binds to phosphatidylserine that is expressed on the surface of cells entering the apoptotic cascade, has been extensively tested in preclinical studies to image apoptosis. Radiolabeling of annexin-V for PET imaging has also been performed. Because annexin-V harbors numerous functional groups requiring protection and deprotection steps, its direct radiolabeling with 18F is impractical. Recently, a set of novel small-molecule probes known as the Aposense compounds (a patented platform technology; Aposense Ltd.) has been designed to detect apoptotic membrane imprint [12]. Within this family, the PET tracer 18F-ML-10 shows selective uptake by apoptotic cells, in correlation with the apoptotic hallmarks of breakdown of mitochondrial membrane potential, caspase activation, or apoptotic DNA fragmentation. Signal is lost on membrane rupture, and thus 18F-ML-10 is capable of distinguishing between apoptotic and necrotic cells [12]. 18F-ML-10 is the first PET tracer for apoptosis that has been advanced into clinical trials, with promising results to date in biodistribution and dosimetry profiles. For imaging necrosis, the tracer hypericin labeled with Iodine-124 (124I), has shown a specific and high affinity, although the exact mechanism of action is still unknown [13]. The 11C-labelled PET tracer PK11195, is a selective ligand of the translocator protein, known as peripheral benzodiazepine receptor (PBR) that is expressed in high density in circulating human phagocyte populations, particularly in monocytes and neutrophils [14]. Matrix metalloproteinase (MMP) imaging is also feasible through a newly developed MMP inhibitor labeled with 18F [15]. Other probes targeting adhesion molecules (such as VCAM-1) labeled with 18F are under investigation in experimental models. Recently, 18F-sodium fluoride (18F-NaF), a radiopharmaceutical used for skeletal imaging, was tested in patients with coronary atherosclerosis and symptomatic carotid disease and proved to provide relevant information regarding active micro-deposition of calcium in atherosclerotic plaques, a process that is associated with plaque instability [16].
Several angiotensin converting enzyme (ACE) inhibitors and angiotensin receptor 1 (AT1R) antagonists have been radiolabeled for molecular imaging techniques. Initial attempts for developing specific ACE binding radiotracers were made by use of fluorine-18 labeled captopril (18F–CAP), the first clinically available ACE inhibitor. 18F–CAP, however, had a number of shortcomings that reduced its potential as a suitable tracer for examining ACE distribution. A new radiotracer, N-succinimidyl-4-[F-18]-fluorobenzoyllisinopril (18F–FBL), was synthetized to bind more specifically to ACE. Multiple PET radiotracers for AT1R have been developed labeled with 11C, but major limitations in pharmakokinetics and biodistribution, rendered these tracers unsuitable for clinical use. Another probe labeled with 11C (11C-KR31173) was found to bind selectively to AT1R in various tissues including the heart and first experimental studies in animals and first-in-man application (in four healthy volunteers) proved the feasibility and safety of imaging cardiac AT1R expression [17]. These results provide a rationale for broader clinical testing of AT1R -targeted molecular imaging.
22.2.1.2 SPECT/MicroSPECT Imaging
The detection system in conventional nuclear medicine is called Anger or γ-camera and typically has a sodium iodide (NaI) crystal. This camera permits planar and tomographic cardiac imaging (SPECT), if projections from different angles around the body are acquired. A new generation of cameras was recently introduced and uses cadmium zinc telluride (CZT) crystals. These systems show improved sensitivity and spatial resolution which allows for shorter acquisition times or reduction of injected tracer [18]. SPECT imaging probes are labeled with γ-emitting radionuclides, such as technetium-99 m (99mTc, T1/2 = 6 h), thallium-201 (201Tl, T1/2 = 73 h), indium-111 (111In, T1/2 = 67 h), iodine-123 (123I, T1/2 = 13.2 h), and iodine-131 (131I, T1/2 = 8 days). Among these, 99mTc labeled tracers are the most readily available in everyday clinical practice from a commercially available molybdenum generator system, while the rest, are cyclotron produced. 99mTc has favorable physical and dosimetric features (it decays by isomeric transition and emits gamma rays at an energy level of 140 keV) and is the preferred isotope for SPECT imaging. The spatial resolution of clinical SPECT systems is 7–15 mm [1].
For pre-clinical research, a number of microSPECT systems are available commercially. MicroSPECT imaging offers several advantages in small animal models of CVD, such as the greater availability of radiotracers with a longer half-life compared to PET and the ability to image multiple tracers labeled with different isotopes simultaneously. The spatial resolution of microSPECT systems is 0.5–2 mm [1].
