Classic genomic action of thyroid hormones
The thyroid hormones triiodothyronine and thyroxine act on almost every cell and organ in the body, with intense effects on the cardiovascular system. The heart is a major target organ for thyroid hormone action and marked changes occur in cardiac function in patients with hypothyroidism or hyperthyroidism. Although thyroxine is the major thyroid secretion product, triiodothyronine is responsible for most of the biological effects ascribed to thyroid hormones, including the negative-feedback system for secretion of thyrotropin-releasing hormone and thyroid-stimulating hormone by the hypothalamus and pituitary, respectively. Among the different tissues, triiodothyronine has pivotal effects on the cardiovascular system, acting as an important regulator of cardiovascular haemodynamics through its direct action on cardiac myocytes, vascular smooth muscle cells (VSMCs) and endothelium . Thyroid disease is quite common. Current estimates suggest that it affects as many as 9 to 15% of the adult female population and a smaller percentage of adult men. This sex-specific prevalence almost certainly results from the autoimmune mechanism underlying the most common forms of thyroid disease .
The mechanism of action of thyroid hormones has been described as beginning in the cell nucleus and requiring the participation of specific receptor proteins in the nuclear compartment. The classic genomic action of triiodothyronine occurs through its binding to the nuclear thyroid hormone receptor, which binds to thyroid hormone response elements in the promoter regions of target genes, thereby activating or repressing their transcription . The thyroid gland, in response to thyroid-stimulating hormone, synthesizes and releases thyroid hormones (mostly as thyroxine with < 5% as triiodothyronine). The majority of biologically active triiodothyronine derives from the conversion of thyroxine by type I or type II 5′-deiodinase in peripheral tissues. Normally, the heart relies on circulating triiodothyronine because no significant cardiac myocyte intracellular deiodinase activity occurs . Among the different tissues, triiodothyronine has pivotal effects on the heart and cardiovascular system, acting as an important regulator of cardiac function and cardiovascular haemodynamics through its direct action on cardiac myocytes, VSMCs and endothelium . The increase in cardiac output observed in hyperthyroidism results from a combination of increased cardiac function (inotropic and chronotropic effects) and changes in cardiovascular haemodynamics (lowered systemic vascular resistance and increased blood volume), suggesting that reduced vascular resistance could be secondary to alterations in the vascular control mechanisms that favour vasodilatation . The precise mechanism of the decline in systemic vascular resistance in hyperthyroidism is not known and there are some contradictory results relating to this subject. Thyroid hormones affect cardiac myocytes as well as the smooth muscle and endothelial cells of the vascular wall. Relatively little is known about the mechanisms that regulate the interaction of triiodothyronine with endothelial cells.
It has been suggested that hyperthyroidism is similar to a hyperadrenergic state; however, no evidence suggests that triiodothyronine excess enhances the sensitivity of the heart to adrenergic stimulation . Triiodothyronine-mediated inhibition/phosphorylation of phospholamban involves intracellular kinase pathways shared by catecholamine signal transduction; this underlines the similarities between triiodothyronine and catecholamine effects on heart and vessels . The classic genomic actions are dependent on interaction with nuclear receptors to modulate cardiac myocyte gene expression. Triiodothyronine enters the cell membrane by simple diffusion, as described for steroidal hormones, or via specific transport proteins, such as an energy-dependent carrier that partially depends on the sodium gradient. In the nucleus, triiodothyronine interacts with the nuclear thyroid hormone receptor. The mechanism of action of thyroid hormones has been described as starting in the cell nucleus and requiring the participation of specific receptor proteins in the nuclear compartment. However, there is now growing evidence for triiodothyronine- and thyroxine-triggered non-genomic pathways, resulting from their binding to plasma membrane, cytoplasm or mitochondrial receptors, which leads to rapid regulation of cardiac functions. Some actions of thyroid hormones are now assumed to involve extranuclear mechanisms in a variety of cells; these are initiated at the plasma membrane receptor for iodothyronines .
Importance of the non-genomic actions of thyroid hormones
The mechanisms of several of the non-genomic actions of thyroid hormone have now been elucidated, at least in part; they depend upon cellular signal transduction systems and either novel cell surface receptors for thyroid hormone or the extranuclear thyroid hormone receptors TRα and TRβ . The non-genomic actions of triiodothyronine in the myocardium are envisaged to affect the transporter localized at the plasma membrane and the mechanisms associated with nitric oxide (NO) metabolism, through mechanisms that involve distinct signalling cascades. It is assumed that these actions involve extranuclear binding sites in several compartments of the cell, including the plasma membrane, cytoskeleton, cytoplasm and mitochondria. Unlike the nuclear effects, the extranuclear effects are independent of thyroid hormone nuclear receptors, may occur within a short time (seconds to minutes) and may be mediated by signal-transducing pathways. A cell surface receptor for iodothyronines has been described on a structural protein of the plasma membrane; this protein is integrin αVβ3, a heterodimer that interacts with a substantial number of extracellular matrix proteins .
