Background
E/e′ is clinically useful for the noninvasive assessment of left ventricular (LV) filling pressure. However, its use in some conditions is controversial, and angle dependence of the Doppler measurement and preload dependence of mitral e′ in nondilated hearts represent major problems. The ratio of early filling rate derived from the time derivative of LV volume to early diastolic strain rate (FRe/SRe), similar to E/e′, by three-dimensional (3D) speckle-tracking echocardiography has the potential to address such limitations. This study investigated whether FRe/SRe could estimate acute changes in LV filling pressure using the models of volume overload and myocardial ischemia in the nondilated heart.
Methods
In 25 dogs, hemodynamic conditions were varied by acute volume overload and coronary occlusion. FRe and SRe were obtained from the same beat and automatically analyzed by the 3D speckle-tracking method, and global SRe was measured from longitudinal (L-SRe), circumferential (C-SRe), and area strain rate (A-SRe). E/e′ was measured by two-dimensional echocardiography. LV pressure was derived from a micromanometer catheter and recorded simultaneously with the acquisition of the 3D images.
Results
Mitral e′ and L-SRe varied by changes in preload, whereas C-SRe and A-SRe did not. C-SRe and A-SRe were more strongly correlated with the time constant of LV relaxation than mitral e′ and L-SRe. FRe/C-SRe and FRe/A-SRe had relatively high correlations with LV preatrial contraction (pre-A) pressure and end-diastolic pressure, but E/e′ and FRe/L-SRe did not. Receiver operating characteristics curve analysis showed that FRe/C-SRe and FRe/A-SRe had larger areas under the curve for the estimation of increased LV filling pressure.
Conclusions
The novel parameter FRe/SRe has potential as a surrogate marker of LV filling pressure. Especially in nondilated hearts, FRe/C-SRe and FRe/A-SRe may be useful to more accurately predict LV filling pressure than E/e′, although their applicability in dilated hearts requires further investigation.
Highlights
- •
The new three-dimensional speckle-tracking parameter, the ratio of the early filling rate to global early diastolic strain rate (FRe/SRe), was proposed for assessing left ventricular (LV) filling pressure in nondilated hearts.
- •
Mitral e’ varied by changes in preload, whereas SRe did not.
- •
SRe was more strongly correlated with tau than mitral e’.
- •
FRe/SRe demonstrated higher correlations with LV pre-A pressure and end-diastolic pressure than E/e’.
- •
FRe/SRe seems to have potential as a surrogate marker of LV filling pressure in nondilated hearts.
Noninvasive evaluation of left ventricular (LV) filling pressure is important for the diagnosis and treatment of heart failure. The ratio of the mitral inflow early diastolic velocity to the mitral annular early diastolic velocity (E/e′) is used as a noninvasive parameter for estimating LV filling pressure. When LV diastolic dysfunction progresses and filling pressure increases, mitral E increases, and mitral e′ is almost always reduced without depending on preload; hence, E/e′ increases. However, several limitations can hamper the clinical utility of E/e′. For example, inappropriate measurement of E/e′ is not rare in patients for whom it is difficult to adjust the Doppler beam alignment. Extrapolation of global diastolic function by regional measurements of mitral e′ seems to be another problem. Moreover, E/e′ may not reflect filling pressure in normal individuals and in patients with heart failure with preserved ejection fraction (HFpEF) because mitral e′ is dependent on preload in such nondilated hearts.
Three-dimensional (3D) speckle-tracking echocardiography is a promising method for the quantitative assessment of whole LV deformation without the through-plane problem. This technique allows the simultaneous evaluation of LV volume and global myocardial strain. The rate of LV volume change (dV/dt), which is equivalent to the flow rate, is calculated by numeric differentiation of LV volume as a function of time. Flow rate denotes the product of blood velocity and cross-sectional area. Assuming that mitral annular area is constant during diastole, the early filling rate (FRe) derived from dV/dt could reflect mitral E. By contrast, global early diastolic strain rate (SRe) has recently been demonstrated to be better correlated with the time constant of LV relaxation (tau) than mitral e′. Hence, the ratio of FRe to SRe (FRe/SRe) may be used as an alternative to E/e′. This parameter can be analyzed from one 3D acquisition and need not take into account the Doppler alignment because the Doppler method is not used.