Currently available SPECT tracers used for myocardial perfusion imaging (MPI) are divided into two groups: 201Tl and 99mTc labeled agents (Table 22.2). 201Tl, an analog of potassium, is extracted actively by cell membrane Na+/K+ pumps on the first pass through the coronary vasculature and its uptake is proportional to regional perfusion over a wide range of flow rates. It has the highest myocardial extraction (85 % at the first passage of tracer from the myocytes) of the three available γ-emitting myocardial perfusion radiopharmaceuticals, with a peak myocardial concentration at 10 min after injection. 201Tl is not sequestered permanently in the myocardial tissue, and it is continuously exchanged between the extracellular and intracellular spaces. 201Tl decays by electron capture to mercury-201, and emits lower energy x-rays (75–80 keV) [19]. In contrast to 201Tl, 99mTc must be bound to another compound so that can become suitable for assessing myocardial perfusion. 99mTc-sestamibi (methoxyisobutylisonitrile) and 99mTc tetrofosmin (1,2-bis[bis(2-ethoxyethyl)phosphino] ethane) are the two commercially available tracers. Both agents are lipophilic cations that passively diffuse into cardiomyocytes along an electropotential gradient and are actively trapped in mitochondria or in cytoplasma. The extraction of sestamibi and tetrofosmin by the myocytes is considerably lower than that of 201Tl (65 and 54 % respectively). The intracellular passage of sestamibi and tetrofosmin is permanent, and redistribution is negligible, particularly with the tetrofosmin [19]. Myocardial uptake is proportional to myocardial blood flow in a specific range of flow rates, but lower than that of 201Tl. The relationship between myocardial blood flow (MBF) and net myocardial uptake of various perfusion tracers (15O-water, 13N-ammonia, 82Rb, 201Tl, 99mTc-sestamibi and 99mTc tetrofosmin) is presented in Fig. 22.3.
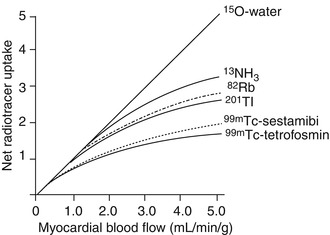
Table 22.3
Potential benefits of hybrid PET/MRI in cardiac imaging
Application | PET | MRI | PET/MRI |
---|---|---|---|
Myocardial perfusion imaging | Allows quantification of myocardial blood flow; accurate method to detect functionally significant coronary lesions. | A potential alternative for diagnosis of CAD and assessment of atheromatous plaques’ morphology (in experienced centres) | MRI-based correction for attenuation, motion, and partial volume correction for improved quantification of myocardial blood flow? |
Non-invasive coronary angiography | – | Presently inferior performance, but no radiation or contrast agents as in coronary CT angiography | Possibility to combine anatomy (plaque burden, luminal obstruction) with haemodynamic consequences (ischaemia) of CAD |
Assessment of left ventricle function | Limited by low spatial resolution | Most accurate technique to determine left ventricle function and structure | Combination of left ventricle function with perfusion, metabolic or molecular imaging for improved stratification of heart failure |
Assessment of infarction and viability | 18F-FDG uptake is a gold standard of viability. | High-resolution delineation of infarction by MRI LGE. Potential of magnetic resonance spectroscopy? | More detailed risk assessment by combining glucose uptake and LGE |
Molecular imaging | Excellent sensitivity; tracers for many targets (inflammation, angiogenesis, sympathetic nervous function, therapeutic genes or cells etc.) | Lower sensitivity than PET; evolving tracers and imaging techniques for molecular targets | Exact anatomical localization and volume correction by MRI for detection and quantification of molecular targets by PET |
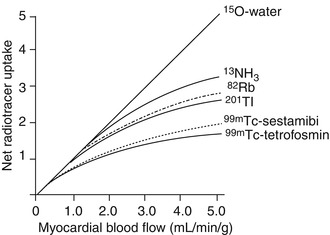
Fig. 22.3
Relation between net myocardial uptake of various perfusion tracers and MBF. With the exception of 15O-water which exhibits a linear uptake-flow relation, the net uptake for all radiotracers increases non-linearly with higher flow rates. Please note that despite this limitation, 13NH3 and 82Rb demonstrate higher myocardial net uptake rates compared to SPECT perfusion tracers at higher flows (Reprinted, with permission from Bravo et al. J Cardiovasc Transl Res. 2011)
SPECT MPI has a history of over 20 years in clinical practice. Such studies can be performed at rest or after physical exercise or pharmacological stress, with vasodilators (dipyridamole, adenosine, or regadenoson) or with the β-agonist dobutamine (Fig. 22.4). Perfusion studies can be coupled with electrocardiogram (ECG) gating (gated SPECT) allowing also assessment of ventricular function.
