Summary
Heart failure is a highly morbid syndrome generating enormous socio-economic costs. The failing heart is characterized by a state of deficient bioenergetics that is not currently addressed by classical clinical approaches. Nicotinamide adenine dinucleotide (NAD + /NADH) is a major coenzyme for oxidoreduction reactions in energy metabolism; it has recently emerged as a signalling molecule with a broad range of activities, ranging from calcium (Ca 2+ ) signalling (CD38 ectoenzyme) to the epigenetic regulation of gene expression involved in the oxidative stress response, catabolic metabolism and mitochondrial biogenesis (sirtuins, poly[adenosine diphosphate-ribose] polymerases [PARPs]). Here, we review current knowledge regarding alterations to myocardial NAD homeostasis that have been observed in various models of heart failure, and their effect on mitochondrial functions, Ca 2+ , sirtuin and PARP signalling. We highlight the therapeutic approaches that are currently in use or in development, which inhibit or stimulate NAD + -consuming enzymes, and emerging approaches aimed at stimulating NAD biosynthesis in the failing heart.
Résumé
L’insuffisance cardiaque est un syndrome hautement morbide qui génère un coût socio-économique considérable. Le cœur insuffisant est caractérisé par un état de déficit bioénergétique qui n’est pas directement adressé par les thérapies les plus communément utilisées en clinique à l’heure actuelle. Le nicotinamide adénine dinucléotide (NAD + /NADH) est un coenzyme majeur des réactions d’oxydo-réduction du métabolisme énergétique qui a récemment émergé comme une molécule de signalisation avec un large spectre d’action allant de la signalisation Ca 2+ (CD38) à la régulation épigénétique de l’expression des gènes de la biogenèse mitochondriale et du métabolisme catabolique et de la résistance au stress oxydant (sirtuines, PARP). Dans cette revue, nous reprenons l’état des connaissances actuelles sur les altérations de l’homéostasie du NAD qui ont été observées dans différents modèles d’insuffisance cardiaque et leur impact sur les fonctions mitochondriales et les fonctions de signalisation Ca 2+ , sirtuines et PARP. Nous mettons en avant les molécules thérapeutiques déjà utilisées ou en cours de développement qui inhibent ou stimulent les enzymes hydrolysant le NAD + et les approches nouvelles visant à stimuler les voies de biosynthèse du NAD + .
Energy failure in heart failure
The population is ageing worldwide, leading to a higher prevalence of age-related cardiovascular and metabolic diseases, such as hypertension, coronary heart diseases and type 2 diabetes mellitus, all of which raise the risk of developing heart failure. Despite improvements in heart failure therapy in the last two decades, patients still have a poor quality of life, repeated hospitalizations and a reduced life expectancy. The last decade of research has shown that mitochondrial dysfunctions, leading to bioenergetics defects and increased production of reactive oxygen species (ROS), are key players in the process of cardiac ageing and the development of heart failure .
Current therapies for heart failure are mainly based on the reduction of heart rate (beta-blockers) and cardiac workload and remodelling (angiotensin-converting enzyme inhibitors, mineralocorticoid receptor antagonists, vasodilators), which contribute to spare energy consumption, but do not address the issue of deficient energy production in heart failure. The decline in energy production and usage capacity observed in the failing heart is caused by a global alteration in bioenergetics systems, including deficient creatine kinase-mediated energy transfer systems, decreased fatty acid β-oxidation and decreased mitochondrial oxidative phosphorylation capacities . The exact causes of this decline in energy production in the failing heart are far from being completely understood, but are thought to be associated with the repression of important transcriptional regulators of metabolic pathways and mitochondrial biogenesis, including peroxisome proliferator-activated receptor gamma coactivator 1-α and β (PGC1α and β) and oestrogen-related receptor-α and γ . Alteration of calcium (Ca 2+ ) handling systems in failing cardiomyocytes may also affect mitochondrial Ca 2+ load and oxidative phosphorylation capacities. A common endpoint of these metabolic alterations is that the myocardium becomes energy starved, which is reflected in an alteration to the homeostasis of high-energy metabolites: first the major energy reserve compound phosphocreatine declines (reduced phosphocreatine/adenosine triphosphate [ATP] ratio), then the ATP/adenosine diphosphate (ADP) ratio and finally the total ATP concentration are progressively reduced .