Global SRe can be measured from three different directions: radial, longitudinal, and circumferential strain rate. Recently, the area tracking based–deformation parameter (area strain rate), which combines circumferential and longitudinal components, can be also measured by 3D speckle-tracking analysis. However, it remains unclear which deformation parameter is most suitable for SRe measurement, one that minimizes preload dependence and reflects LV relaxation. Therefore, the goal of this study was to investigate (1) whether FRe/SRe could estimate acute changes in filling pressure and (2) which SRe was best for this assessment using the models of volume overload and myocardial ischemia in the nondilated heart.
Methods
Animal Preparation
Our experimental study was approved by the animal experimentation committee and performed in accordance with the guidelines for the care and use of laboratory animals at our institution. Thirty open-chest dogs weighing 8.5 kg to 14.6 kg were used in this study. The dogs were anesthetized with intramuscular xylazine (0.5 mg/kg) followed by intravenous pentobarbital sodium (25.9 mg/kg) and then were intubated and ventilated using a respirator. Oxygen saturation was monitored by a pulse oximeter and was maintained within normal ranges. Anesthesia was induced with pentobarbital sodium (6 mg/kg/h) and midazolam (0.18 mg/kg/h) throughout the experiment. Buprenorphine (4 μg/kg) was administered intramuscularly for analgesia. The electrocardiogram was monitored continuously. A 6-Fr micro-manometer catheter (Millar Instruments, Houston, TX) was inserted through the right femoral artery into the LV to measure LV pressure. The chest was opened through a left parasternal thoracotomy, and the heart was exposed. In the model of volume overload, the pericardium was not opened so as to prevent rapid distension of the LV. In the models of myocardial ischemia and changes in inotropic states, the pericardium was opened, and the heart was suspended in a pericardial cradle. In the model of myocardial ischemia, the proximal portion of the left anterior descending artery (LAD) was dissected free of the surrounding tissues, and a vascular occluder was placed around the artery.
Echocardiography
Echocardiography was performed with an Aplio Artida SSH-880CV ultrasound system (Toshiba Medical Systems, Otawara, Japan). The 3D full-volume datasets were acquired from apical positions with a PST-25PX transducer, which was placed on a water bath as a standoff. The frequency was 2 MHz on transmit and 4 MHz on receive. The scan range angle was set from 70° × 70° to 80° × 90°. The acquisition was done during six consecutive heartbeats using electrocardiogram triggering, resulting in a volume rate of 31.2 to 38.8 volumes/s.
Doppler images were recorded with a PST-50BT transducer. The transmitting and receiving frequency was 4.2 MHz. Mitral inflow velocity and mitral annular velocity at the septal and lateral sites were measured. The Doppler recordings were obtained while keeping the ultrasound beam as parallel as possible to the direction of blood flow or cardiac longitudinal motion.
The 3D and Doppler images were acquired while the respirator was stopped to minimize the effect of heart motion caused by breathing.
Experimental Protocol
Protocol 1
The relationships between FRe/SRe and LV filling pressure during acute changes in the model of volume overload were investigated in 14 dogs. Esmolol was infused at 300 μg/kg/min from the beginning of this protocol for two reasons: (1) reactive tachycardia caused by volume overload and (2) the difficulty in increasing LV filling pressure in healthy dogs. The LV filling pressure was escalated by two stepwise infusions of dextran solution (100 mL over 5 minutes each time). Echocardiographic data were obtained at baseline, and after 100 mL and 200 mL of dextran infusion.
The relationships between FRe/SRe and LV filling pressure in the model of myocardial ischemia were investigated in eight dogs. Echocardiographic data were obtained before and 1 minute after LAD occlusion.
Protocol 2
The relationships of tau with mitral e′ and SRe in the model of changes in inotropic states were investigated in eight dogs. Inotropic states were altered by stepwise infusion of esmolol from 100 μg/kg/min to 300 μg/kg/min. Echocardiographic data were obtained before and 5 minutes after esmolol infusion at 300 μg/kg/min.