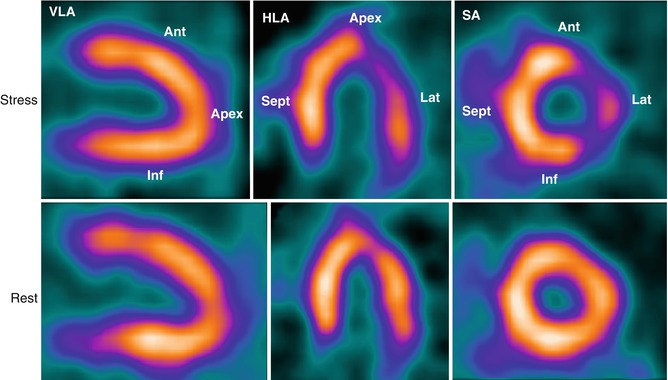
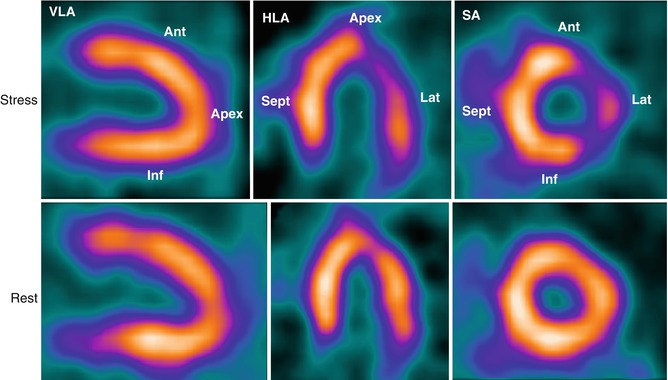
Fig. 22.4
SPECT myocardial perfusion imaging (MPI). Top row (stress study): there is reduced uptake of Thallium-201 in the lateral wall (HLA and SA views) following pharmacological stress with adenosine (140 μg/kg/min administered over a period of 6 min) but normal uptake elsewhere. Bottom row (resting study): the resting study is normal. Taken together, the stress and rest imaging data demonstrate an inducible perfusion abnormality in the lateral wall compatible with disease of the left circumflex artery. SA short axis, VLA vertical long axis, HLA horizontal long axis, Ant anterior segment, Lat lateral segment, Inf inferior segment, Sept septum
Beyond perfusion, other parameters can also be assessed such as FA metabolism and innervation by molecules labelled with 123I (Table 22.2). 123I emits predominantly γ photons with energies of 159 keV. 123-iodinated modified FA such as b-methyliodophenylpentadecanoic acid (BMIPP), have provided a sensitive SPECT marker of altered FA transport into the myocyte [20]. In contrast to straight long-chain FA that enter the mitochondria and are metabolized by b-oxidation immediately, BMIPP is not metabolized via b-oxidation because the methyl substitution precludes the formation of the ketoacyl coenzyme A intermediate. The prolonged retention of BMIPP in the cardiomyocyte is suitable for a longer acquisition time which is necessary for SPECT. Cardiac autonomic innervation imaging is also feasible by the use of a radiolabeled norepinephrine analog 123I-MIBG. 123I-MIBG, although similar to norepinephrine in terms of its storage, release, and reuptake in the presynaptic nerve terminal, it neither binds to the postsynaptic receptors nor undergoes presynaptic or postsynaptic metabolism. 123I-MIBG undergoes avid uptake and storage in the cardiac nerve terminals through norepinephrine transporter-1. Myocardial retention of 123I-MIBG depends mainly on the integrity of the presynaptic nerve terminal [21]. Imaging with 123I-MIBG is in clinical use for many years. Semiquantitative measurement of 123I-MIBG uptake is performed with early (10–20 min) or late (3–4 h) heart-to-mediastinum ratio (H/M) or washout rate of this tracer [22].