Nicotinamide adenine dinucleotide (NAD) at the crossroad between energy metabolism, cell signalling and epigenetics
NAD coenzyme functions
While the alteration in ATP nucleotide homeostasis has been studied extensively in the context of cardiac ageing and heart failure, much less is known about the homeostatic regulation of NAD, despite its major role as a coenzyme of oxidoreduction reactions in energy metabolism. Oxidation of glucose and fatty acids lead to the reduction of oxidized NAD (NAD + ) to NADH. Glycolysis occurring in the cytosol produces two NADH molecules. This cytosolic NADH is converted back to NAD + by the malate dehydrogenase that initiates the transfer of reducing equivalents to the mitochondria though the malate–aspartate shuttle system. On the other hand, fatty acid β-oxidation takes place inside the mitochondria, and generates one flavin adenine dinucleotide-2 (FADH2) molecule, one NADH molecule and one acetyl coenzyme A molecule for each cycle of cleavage of two carbons. For instance, a 16-carbon long palmitate molecule generates seven NADH and FADH2 molecules and eight acetyl coenzyme A molecules. As the Krebs cycle produces three NADH molecules and only one FADH2 molecule per cycle, NADH is the major electron donor to the electron transport chain in the mitochondria. In addition to this major function in energy metabolism, NAD is also the precursor of nicotinamide adenine dinucleotide phosphate (NADP), by means of NAD kinase-mediated phosphorylation or the mitochondrial nicotinamide nucleotide transhydrogenase. NADP in its reduced form (NADPH) is the essential coenzyme of the enzymatic pathways dedicated to the detoxification of ROS, such as the glutathione and thioredoxin reductase systems. In all these functions as a coenzyme for oxidoreductases of the energy metabolism, the recycling of NAD + and NAD does not modify the total pool of NAD. However, several cellular pathways exist that are net consumers of NAD + .
NAD consumption by signalling pathways
Indeed, NAD + has emerged in the recent years as an important signalling molecule that is used by different pathways involved in the regulation of energy metabolism (sirtuins), the response to oxidative stress (poly[ADP-ribose (ADPR)] polymerase [PARP], sirtuins) and Ca 2+ signalling (CD38 ectoenzyme), setting NAD + as a major regulatory hub directly interfacing with energy metabolism and cellular functions ( Fig. 1 ).


NAD + -dependent sirtuin deacetylases
Sirtuins function as metabolic regulators in response to energy stress, through the stimulation of mitochondrial biogenesis and oxidative phosphorylation genes . Sirtuins are enzymes (SIRT1 to 7) that cleave NAD into nicotinamide (NAM) and ADPR moieties ( Fig. 1 A) to perform different types of post-translational modifications on cellular proteins. In one type of reaction, sirtuins remove acetyl (SIRT1, SIRT2, SIRT3, SIRT6, SIRT7), succinyl (SIRT5) or lipid (SIRT6, SIRT7) groups that have been covalently linked to lysine residues on cellular proteins, modifying their charge and activity . In that case, ADPR is used as the acceptor of the removed group. Alternatively, in the mono-ADP-ribosylation reaction performed by some sirtuins (SIRT4, SIRT6, SIRT7), the ADPR moiety is transferred to arginine residues on target proteins, modifying their charges and activities. SIRT1, SIRT6 and SIRT7 are predominantly nuclear, and exert their functions through a direct effect on transcription factors involved in the regulation of metabolism, autophagy and cell survival. SIRT3 and SIRT5 reside in the mitochondrial matrix, and enhance the activity of enzymes involved in the Krebs cycle and oxidative phosphorylation metabolism . One of the most extensively studied sirtuins is SIRT1. SIRT1 plays a role in chromatin remodelling and gene expression by deacetylating histones as well as transcription factors, including forkhead box O (FoxO) factors, p53 and PGC1α, which regulate autophagy, survival and metabolic pathways. SIRT1-mediated deacetylation of the PGC1α transcription factor stimulates its activity and increases mitochondrial biogenesis . Resveratrol, a SIRT1 activator, improves mitochondrial function in cardiac and skeletal muscles . A moderate level of SIRT1 overexpression in the myocardium protects the heart against ageing and oxidative stress , as well as against ischaemia/reperfusion injury . Other sirtuins, including SIRT3, SIRT4, SIRT6 and SIRT7, have also been shown to play a protective role in the heart by targeting mitochondrial or nuclear proteins . Notably, SIRT3 plays an essential role in counteracting inhibitory non-enzymatic acetylation of mitochondrial proteins caused by the excess of free acetyl coenzyme A, observed in the setting of a high-fat diet . SIRT3 overexpression in transgenic mice protects the heart from pressure overload stress . Angiotensin 2 and phenylephrine agonists have been shown to lower NAD + concentrations in the heart, and NAD + administration was able to blunt left ventricular hypertrophy in a SIRT3-dependent manner .