The LV pressure was recorded simultaneously with the acquisition of the 3D image. The LV systolic pressure, LV pre-A pressure, LV end-diastolic pressure, and tau were averaged over five consecutive cardiac cycles. The LV pre-A pressure reflects mean left atrial pressure as a surrogate parameter of LV filling pressure and was defined as the LV pressure at the onset of the P wave on an electrocardiogram. The LV end-diastolic pressure was defined as the LV pressure at the peak of the R wave. Tau was calculated assuming non-zero asymptote in the data from the point of the minimum time derivatives of the LV pressure to the point at which pressure decreased to the level of the LV end-diastolic pressure.
Data Analysis
The 3D speckle-tracking analysis was performed by offline software (Ultra Extend; Toshiba, Otawara, Japan) ( Figure 1 ). From 3D full-volume datasets, the apical four-chamber and two-chamber views and the three short-axis views were displayed. The endocardial borders of the four-chamber and two-chamber images were manually traced at end-diastole. The epicardial borders were determined by setting an even width of myocardium. The 3D endocardial and epicardial surfaces were automatically reconstructed, and traced borders were finely adjusted on short-axis images. After these tracing processes, the LV volume and regional strain rate curves were measured. The FRe (obtained from the derivative of the LV volume curve) and SRe (obtained from the global strain rate curve averaged over all 16 segments) were used to calculate FRe/SRe. Global SRe was measured from longitudinal strain rate (L-SRe), circumferential strain rate (C-SRe), and area strain rate curves (A-SRe).

From Doppler recordings, mitral E and e′ were measured, and mitral e′ was averaged at the septal and lateral sites. Doppler parameters were obtained from three consecutive cardiac cycles. When mitral E and mitral inflow late diastolic velocity (mitral A) were fused, the datasets were excluded in the final analysis.
Interobserver and Intraobserver Variability
Seven image clips were randomly selected from the model of volume overload. To determine the interobserver variability for FRe and SRe, the analysis was repeated by a second observer who was blinded to the values obtained by the first observer. To determine the intraobserver variability, the analysis was repeated 3 months later by the same observer.
Statistical Analysis
Data are expressed as mean ± standard deviation. The hemodynamic and echocardiographic measurements were compared at baseline and at two stages of dextran infusion by one-way analysis of variance followed by a Tukey-Kramer post hoc test. The data before and after LAD occlusion and esmolol infusion were compared by paired t test. Regression analysis was applied to examine the relationship between echocardiographic and hemodynamic parameters. The slopes of the regression lines in the models of volume overload and myocardial ischemia were compared by analysis of covariance. Increased LV filling pressure was defined as LV pre-A pressure ≥12 mmHg and LV end-diastolic pressure ≥16 mmHg. Receiver operating characteristic (ROC) curve analysis was performed to determine the ability of FRe/SRe to estimate the increased filling pressure, and the areas under the ROC curve (AUCs) were calculated. These AUCs were compared with the line of no information (AUC = 0.50). The interobserver and intraobserver variability for FRe and SRe was determined by intraclass correlation coefficients (ICCs). Values of P < .05 were considered to indicate statistical significance. Statistical analyses were performed using IBM SPSS Statistics for Windows, version 20.0 (IBM Corp., Armonk, NY) and MedCalc for Windows, version 15.2.2 (MedCalc Software, Ostend, Belgium).
Results
In Protocol 1, four dogs with fusion of mitral E and A during volume overload or myocardial ischemia and one dog with insufficient image quality for speckle-tracking analysis were excluded. Because three datasets that had fusion of mitral E and A only at 200-mL infusion of dextran in the remaining dogs were also excluded, 42 datasets from a total of 17 dogs (11 dogs with volume overload and six dogs with myocardial ischemia) were included in the final analysis. In Protocol 2, FRe/SRe could be analyzed in 16 datasets from a total of eight dogs.
Protocol 1
Hemodynamic and Echocardiographic Measurements
The hemodynamic and echocardiographic data are shown in Table 1 . Stepwise infusion of dextran induced a significant gradual increase of LV pre-A pressure and LV end-diastolic pressure. E/e′ did not change during dextran infusion. FRe/SRe in all directions tended to increase during 100-mL infusion of dextran. FRe/C-SRe and FRe/A-SRe significantly increased during 200-mL infusion of dextran compared with baseline, but FRe/L-SRe did not.