< div class='tao-gold-member'>
Only gold members can continue reading. Log In or Register a > to continue
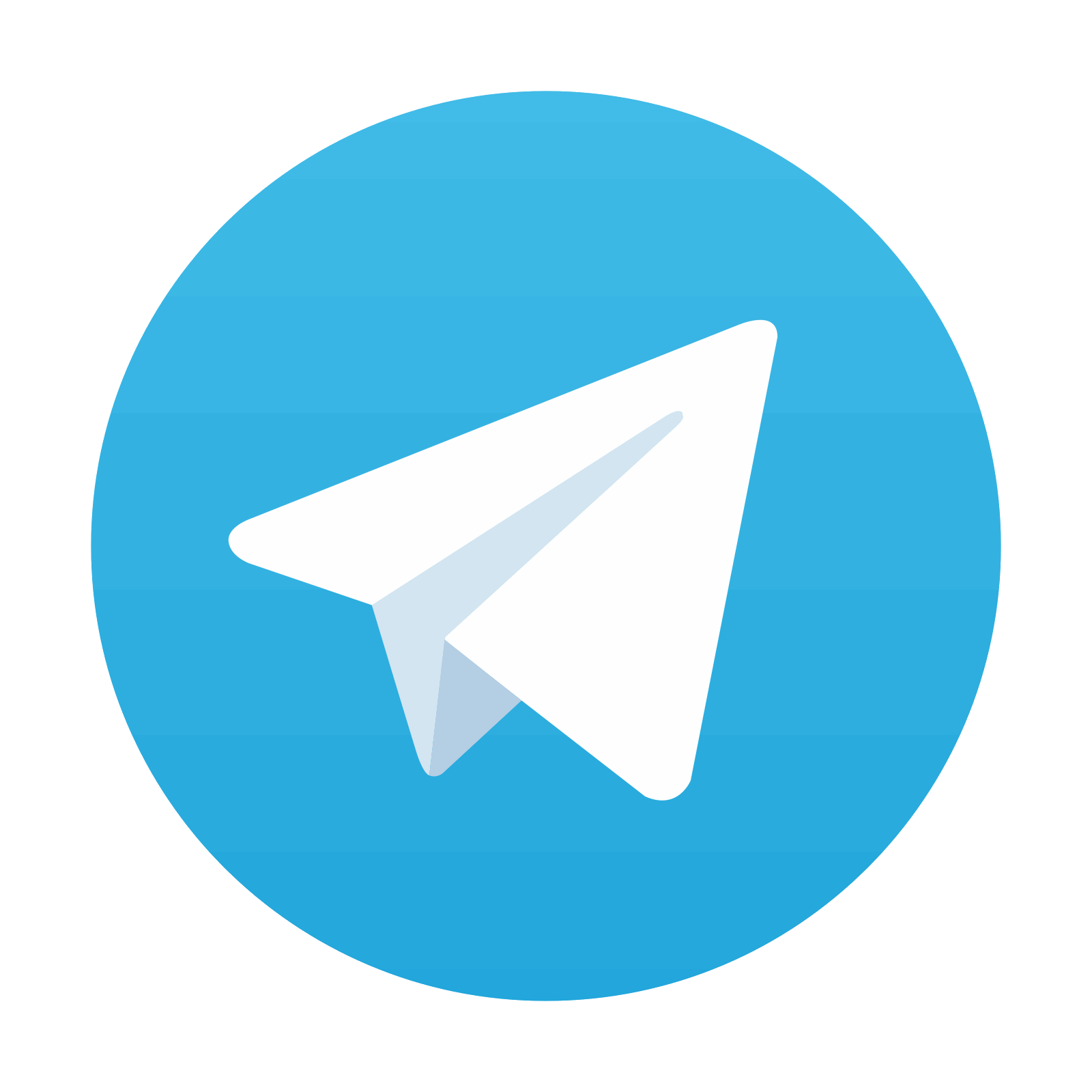
Stay updated, free articles. Join our Telegram channel

Full access? Get Clinical Tree
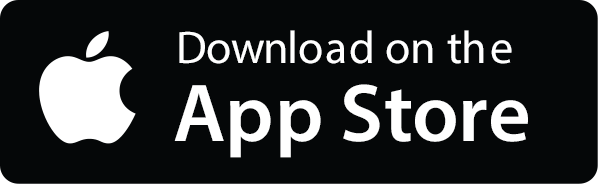
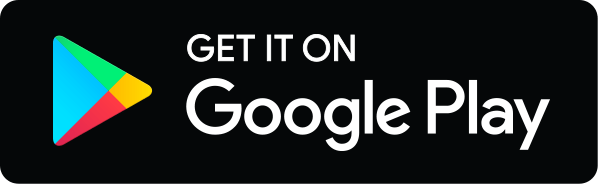