Nitric oxide synthases: targets for the actions of thyroid hormones?
NO can be generated by three different isoforms of the enzyme NO synthase (NOS). The isozymes are referred to as neuronal NOS (nNOS or NOS I), inducible NOS (iNOS or NOS II) and endothelial NOS (eNOS or NOS III). All isoforms of NOS use l-arginine as substrate and molecular oxygen and reduced nicotinamide-adenine-dinucleotide phosphate as co-substrates. Flavin adenine dinucleotide, flavin mononucleotide and (6R)-5,6,7,8-tetrahydro-l-biopterin (BH4) are co-factors of all isozymes . The endothelium plays a crucial role in the regulation of cardiovascular homeostasis, through the release of vasoactive compounds such as NO, prostacyclin and endothelium-derived hyperpolarizing factor. eNOS has been reported to be under the control of caveolin, the structural protein of caveolae. eNOS is reversibly targeted to signal-transducing plasmalemmal caveolae, where the enzyme interacts with a number of regulatory proteins, many of which are modified in cardiovascular disease states . Recently, NO production by VSMCs was identified as an important mechanism for overproduction of NO in the vascular wall and might be of importance for the local control of vascular function .
Substrate and co-factor availability play important roles in regulating NOS activity, not only by limiting enzyme activity but also by influencing the coupling of NOS with its co-factors. Other potential regulators of NOS, such as hormones, may also be important. Thyroid hormone rapidly relaxes VSMCs, but the mechanisms involved in this effect remain unclear. Thyroid hormone-induced rapid vascular relaxation is mediated by VSMC-derived NO production and is associated with the phosphatidylinositol 3-kinase/protein kinase B (PI3K/Akt) signalling pathway. Triiodothyronine significantly increased the expression of three NOS isoforms in endothelial and muscular cells, but only nNOS and iNOS played a significant role in NO production by VSMCs . New data have identified only nNOS and eNOS as targets for the actions of thyroid hormone. There is evidence that triiodothyronine exerts a direct vasodilator effect through different endothelial mechanisms and new studies suggest that the smooth muscle layer also constitutes an important target for the vasorelaxant effects of triiodothyronine. Given that the amount of NO produced by iNOS is greater than that produced by eNOS and nNOS, it is conceivable that iNOS makes a greater contribution than nNOS to triiodothyronine-induced NO production in VSMCs.
In addition, increased vascular NO production has been reported in rats in hyperthyroid states . Although this response has been associated with augmented endothelium-dependent vasodilation, greater nNOS and eNOS expression was found in the VSMCs, suggesting that changes in NO production occur not only in the endothelial cells but also in other layers of the vascular wall, contributing to the augmented vasodilation. After thyroid hormone replacement in patients with subclinical hypothyroidism, a significant improvement in acetylcholine-induced vasodilatation was observed (along with an unchanged response to sodium nitroprusside) .
An interesting study aimed to evaluate plasma small dense low-density lipoprotein (LDL), l-arginine and dimethylarginine concentrations and their relationship to NO production in patients with subclinical hypothyroidism before and after thyroxine replacement therapy and compared with control group values . Among the new biochemical risk factors, asymmetrical dimethyl arginine (ADMA), an endogenous inhibitor of NOS, was defined in cardiovascular risk assessment . ADMA and l-arginine concentrations in patients with subclinical hypothyroidism were significantly higher than in the control group and were not different from the control group after thyroid hormone replacement. Exogenous thyroid hormone appears to benefit endothelial function in patients with subclinical hypothyroidism by lowering blood ADMA concentrations.
Recently, a study investigated the effects of both native and oxidized LDL on triiodothyronine-mediated Akt phosphorylation and NO and cyclic guanosine monophosphate production in human endothelial cells. The results of this study demonstrated that oxidized LDL may contribute to a blunting of the non-genomic action of triiodothyronine and impair the effect of triiodothyronine on NO and cyclic guanosine monophosphate production in endothelial cells. These data suggest that oxidized LDL, apart from inducing the atherosclerotic process, may also promote a mechanism of peripheral resistance to triiodothyronine . It is also important to remember that, in certain conditions, thyroid hormones are able to limit the oxidative modifications of LDL via a free radical scavenging capability . The results of these studies are of potential clinical relevance. Endothelial dysfunction, resulting from a reduction in NO availability and LDL oxidation, is a common event in cardiovascular disease, and thyroid dysfunction is a frequent condition that confers an increased risk of cardiovascular complications.