NAD + -dependent ADPRs
NAD + is also used as an ADPR donor by a large family of enzymes known collectively as ADP-ribosyl transferases (ARTD1 to 18 [previously known as the PARPs] and ARTC1 to 5) . PARP1 (ARTD1 in the new nomenclature) is a major NAD-consuming enzyme in the cell, which recognizes DNA lesions induced by an excess of ROS. When activated, PARP1 catalyzes the formation of long polymers of ADPR (parylation) on itself and partner proteins, to recruit the machinery of DNA repair enzymes. Mild activation of PARP1 is protective, but overactivation can deplete the cellular pool of NAD and alter SIRT1 activity, which led to cardiomyocyte death in a mouse model of pressure overload hypertrophy . Interestingly, SIRT1 was shown to deacetylate and repress PARP1 . As both enzymes use NAD + for their catalytic activity, they appear to be involved in competitive pathways to decipher cell fate in a stress situation. PARP1 is also involved in the regulation of energy metabolic pathways, as it was recently shown to bind to peroxisome proliferator-activated receptor-γ nuclear receptor, enhancing ligand binding and co-factor exchange in adipocytes . Other ART enzymes are less well characterized, but the ectoenzyme ART1 (ARTC1 in the new nomenclature), for instance, has been shown to ADP-ribosylate a number of membrane proteins, including integrin α7 in skeletal muscle . Interestingly, this modification was shown to enhance the adhesion of integrin α7 to the laminin protein in the extracellular matrix , which may be of importance in the highly active cardiac muscle.
NAD + -dependent Ca 2+ signalling
A major enzyme involved in NAD hydrolysis is the CD38 ectoenzyme that generates NAM, ADPR and cyclic ADPR (cADPR); ADPR and cADPR act as second messengers in calcium signalling . CD38 can also generate the nicotinic acid adenine dinucleotide phosphate (NAADP) derivative of NADP involved in lysosomal Ca 2+ mobilization . The activity of the ryanodine receptor is stimulated by cADPR in cardiac myocytes , and cADPR increases the frequency of Ca 2+ sparks that are essential for the Ca 2+ -induced Ca 2+ release mechanism at the basis of cardiac rhythmic contractility . The cADPR second messenger is also required for the angiotensin II-induced sustained Ca 2+ rise that is observed after the initial rapid transient Ca 2+ elevation triggered via the inositol trisphosphate receptor . Some authors, however, found that connexin 43 hemichannels could be an alternative route of entry for cADPR , and that NAD + may also enter directly by these channels .
CD38 hydrolysis of NAD + also generates ADPR, which, together with cADPR, is a known activator of the TRPM2 channel, a member of the M-family of transient receptor potential channels that are permeable to Ca 2+ . Interestingly, Ca 2+ entry via TRPM2 is essential for cardiac myocyte bioenergetics maintenance in the context of ischaemia-reperfusion injury or doxorubicin cardiomyopathy , suggesting that TRPM2 is a potential link between NAD + and Ca 2+ signalling for cardiomyocyte survival.
CD38 was once thought to essentially hydrolyze extracellular NAD + , but recent data have shown that the enzyme exists in two conformations, one with the catalytic side on the outer side of the membrane and one with the catalytic side facing the cytosol, hence it is able to hydrolyze the intracellular pool of NAD + .
CD38-deficient mice display a general increase in NAD + tissue concentration, although results discrepancy was notable for the heart between the study of Aksoy et al., which reported a 30-fold increase , and the study of Young et al. , which reported no change. Myocardial contractility, contraction and relaxation velocities are significantly enhanced in male CD38-deficient mice . Altogether, these studies suggest that CD38 is an important regulator of the balance of extra- and intracellular NAD homeostasis, and establish a potential link between NAD + and Ca 2+ signalling, although further research is needed to fully understand this connection.