Variable | Baseline | 100-mL infusion of dextran | 200-mL infusion of dextran | Baseline | LAD occlusion |
---|---|---|---|---|---|
Hemodynamic data | |||||
Heart rate (beats/min) | 99 ± 13 | 99 ± 15 | 102 ± 8 | 123 ± 12 | 129 ± 17 |
LV systolic pressure (mmHg) | 111 ± 19 | 127 ± 21 | 137 ± 25 | 104 ± 8 | 97 ± 11 ∗ |
LV pre-A pressure (mmHg) | 7 ± 2 | 11 ± 4 ∗ | 16 ± 4 ∗ , † | 3 ± 1 | 6 ± 1 ∗ |
LV end-diastolic pressure (mmHg) | 8 ± 3 | 15 ± 4 ∗ | 21 ± 4 ∗ , † | 6 ± 2 | 8 ± 2 ∗ |
Echocardiographic data | |||||
E/e′ | 9.6 ± 3.0 | 9.9 ± 3.9 | 9.7 ± 3.0 | 12.0 ± 2.8 | 12.3 ± 2.7 |
FRe/L-SRe (mL) | 68.7 ± 11.9 | 73.7 ± 17.1 | 73.3 ± 13.6 | 75.0 ± 13.4 | 101.4 ± 22.2 |
FRe/C-SRe (mL) | 33.1 ± 8.3 | 40.5 ± 6.6 | 47.4 ± 8.2 ∗ | 29.3 ± 4.6 | 39.9 ± 9.8 ∗ |
FRe/A-SRe (mL) | 24.2 ± 4.8 | 28.3 ± 4.5 | 32.2 ± 5.5 ∗ | 22.1 ± 2.5 | 28.9 ± 3.1 ∗ |
The LAD occlusion induced a significant decrease in LV systolic pressure and a significant increase in LV pre-A pressure and LV end-diastolic pressure. E/e′ did not change during LAD occlusion. FRe/C-SRe and FRe/A-SRe significantly increased during LAD occlusion. FRe/L-SRe tended to increase, but the increase was not statistically significant.