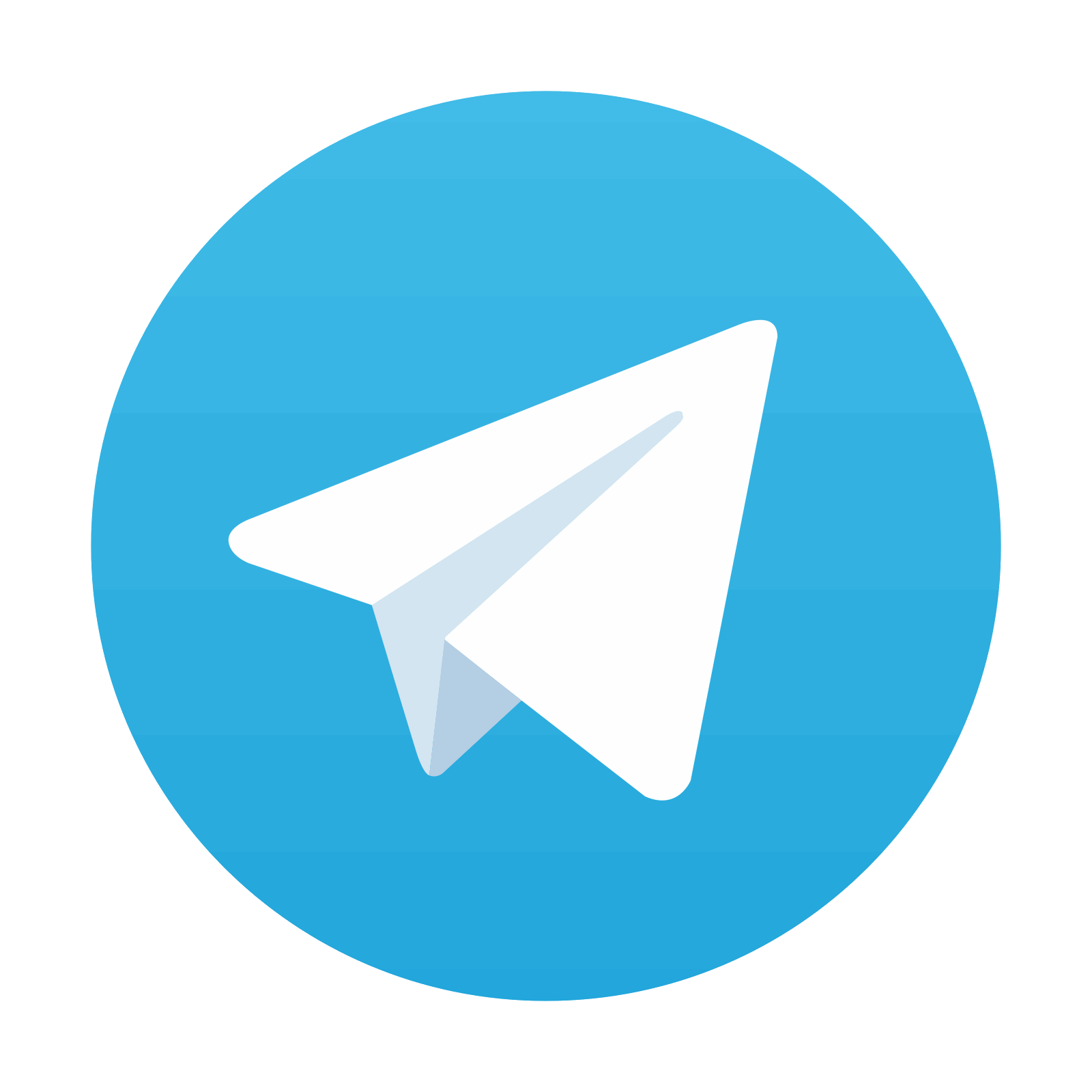
Stay updated, free articles. Join our Telegram channel

Full access? Get Clinical Tree
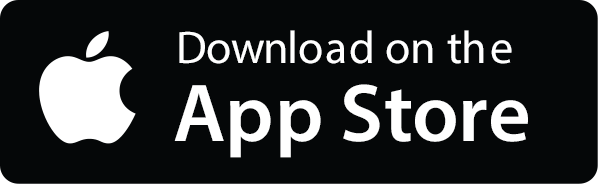
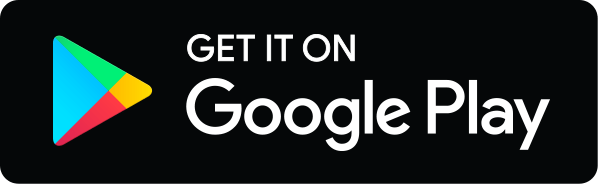