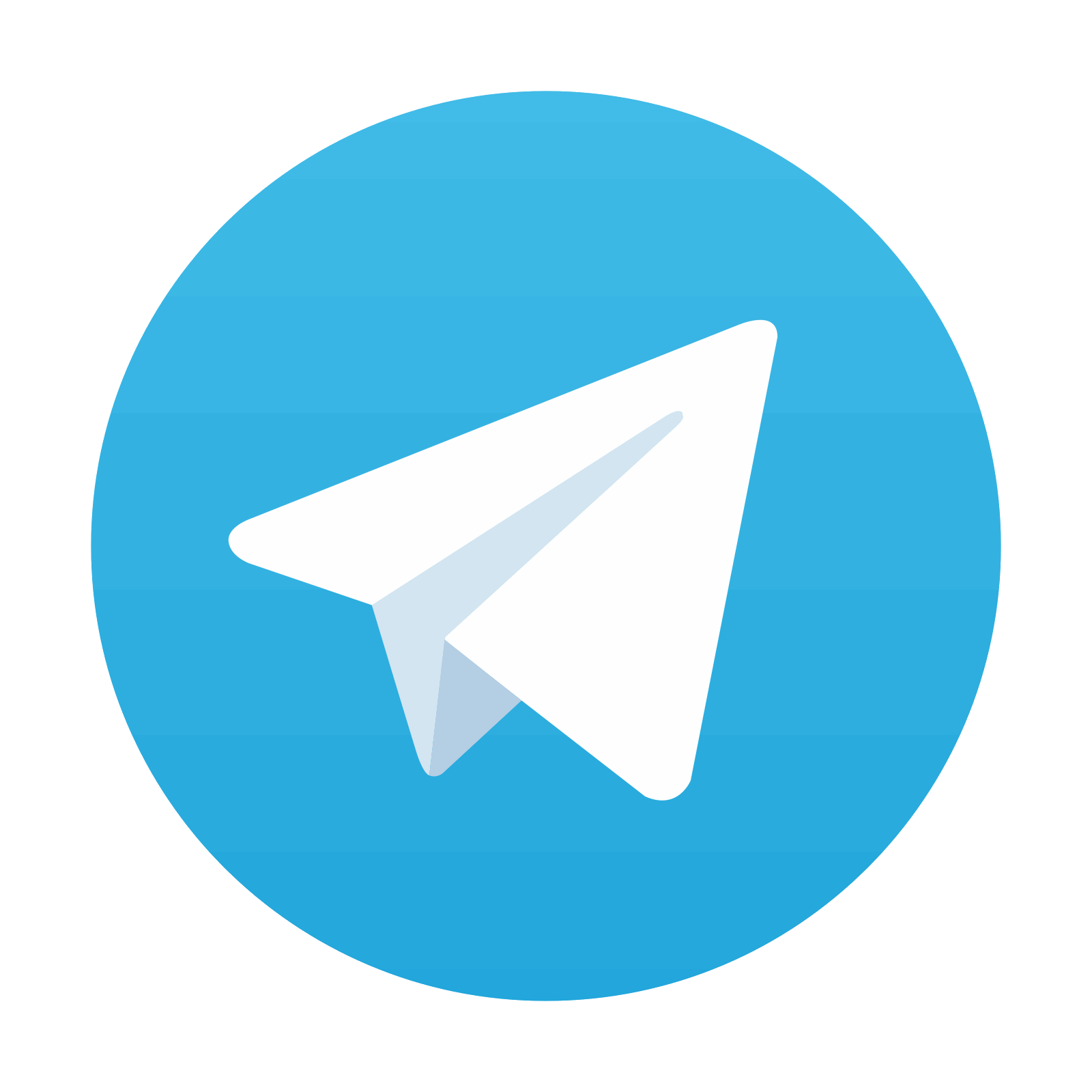
Stay updated, free articles. Join our Telegram channel

Full access? Get Clinical Tree
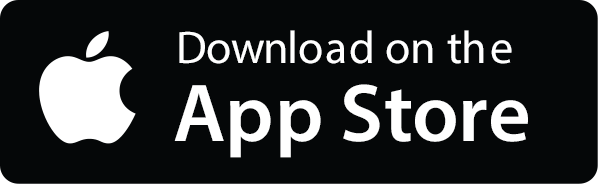
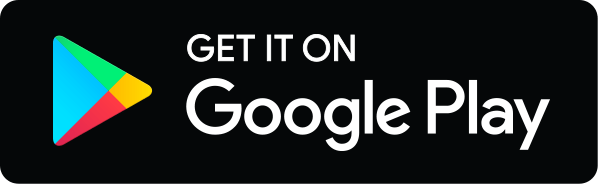