Correlations of Echocardiographic Parameters with LV Filling Pressure in the Model of Volume Overload
A range of pre-A pressure from 2 mmHg to 22 mmHg was achieved by dextran infusion. According to Doppler measurements, mitral E and e′ gradually increased along with the stepwise infusion of dextran ( Figure 2 ). Positive correlations with pre-A pressure were found for mitral E and e′, resulting in no correlation between pre-A pressure and E/e′. Positive correlations with LV end-diastolic pressure were also found for mitral E and e′ but not for E/e′ ( Table 2 ).
Variable | Correlation coefficients ( r ) | |
---|---|---|
LV pre-A pressure | LV end-diastolic pressure | |
Doppler | ||
Mitral E (cm/s) | 0.48 ∗ | 0.55 ∗ |
Mitral e′ (cm/s) | 0.65 ∗ | 0.52 ∗ |
E/e′ | −0.27 | −0.10 |
3D speckle-tracking echocardiography | ||
FRe (mL/s) | 0.76 ∗ | 0.68 ∗ |
L-SRe (/s) | 0.56 ∗ | 0.54 ∗ |
C-SRe (/s) | 0.18 | 0.17 |
A-SRe (/s) | 0.35 | 0.33 |
FRe/L-SRe (mL) | 0.40 ∗ | 0.32 |
FRe/C-SRe (mL) | 0.80 ∗ | 0.70 ∗ |
FRe/A-SRe (mL) | 0.78 ∗ | 0.69 ∗ |
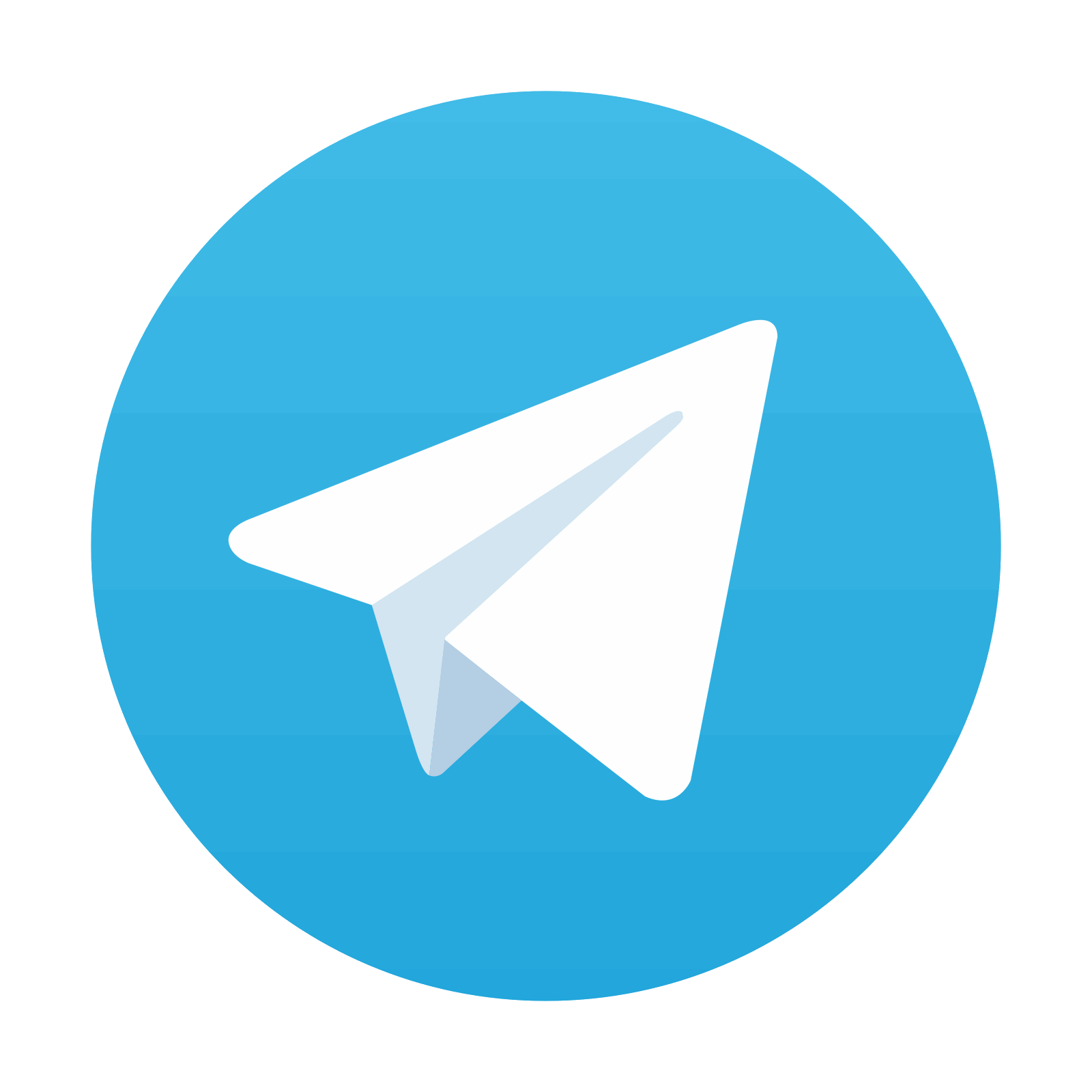
Stay updated, free articles. Join our Telegram channel

Full access? Get Clinical Tree
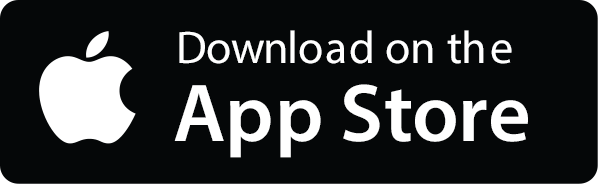
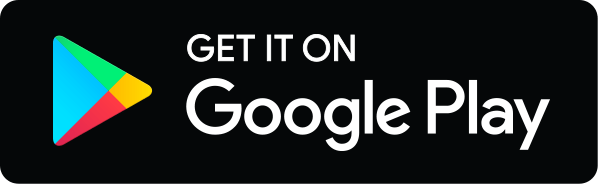
